Central nervous system
Peripheral nervous system
Uremic encephalopathy
Seizures
Cognitive Impairment/dementia/delirium
Cerebrovascular disease
Intracerebral hemorrhage
Cerebral micro-bleeds
Cerebral infarction
Silent cerebral infarction
White matter lesions
Dialysis encephalopathy/dementia
Dialysis-induced abnormalities
Dialysis disequilibrium syndrome
Cognitive dysfunction induced by rapid fluid, electrolyte and blood pressure shifts
Uremic polyneuropathy
Autonomic and cranial neuropathy
Mononeuropathies
Carpal tunnel syndrome
Ischemic monomelic neuropathy
Compressive neuropathies
Combination pathologies
Restless leg syndrome*
Table 36.2
Complications and outcomes of cognitive dysfunction/dementia in patients with renal disease
Increased risk of hospitalizations |
Prolongs hospitalizations |
Leads to lower levels of compliance |
Higher incidence of cerebral vascular disease |
Increased costs of care |
Increased risk of death |
Decreased average life span after beginning dialysis |
Impairs the ability the ability to weigh risks vs benefits of initiating dialysis or withdrawing from dialysis once it is initiated |
Epidemiology
While the presence of CI/D in patients with CKD or ESRD is well recognized, its prevalence has been difficult to accurately establish for several reasons. First, relatively few large studies examine their connection, and the available studies vary greatly in their estimation of disease prevalence [22–25]. Moreover, the cohorts studied may not be representative of the general CKD/ESRD populations, and CI/D may be under-diagnosed in cohorts that rely on diagnostic codes and billing data [17, 26]. Second, CI/D is under-diagnosed in these patients because nephrologists and dialysis staff are not adequately trained to identify mild CI/D. Furthermore, when signs and symptoms are spotted, they are often ascribed to normal aging, other medical problems, or side effects of therapy. Thus, only advanced/obvious dementia is typically diagnosed. Third, cognitive assessments are typically achieved using short screening tests [e.g., MiniMental State Examination (MMSE), Modified Mini-Mental State Exam (3MS)] that have limited sensitivity in ESRD patients [11, 27]. In addition, the timing of testing may also introduce substantial variability in the results [11, 28, 29]. Finally, cognitive impairment and dementia are not listed as comorbid conditions in the CMS 2728 form (ESRD Medical Evidence Report), and cognitive assessments are not required at initiation of dialysis, nor during any of the routine assessments of dialysis patients [30]. Together, these limitations raise the possibility that CI/D is being grossly underdiagnosed, and thus its prevalence is underestimated. This supposition is supported by the fact that the prevalence of dementia is only 7% in the US Renal Data System (USRDS), and 4% in the Dialysis Outcomes and Practice Patterns Study, which represent only one-fifth of probable dementia cases, as determined by more rigorous neuropsychological testing [24, 31, 32]. Similarly, Sehgal et al. [25] found that only 15% of the cognitively impaired patients (MMSE score <24) had a medical record diagnosis of cognitive problems. These studies suggest that there are a vast number of patients with CKD and ESRD who are afflicted with CI/D.
Prevalence of CI/D in CKD
CKD is a term that encompasses a wide spectrum of renal dysfunction ranging from mild (which is usually only diagnosed by blood and urine testing) to ESRD, in which kidney function is impaired to such an extent that the patient requires renal replacement therapy in order to prevent potentially fatal complications from the retention of metabolic waste products, salt, and water. This continuum of CKD has been classified into a five-stage system based on the estimated glomerular filtration rate (eGFR) to help assess the severity of the CKD and its potential for progression [11] (Table 36.3). Overall, CKD has increased in prevalence to the point that it is now recognized as a worldwide public health problem [33]. More than 10% of the adult population currently has some degree of CKD [34], and its prevalence increases considerably with advancing age; 20 to 25% in people aged 65 to 74 to nearly 50% in those aged 75 and over [11, 23, 35].
Table 36.3
Classification of chronic kidney disease
Stage 1: eGFR >90 ml/min with albuminuria, hematuria or abnormal kidney imaging |
Stage 2: eGFR 60–90 ml/min |
Stage 3: eGFR 30–59 ml/min |
Stage 3A: eGFR 45–59 ml/min |
Stage 3B: eGFR 30–44 ml/min |
Stage 4: eGFR 15–29 ml/min |
Stage 5: eGFR <15 ml/min |
ESRD: Patient requires renal replacement therapy or transplantation |
The prevalence of CI/D, like CKD, also increases with age, affecting 10% of the population over 65 [36–38]. Interestingly, CI/D has many similar risk factors to CKD including obesity, diabetes mellitus, hypertension, and dyslipidemia [39–41], suggesting that similar mechanisms may be contributing to its development. Thus, it is not surprising that these two diseases occur concomitantly in many patients, particularly the elderly. However, the prevalence of CI/D in CKD patients far outpaces its prevalence in the non-CKD population [42, 43], suggesting that CKD itself exacerbates the risk of CI/D [10, 44]. Indeed, CKD has been shown to be an independent risk factor for the development and progression of CI/D by both cross-sectional studies and longitudinal studies [22, 24, 39, 40, 44]. Even moderate CKD increases the risk of CI/D by nearly 40% after adjusting for confounders [22, 35, 45, 46]. Moreover, the type of dementia induced by ageing alone compared to ageing plus CKD is different. Alzheimer’s Disease is the most common form of dementia in the elderly [47], whereas vascular dementia accounts for the increased prevalence of CI/D in CKD patients [48]. One explanation for this may be the greater prevalence of both cardiovascular and cerebrovascular risk factors among CKD patients, predisposing them to develop cerebrovascular diseases and consequently CI/D [32, 49, 50].
Patients with CKD of any stage have a greater risk of developing CI/D than the general population [22, 51, 52]. As might be expected, the risk of CI/D increases markedly with worsening kidney function. For every 10 ml/min/1.73m2 drop in eGFR, the risk of developing CI/D increases by 11% [35, 53], and the risk of declining memory, language skills, executive functioning, and global cognition increases by 15–25% [45]. While most studies have found that CKD is associated with increased risks of CI/D, and that the more advanced the CKD the greater the risk, there are a few studies that have not confirmed this connection. Neither the 3C study [48], nor the study by Slinin et al. [54] found a consistent correlation between CKD and dementia. However, the patient cohort in these studies tended to be healthier, had a relatively low incidence of CKD at baseline, and had fewer cardiovascular risk factors, complicating direct comparisons with other studies. Overall, the bulk of the evidence indicates that CKD is associated with an increased risk for the development of CI/D and accelerated decline in cognitive function.
Prevalence of CI/D in ESRD
ESRD, the last stage of CKD, means that the kidneys are functioning below 15% of their normal function and can no longer support a person’s day-to-day life. In the US, more than 300,000 people are diagnosed with ESRD, and its prevalence is increasing worldwide [33, 55]. As previously mentioned, the rate of CI/D increases together with the severity of kidney disease, thus patients with ESRD have the highest prevalence. In ESRD, the prevalence rate of CI/D ranges between 16 and 38%. This is nearly 3 times greater than in the age-matched general population [22, 24, 25, 56, 57]. In fact, some studies that used more robust diagnostic criteria report even higher levels, up to 87% [24]. The variability in the reported prevalence may in part be due to the patient population studied, but more importantly to study design and the large variability in diagnostic testing and criteria used in the distinct studies. For instance, early studies reported moderate rates of CI/D that probably underestimated the true prevalence because they often excluded older and sicker patients from analysis, and used screening tests of limited sensitivity. Later studies tended to be more inclusive and used more thorough neuropsychological testing, thus increasing the diagnostic sensitivity [39]. Not surprisingly, they found the prevalence to be much higher. The cross-sectional analysis by Murray et al. highlights not only the high prevalence of CI/D in hemodialysis patients, but also the marked disparity between the documented history of CI/D (only 2.9%) and its presence upon more thorough neuropsychological testing; 12.7% had normal cognitive function, whereas 13.9%, 36.1%, and 37.3% had mild, moderate, and severe cognitive impairment respectively [24]. Thus overall, it is clear that the prevalence of CI/D is much higher in the CKD/ESRD population than in the general population, even after adjusting for age and other common risk factors. This implies that renal disease per se is a strong risk factor for the development of CI/D. It remains to be determined how much of this increase in risk is due to the same mechanisms that catalyze the accelerated vascular disease present during renal disease, and how much is due to other mechanisms, including those brought on by the treatment strategies used in CKD/ESRD.
Pathogenesis and Pathophysiology
Patients with CKD/ESRD develop CI/D in part via similar mechanisms as the general population, with the caveat that while Alzheimer’s Disease is the most common form of dementia in the general population, the increased incidence of CI/D in CKD/ESRD patients is mainly due to an increase in vascular dementia; there is little if any change in the prevalence of Alzheimer’s Disease [46, 58]. Moreover, there are many additional factors that contribute to and/or exacerbate the vascular and neurologic injury in patients with renal dysfunction (Table 36.4). In general, it is practical to divide the mechanisms/factors that cause CI/D in CKD/ESRD into the following. First, there is a marked increase in the occurrence of cerebrovascular disease due to the accelerated vascular disease that is a characteristic of CKD/ESRD. Moreover, the brain is highly susceptible to microvascular disease, including subclinical cerebrovascular lesions, and its other manifestations (e.g., white matter lesions, micro bleeds). Finally, there are numerous additional mechanisms that render CKD/ESRD patients very susceptible to brain injury and CI/D. These may be related to the renal dysfunction itself (non-traditional vascular and neuropsychological risk factors), or to its therapy. Table 36.4 provides a partial list of the various factors that may contribute to the development and progression of CI/D in CKD/ESRD patients. All of these factors interact to a varying degree in individual patients, causing vascular injury, endothelial dysfunction, and/or direct neurotoxicity, thereby resulting in CI/D of variable manifestations and severity (Fig. 36.1).

Table 36.4
Pathogenic factors that may contribute to CKD/ESRD-associated dementia
Cardiovascular risk factors | Cerebrovascular neuro-psychological | Treatment-related | |
---|---|---|---|
Traditional | Non-traditional/uremic | ||
Older age Hypertension Diabetes Mellitus Dyslipidemia Albuminuria Sex Race Educational status Cardiovascular disease Smoking Atrial fibrillation | Uremic factors Volume overload Hyperhomocysteinemia Hyperparathyroidism Elevated FGF-23 Low vitamin D levels Anemia Hypercoagulation Inflammation Oxidative stress Malnutrition Frailty | Cerebrovascular Stroke Silent stroke Lacunar infarcts Microembolism Microbleeds White matter lesions Cortical atrophy Psychological Depression | Polypharmacy Aluminum Dialysis-related Modality? Hemodialysis initiation Hemodynamic instability Fluid and solute shifts |

Fig. 36.1
Pathogenesis of cognitive impairment in chronic kidney disease
Cerebrovascular Disease
Thromboembolic Disease
Patients with CKD/ESRD frequently have accelerated vascular disease, which is why most of those with advanced disease also have advanced vasculopathy, and have high rates of hypertension (80%), diabetes (60%), vascular endothelial dysfunction, carotid atherosclerosis, and cardiovascular events including stroke [19]. Indeed, the prevalence of stroke in the USRDS CKD and ESRD populations are 2.5 and 4 times greater respectively than in the general Medicare population [19]. Moreover, silent strokes occur in nearly 1/3rd of the older population or 5 times more often than symptomatic strokes [59, 60]. They are associated with increased risk of subsequent clinically evident stroke, cognitive and physical decline, and incident dementia [59–62]. Those who develop symptoms have more severe symptoms and worse outcomes; they have a higher morbidity and mortality [63], increased tendency to develop new strokes [64], and suffer 6 to 9 times more strokes when hospitalized than non-CKD/ESRD patients [65]. Atherosclerosis in patients with CKD/ESRD is generally more diffuse and distally located than in the general population, probably because of a combination of traditional atherogenic risk factors (e.g., male gender, age, diabetes mellitus, hypertension, dyslipidemia, and smoking) and factors more specifically related to renal failure and its treatment that impact both cerebrovascular and microvascular disease (discussed below).
The increase in thromboembolic strokes in CKD/ESRD is also related to the high frequency of dilated cardiomyopathy and arrhythmias [66, 67]. In this respect, atrial fibrillation deserves special attention, as it is the most common cardiac dysrhythmia and is associated with increased cardiovascular morbidity and mortality [68, 69]. Patients with advanced CKD and ESRD are especially prone to it because of (a) structural heart disease including left ventricular hypertrophy, coronary artery disease, and degenerative valvular disease (as a result of accelerated calcifications) [69, 70], (b) increased activity of the sympathetic nervous system and the renin angiotensin system, and (c) rapid shifts of fluid and electrolytes [68, 69, 71, 72]. Its prevalence is 10–20 times higher than in the general population; it is 7% and 13% in peritoneal dialysis patients and hemodialysis patients respectively, according to USRDS data [73]. Smaller studies using more sensitive methods suggest much higher rates [74, 75]. The Monitoring in Dialysis Study recorded patients’ heart rhythms continuously for up to 30 days, and found that 44% of patients experienced at least one episode of sustained atrial fibrillation (>6 min duration), while 85% developed some period of atrial fibrillation during the 30-day follow-up [76]. Thus, while the risks of atrial fibrillation are well recognized, its impact on CI/D in CKD/ESRD is poorly described and probably grossly under-recognized.
Microvascular Disease
The microvasculature of the brain is highly susceptible to microvascular injury; thus the same mechanisms implicated in the progression of CKD may also affect brain function and morphology, and hence cognition. This parallel injury model is appealing because of the similarities in the microvasculature of the brain and the kidneys, in that both organs have low resistance and are exposed to high-volume blood flow, which make them highly susceptible to microvascular disease (e.g., hypertension, diabetes), which in turn may contribute to dementia. Indeed, there appears to be a positive correlation between hemodynamic impairment and cognitive impairment, suggesting that microvascular damage contributes to CI/D [32, 77].
Microvascular disease may manifest itself as CKD in the kidney, and as white matter lesions, silent brain infarcts, and micro-bleeds in the brain [60, 78]. White matter lesions are caused by the accumulation of degenerating cells. Their prevalence is greatly increased in patients with CKD compared to controls (33% vs 6%) [78, 79]. Indeed, they are independently associated with CKD and albuminuria even after adjusting for hypertension and diabetes mellitus [80], and are associated with deficits in the cognitive domains of executive function and processing speed. Importantly, they are predictors of stroke, dementia, and death [81].
Intracerebral Hemorrhage, Subarachnoid Hemorrhage, and Subdural Hematomas
Advanced CKD/ESRD patients also have a large increase in hemorrhagic strokes including intracerebral, subarachnoid, or subdural hemorrhage, which carry a high morbidity and mortality that reaches up to 60% incidence [82–84]. This increased susceptibility for intracerebral and subarachnoid hemorrhage is multifactorial. First, there are patient-related factors such as hypertension or the presence of cerebral vascular malformations (e.g., saccular aneurysms and dolichoectasia) in certain families with polycystic kidney disease [85, 86]. Second, uremia increases bleeding tendency by altering platelet function and platelet–vessel wall interaction [87–89]. The final and most important risk factors are the use of anticoagulation or platelet antiaggregants [88–92]. These agents, together with hypertension, head trauma, rapid ultrafiltration, and the use of hypertonic dialysate, also increase the risk of acute and chronic subdural hematoma [9]. It is important to note the role of hemodialysis in this risk. On the one hand, uremic bleeding may be largely corrected by dialysis. However, systemic anticoagulation is frequently necessary during hemodialysis to prevent circuit clotting, and thus the hemodialysis procedure is associated with a higher incidence of intracerebral and subarachnoid hemorrhage [93–95], as well as subdural hematomas, all of which impair cognition.
Non-traditional Vascular Factors and Other Factors
CKD/ESRD brings about several changes in the non-traditional vascular factors.This includes retention of some (such as uremic toxins), or abnormal production of others (such as increased oxidant stress and inflammation and decreased erythropoietin), leading to a dysregulation of the internal milieu (e.g., acid base and electrolytes). It is thus easy to imagine how the subsequent metabolic quagmire post-CKD/ESRD can facilitate increased prevalence of CI/D. For instance, retention of uremic factors leads to derangements in glutamine, glycine, aromatic and branched-chain amino acids, and subsequently an imbalance of gamma-aminobutyric acid, serotonin, and dopamine neurotransmitters [40]. The metabolism of these neurotransmitters is additionally impaired by the presence of secondary hyperparathyroidism via abnormally increased cellular uptake of calcium. Moreover, increases in oxidative stress and inflammation, together with hypercoagulability, can cause endothelial dysfunction with subsequent vascular injury including leukoaraiosis, silent strokes, and micro-bleeds or even severe, neurodegenerative stroke [39, 96]. Finally, the episodic electrolyte abnormalities can contribute to CI/D by virtue of their direct effects on cellular function, or via their correction. For instance, an ESRD patient may develop episodic hyponatremia because of bouts of excessive consumption of hypotonic fluids that are then inadvertently corrected too rapidly by hemodialysis, which may result in progressive injury.
Uremic toxins
Declining renal function leads to accumulation of a diverse group of uremic retention products including (a) small water-soluble, non-protein-bound compounds (e.g., urea and related carbamylation products, guanidines, uric acid), (b) small, lipid-soluble and/or protein-bound compounds (e.g., homocysteine, indols, phenols), and (c) the middle molecules (parathyroid hormone, advanced glycation end products, β2 microglobulin, FGF-23). These factors individually and/or in combination can effect a variety of cell types that in turn can cause organ dysfunction including cognitive dysfunction [97]. For instance, accrual of guanidino compounds activate N-methyl-d-aspartate receptors while concomitantly inhibiting gamma-aminobutyric acid receptors, causing neurotoxicity [98]. Guanidino compounds can also elevate free levels of homocysteine, which promotes endothelial/vascular injury and thus ultimately contributes to the development of CI/D [99]. Homocysteine levels are two- to four-fold elevated in CKD compared to patients without CKD. This exacerbates atherosclerosis by increasing the proliferation of vascular smooth muscle cells, and disrupts vessel wall-related anticoagulant functions, resulting in enhanced thrombogenicity [100, 101]. These vascular effects, when present in cerebral vessels may contribute to cognitive dysfunction. Indeed, hyperhomocysteinemia is a strong independent factor for the development and progression of CI/D [99]. Other protein-bound uremic factors such as p-cresyl sulfate also contribute to the vascular injury in part by inducing oxidative stress and inflammation, which results in tubular injury, and endothelial injury (increased vascular permeability). In addition, it decreases Klotho, a transmembrane protein that when deficient (as occurs in CKD) is involved in premature aging syndromes and cell senescence [102, 103]. Experimental studies have shown that Klotho-deficient mice have impaired cognition [104], raising the possibility that it may be implicated in CKD/ESRD-induced CI/D. We have enumerated only a few important uremic toxins; indeed, a large variety of other uremic toxins have also been implicated in CKD/ESRD-induced CI/D, but it is outside the scope of this chapter to list them all.
Oxidative stress and inflammation
There are numerous mechanisms by which oxidative stress is increased in CKD/ESRD [105–107]. It fosters endothelial dysfunction, vascular injury, and direct neurotoxicity, which together may have a causal role for the development of CI/D [44, 108, 109]. One suggested mechanism is via uremia-induced oxidative stress, which upregulates the NMDA receptors, thus inducing neuronal nitric oxide synthase. The ensuing increase in nitric oxide combines with the superoxide, resulting in the formation of peroxynitrite that causes protein nitration leading to structural and functional abnormalities in the brain, and ultimately cognitive dysfunction [110, 111]. In a recent study, experimental CKD induced by subtotal nephrectomy increased oxidative stress levels, and led to cognitive dysfunction. Histological evaluation revealed that the CKD group had increased oxidation in the hippocampal neurons (an important center for learning and memory function) and exacerbated levels of neuronal apoptosis. However, administration of antioxidants attenuated the cognitive dysfunction, suggesting a link between oxidative stress and cognitive decline in these animals [112]. Similarly, inflammatory mediators have been associated with dementia in the general population [113]. Moreover, dialysis patients have elevated levels of pro-inflammatory mediators such as prostaglandin D2-synthase, and C-reactive protein, which has been found to induce neuronal apoptosis [114, 115]. Overall, oxidative stress and inflammation work in tandem, inducing endothelial dysfunction and vascular injury causing accelerated atherosclerosis and thus increasing the risk of CI/D in these patients.
Treatment-Related Factors
Aluminum toxicity
Aluminum toxicity was a major cause of dialysis dementia in the early days of dialysis [116]. The exact mechanism through which aluminum exerts its neurotoxicity is still unclear. It has been suggested that aluminum increases oxidative stress and inflammatory cytokines. This incites apoptotic cell death, thereby causing neurotoxicity. In the early dialysis set-ups, aluminum contamination in the dialysate fluid, and also the use of phosphate binders with aluminum, had contributed to this toxicity [116]. However, since then, with the advent of more modern water filtering techniques and decreased use of aluminum containing phosphate binders, the prevalence of aluminum related toxicity in dialysis patients has been gradually reducing. Indeed, the Dialysis Outcomes and Practice Study could only detect a prevalence of 0.6–1% of all dialysis patients [31]. Despite concerns about the cost-effectiveness of routine aluminum testing in the United States, the K/DOQI guidelines recommend testing serum aluminum levels at least once a year in all hemodialysis patients, and once every 3 months in patients receiving aluminum containing medications [117].
Dialysis-induced causes
While modern renal replacement therapies have numerous benefits and much improved safety profile (e.g., much more accurate ultrafiltration rates and much purer dialysis solutions), the dialysis process itself is still associated with many complications that negatively impact the dialysis patient. For instance, excessive ultrafiltration or the rapid compartmental shifts in fluids, electrolytes, and metabolic products can cause hemodynamic instability, with subsequent alterations in cerebral hemodynamics and metabolism [32, 118]. Indeed, studies have found evidence of substantially lowered cerebral blood flow velocity, perfusion, and decreased oxygen metabolism after dialysis [118–120]. These changes precipitate the production of cytokines and consequent inflammation, which exacerbates cerebral vascular injury. These acute factors can result in delirium and acute cognitive impairment, which can progress or contribute to chronic dementia. Moreover, hemodynamic instability is often accelerated by the presence of other systemic inflammatory entities such as sepsis and cardiac dysfunction, which may exacerbate the local hypoxemia and thus CI/D. Indeed, dialysis has been linked to cerebrovascular accidents, with the ensuing development and progression of acute and chronic cognitive dysfunction, particularly in the elderly patient [32, 121]. The immediate post-dialytic period appears to be the time when the patients are most susceptible to cerebral ischemia. Indeed, Toyoda et al. found that nearly one third of new onset acute strokes occurred in the first half hour post-dialysis [15]. Interestingly, they reported a greater incidence of infarcts in the vertebrobasilar territory, a region that is more susceptible to hypovolemia, suggesting that hemodynamic changes during the dialytic process may play a central role in instigating the ischemic events.
Diagnosis
Mild to moderate CI/D exhibits subtle signs and symptoms which can easily be missed by an untrained professional. Indeed, most nephrologists and dialysis staff are not trained to recognize cognitive changes in CKD/ESRD patients. However, the presence of CI/D directly impacts their prognosis — this significantly impacts the therapy, overall duration of hospitalization, and health care costs — thus it is crucial for health care professionals to recognize these signs and symptoms [11, 32]. Specifically, CI/D decreases quality of life and increases mortality of all patients, and even more so CKD/ESRD patients. It also interferes with their ability to comprehend and follow the dietary, fluid, and medication regimens, which is of crucial importance because of their impact on outcomes (the average ESRD patient takes 19 pills/day) [122]. Finally, CI/D greatly interferes with their ability to make complex decisions including those related to initiation of dialysis and vascular access placement [11, 32, 123]. Thus, periodic screening is needed to identify patients with CI/D so that proper steps can be taken to improve their clinical care.
Initial Evaluation of CI/D in CKD/ESRD: Ruling Out Depression and Delirium
The first step in evaluating these patients is to elicit a good history when possible. A proper history should optimally include the family/caregivers in addition to the patient, due to the inherent nature of the cognitive defect and also since the caregivers will be the first to notice any overt changes in the patient’s cognitive symptoms. The history should include a complete physical examination looking for signs of neurodegenerative diseases such as Parkinson’s, tardive dyskinesia, and others, as they can mimic early signs of CI/D [11]. Despite the importance of the history, it is important to recognize that it lacks sensitivity and thus one must have a low threshold to perform additional screening or testing.
The initial evaluation must also assess for the presence of other conditions that can have similar signs and symptoms to those of CI/D, in particular depression and delirium. Indeed, depression is the most common neuropsychiatric disease observed in ESRD patients [13, 124]. The early signs and symptoms of depression are usually indistinguishable from those of early CI/D. It must be ruled out as a reason by performing depression screening tests such as the Beck depression inventory, the five-item Geriatric Depression Scale, or a patient health questionnaire [125, 126]. Similarly, delirium is especially common in older patients who are on multiple medications (such as elderly CKD patients), and must be ruled out by performing the confusion assessment method (a highly sensitive and specific test to detect delirium) [127, 128]. Delirium can be precipitated by a number of causes such as electrolyte disorders (hyponatremia, hypercalcemia, or hypoglycemia), medications (opioids, anti-psychotics, anti-cholinergic and anti-histaminergic drugs) infections, alcohol and drug intoxication, or withdrawal among others [129] (Table 36.5). Once delirium is suspected, the nephrologist must assess the likelihood that the metabolic and chemical abnormalities (a measure of dialysis adequacy) may be contributing to the CI/D. Note that because uremia causes encephalopathy, it mistakenly gets blamed for delirium quite frequently. The physician who is following the patient in a longitudinal manner will be able to determine this with more accuracy.
Table 36.5
Differential diagnosis of neurological dysfunction in dialysis
Type | Disorder | Presenting signs and symptoms |
---|---|---|
Common causes | Delirium | Impaired memory and attention, disorganized thoughts |
Depression | Depressed mood, anhedonia, insomnia/hypersomnia, psychomotor agitation or retardation | |
Alzheimer’s | Progressive memory loss, impaired executive function and abnormal visuospatial skills | |
Metabolic abnormalities | Hypoglycemia | Confusion, lack of concentration, headache, personality changes |
Hypophosphatemia | Ranges from mild paresthesia to severe mental status alterations. | |
Hyponatremia | Ranges from mild headache and cramps to severe altered mental status changes and status epilepticus | |
Hyperosmolarity | Ranges from mild disorientation to coma | |
Hypercalcemia | Anxiety, altered sensorium, depression, insomnia | |
Uremia | Depression, delusions, irritability, loss of memory and concentration, psychosis, coma. | |
Trace element intoxication | Altered sensorium and confusion | |
Aluminum | Altered mental status, seizures, myoclonus, impaired speech | |
Vitamin deficiency: Thiamine Vitamin-C Vitamin-B12 | Wernicke–Korsakoff, impaired memory, lack of coordination and paralysis | |
Depression, increased arousal, decreased | ||
motivation Depression, mania, psychosis | ||
Traumatic brain lesions | Subdural hematoma | Headache, confusion, vomiting, slurred speech, coma |
Structural brain lesions | Normal pressure hydrocephalus | Non-specific neurological symptoms |
Hypertension induced | Hypertensive encephalopathy | headache, altered consciousness, seizures and impaired vision |
Stroke | confusion, dementia, memory loss, impaired communication | |
Dialysis induced | Hemodynamic instability | Confusion |
Dialysis delirium syndrome | focal neurological defects, altered consciousness | |
Alcohol or drug withdrawal | Wide range from mild disorientation to seizures and coma |
Screening Tests
After eliminating the reversible causes of cognitive impairment, the patient with suspected CI/D undergoes general screening to detect the presence and type of cognitive dysfunction. Since these patients are followed frequently in CKD/dialysis units, ideally they should undergo these screening tests during their clinic visit or just prior to the dialysis session. However, this may be difficult to accomplish in most renal clinics and dialysis units due to logistical reasons, so early referral to specialized services for evaluation using neuropsychological and neurophysiological tests is warranted.
When screening in CKD clinics and hemodialysis is possible, it normally starts with generalized neuropsychological tests. The ones most commonly used in renal clinics/hemodialysis units include the MMSE, the 3MS, Cognitive Capacity Screening Exam (CCSE) and the Kidney Disease Quality of Life Cognitive Function (KDQOL-CF) scale [11, 130, 131]. Their main drawbacks are that they have suboptimal sensitivity and specificity, and limited efficacy in detecting abnormalities in executive function, a very common finding in vascular dementia and ESRD [39, 131, 132]. Moreover, none of these screening tests (other than the KDQOL-CF) have been validated by clinical trials in CKD/ESRD patients with CI/D [11, 39, 131, 132]. It is also important to point out that CKD/ESRD clinics have a large variability of ethnic groups and tend to have a high proportion of patients with limited education, which further limits the effectiveness of the tests, as some rely on the patient’s verbal or mathematical skills [133–135]. The results may also vary depending on when they are administered. For example, they are commonly run on ESRD patients during dialysis (for logistical reasons), yet this is the worst time to run them since cognitive function is at its lowest. One study which looked at the cognitive function 1, 24 and 67 h post-dialysis session in patients on hemodialysis versus peritoneal dialysis found that the hemodialysis patients fared worse after 67 h compared to 1 and 24 h, whereas the peritoneal dialysis patients fared similarly throughout the testing period [136]. Despite these drawbacks, they are valuable in identifying patients that may benefit from referral to a specialist. In our view, any CKD/ESRD patient with abnormal screening or in which the nephrologist has a high suspicion that CI/D may be present, should be referred to a specialist (Fig. 36.2).


Fig. 36.2
Diagnostic algorithm for cognitive dysfunction in a CKD/ESRD patient
Referral to a specialist will trigger a more comprehensive assessment, including in-depth testing to detect specific abnormalities in orientation, memory, intelligence, attention span, depression, and verbal skills (Fig. 36.2). These may include the Wechsler Memory Scale — Third Edition (WAIS-III) to assess personal, temporal, and spatial orientation, estimated verbal IQ test (NAART) to assess intelligence, Simple reaction time (SRT) to assess attention and vigilance, Boston naming test for verbal skills, and Wechsler Memory Scale — Third Edition (WMS-III) for memory [77, 121]. These tests are more sensitive and can thus detect mild to severe cognitive impairment [77, 137]. The specialist can additionally determine whether the patient merits neurophysiological techniques such as EEG and event-related potentials, or specialized imaging.
Imaging Studies
In general, imaging studies are most useful in determining potential etiologic factors that may need to be addressed. For instance, they can confirm the presence of carotid artery stenosis, or suggest the presence of paroxysmal atrial fibrillation because of embolic strokes. Imaging can also not only uncover the presence of white matter lesions, micro-bleeds, lacunar infarcts, and cerebral atrophy, but can also sometimes surprise us with findings consistent with cerebral edema from dialysis disequilibrium syndrome, osmotic demyelination syndrome, posterior reversible encephalopathy syndrome, infections, and sinus vein thrombosis among others [138–140].
As in non-CKD/ESRD patients, neuroimaging is not used to diagnose cognitive impairment or dementia (these are of course clinical diagnoses); rather, it assists the physician to rule out the structural and functional causes which can contribute to CI/D. Routinely used imaging techniques include computed tomography (CT) and magnetic resonance imaging (MRI) to detect structural abnormalities, and positron emission tomography (PET) to exclude functional abnormalities. The American Academy of Neurology recommends using CT or MRI imaging to identify structural brain lesions leading to cognitive impairment [141]. CT is usually obtained first in patients presenting with cognitive symptoms because it is widely and quickly available. CT can detect lacunar strokes, periventricular white matter lesions, and infarcts, which when present in the dominant cerebral hemispheres and limbic system suggest the presence of vascular dementia [142]. Moreover, it can also help detect silent brain infarcts, intracerebral hemorrhage, micro-bleeds, and cerebral atrophy, a very common finding in CKD/ESRD patients [32, 79, 140]. While using intravenous contrast can increase the sensitivity of CT for certain etiologies, it must be used with care in patients with renal dysfunction because it may precipitate or exacerbate acute kidney injury. It should be noted that the presence of renal failure is not a complete contraindication to the use of contrast; the risk of contrast nephropathy is actually quite low if appropriate prophylactic measures are undertaken, particularly ensuring adequate hydration [143, 144].
MRI is more sensitive than CT scans due to the better tissue contrast, flexibility of the image, and also absence of ionizing radiation [145]. MRI studies in CKD/ESRD patients have revealed increased incidence of large and small vessel strokes, along with white matter lesions (risk factors for cognitive dysfunction). Some of the conventional MRI techniques used to detect structural lesions in CKD patients include T2-diffusion weighted imaging and diffusion tensor imaging [146, 147]. However, these conventional MRI techniques are limited in detecting the subtle vascular changes seen in mild to moderate cognitive impairment in some of these CKD/ESRD patients. Advances in MRI techniques, including voxel-based morphometry, have been developed which can be used to better detect these changes. Using gadolinium as a contrast agent improves the sensitivity, but it should not be used in patients with Stage 4 or 5 CKD (an eGFR of <30 ml/min) because it can instigate nephrogenic systemic fibrosis, a catastrophic complication causing systemic fibrosis [148, 149]. If gadolinium has inadvertently been administered to a patient with advanced CKD or ESRD, dialysis should be considered, as it may reduce the contrast load [150]. Various advances to the MRI technique have been developed in order to improve the imaging of the white matter (diffusion tensor imaging) and grey matter (voxel-based morphometry). These methods have been used to demonstrate that CKD/ESRD with CI/D have white matter fiber abnormalities and/or minute microstructural brain atrophy and lower grey matter volume, including in the insular gyrus when compared to healthy controls [14, 151, 152]. These changes may explain the propensity of these patients to develop CI/D [152, 153]. Other advanced techniques include arterial spin-labeled perfusion (ASL) and blood-oxygen-level-determining (BOLD) MRIs. ASL-MRI has been utilized to study the cerebral perfusion changes in hemodialysis patients and the BOLD-functional MRI (fMRI) has the potential to be used to examine the neural mechanisms linked to the pathogenesis of CI/D in ESRD patients [14, 154]. Overall, CT and MRI are very useful in detecting cerebrovascular lesions. In the absence of such lesions, the chances of a vascular etiology for the dementia are low [155, 156].
While CT and MRI reveal structural abnormalities, functional and metabolic abnormalities can be assessed using the PET imaging technique. PET imaging can detect frontal lobe hypoperfusion and hypometabolism in vascular dementia patients, thereby assisting in differentiating it from Alzheimer’s Disease, which shows a more parieto-temporal pattern of lowered cerebral perfusion [157–159]. Using PET imaging, Kanai et al. found that hemodialysis patients metabolized less oxygen in the brain and have lower regional blood flow compared to normal individuals [160]. These parameters were greatly diminished post-dialysis. These hemodynamic changes have been postulated to play a role in the cognitive dysfunction observed in these patients.
Therapeutic Strategies
Evidence-based recommendations for managing CI/D in the CKD/ESRD patient are lacking because there are almost no trials targeting these specific populations, and because most clinical trials exclude CKD/ESRD patients due to their complexities brought about by the presence of numerous confounding comorbidities and alterations in drug metabolism. Thus, most approaches are extrapolated from patients with mild to no renal disease, and based on our knowledge of the disease processes causing the CI/D. The initial step is to treat any associated condition such as depression and delirium; in particular, one must ensure appropriate fluid, electrolyte, acid base, and metabolic balance, as well as eliminate non-essential medications. After ruling out or addressing these factors, the therapy should be aimed at the specific causes and risk factors of CI/D. We will not focus on general approaches (N–methyl D-aspartate receptor antagonists and cholinesterase inhibitors) to CI/D caused by Alzheimer’s Disease and vascular dementia, since it is largely unchanged (other than dosing the drugs appropriately to adjust for renal function). Rather, we will focus on the components and risk factors that are more directly related to CKD/ESRD that may impact CI/D.
Managing Traditional Cerebrovascular Risk Factors for CI/D in CKD
Vascular dementia in CKD is often interlinked with cerebrovascular events. Hence, medications decreasing stroke risk, such as anti-hypertensives, statins, antiplatelet agents, and anticoagulants may be beneficial in CKD-induced CI/D. In addition, smoking and diabetes should be managed as in patients without CKD. We will discuss three main risks in which there are important considerations in this patient population: hypertension, dyslipidemia, and atrial fibrillation.
Hypertension is highly prevalent in patients with CKD/ESRD, and can be refractory to therapy. While most nephrologists agree that lowering blood pressure is important to slow the progression of CKD and to reduce the risk of cardiovascular complications in CKD /ESRD patients, its effect on CI/D is less clear. Observational studies and clinical trials suggest that antihypertensive therapy reduces CI/D, but longitudinal studies have provided inconsistent results [39, 161]. Moreover, there is controversy as to: (a) what is the optimal blood pressure (cohort studies suggest that aggressive control increases the risk of worsening cognition), (b) what are the best agents for achieving this target, and (c) what is the true risk/benefit ratio for CI/D at different blood pressures [162]. Hence, there are no absolute blood pressure targets, and thus management should be individualized according to the patient’s age, severity of albuminuria, and comorbidities [162, 163]. The Kidney Disease: Improving Global Outcomes (KDIGO) clinical practice guidelines for the management of blood pressure in CKD suggests a blood pressure threshold of <140/90 mm Hg for CKD patients without proteinuria, and a lower threshold of <130/80 mm Hg in those patients with albuminuria of >30 mg/24 h [164]. The recent SPRINT trial [165] suggests that our target should actually be lower, <120/80 mm Hg in patients with high cardiovascular risk (e.g., CKD patients). However, there are no trials where the main outcome was CI/D (we anxiously await the results of the SPRINT–MIND study). Thus, particular attention must be paid to complaints that may be related to episodic hypotension and changes in neuropsychological symptoms. This is especially true in ESRD patients, who often require more precise adjustments of their antihypertensive regimen because of the additional risk of intradialytic hypotension [166].
Whether there is a preferred class of medication for CI/D in patients with CKD also remains to be determined. Due to the unique microvascular susceptibility seen in both the kidneys and the brain, some studies have suggested that the microvasculature of both organs may benefit from renin–angiotensin system (RAS) blockade, particularly in the presence of albuminuria [138]. Indeed, several studies have shown that RAS blockers prevent renal damage in the kidneys and also the occurrence or recurrence of stroke in the brain [167, 168]. Interestingly, the protective effects of ACE inhibitors on cognitive decline and dementia do not correlate well with their antihypertensive effects [169]. However it should be mentioned that despite their reported beneficial effects, ACE inhibitors might actually worsen cognitive defects, since they inhibit the conversion of Aβ42 (amyloid beta peptide) to the less toxic and amyloidogenic Aβ40 [39]. Angiotensin receptor blockers (ARB) do not have this potential negative effect, and thus theoretically may be a better alternative. Calcium channel blockers (CCB) have also been shown to be protective against dementia in a large European trial [170]. Moreover, the combination of a CCB with an ARB was more effective than that of a diuretic and an ARB [138, 171]. Despite these promising results, more work is needed before we can offer strong recommendations.
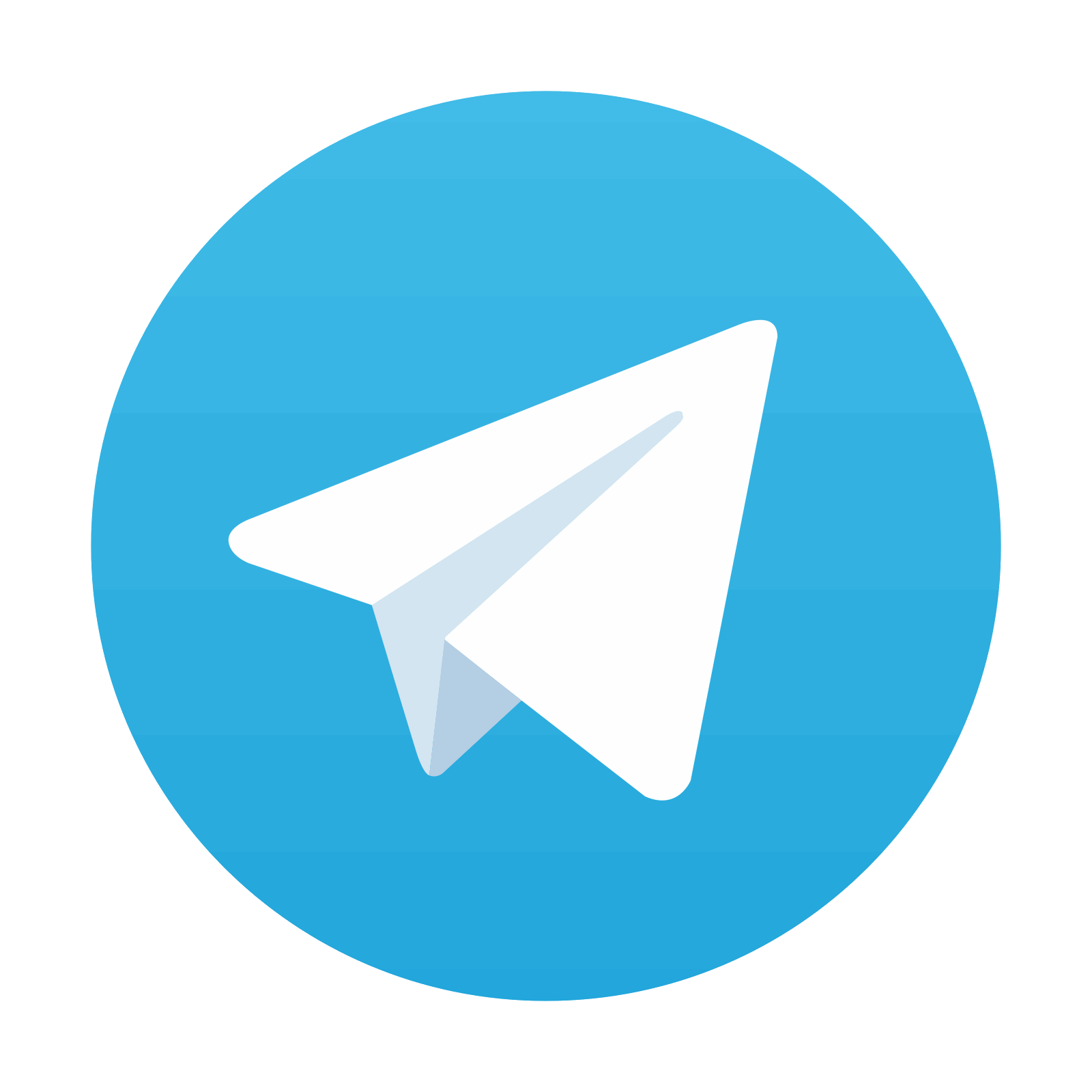
Stay updated, free articles. Join our Telegram channel

Full access? Get Clinical Tree
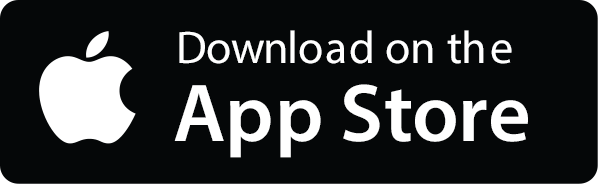
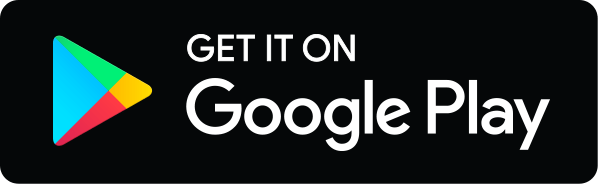