Fig.3.1
Percentage of patients achieving any recanalization (as detected by transcranial doppler ultrasound or CT angiography) within 2 h of tPA bolus, complete recanalization 2 h after tPA bolus, reocclusion within 2 h of tPA bolus, symptomatic intracerebral hemorrhage, or parenchymal hemorrhage type 2 in 65 patients treated with tPA + Argatroban (100 μg/kg bolus started during the tPA infusion then followed by a 1 μg/kg/min infusion for 48 h) compared to matched controls treated with tPA alone [18]
The ARTSS-2 clinical trial is a phase IIb, randomized, multi-center trial to estimate benefit (improvement in disability) among stroke patients treated with standard full dose rt-PA who are randomized to also receive either lower-dose Argatroban, higher-dose Argatroban or neither [19]. Targets for PTT will be 1.75 for lower-dose Argatroban (same algorithm as ARTSS-1 study) and 2.25 for higher-dose. As of October 2013, enrollment is at 44 % of target with an estimated trial completion date of end 2014 or early 2015.
Combination Intravenous Thrombolysis with Transcranial Ultrasound
Ultrasound energy in-vivo potentiates IV rt-PA by temporarily separating strands of fibrin allowing for more rt-PA to reach binding sites. This mechanism has been described as “plasma streaming”. In a phase II clinical trial, FDA-approved diagnostic equipment (2-MHz frequency) augmented the effects of rt-PA in the CLOTBUST study (Combined Lysis Of Thrombus in Brain ischemia Using transcranial ultrasound and Systemic RT-PA) [20]. The term sonothrombolysis is now used to describe ultrasound-associated clot lysis. In the CLOTBUST study, 2-h of 2-MHz TCD aimed at the proximal MCA was safe (i.e., no increase in sICH) and resulted in a significant increase in the percentage of patients with complete MCA recanalization (38 % versus 13 %, P = 0.002). Although not powered for clinical efficacy, there was a non-significant 13 % absolute increase in 3-month modified Rankin Scale (mRS) 0 or 1 the ultrasound arm (42 % versus 29 %, P = 0.2).
After CLOTBUST, several sonothrombolysis ischemic stroke studies were reported. In an attempt to increase the transmitted energy, some studies tried using lower frequencies of ultrasound which penetrated the skull better, but unfortunately demonstrated unsafe bioeffects [21]. Others combined intravenous microbubbles with the ultrasound and rt-PA in an attempt to further enhance clot dissolution. Microbubbles (also referred to as microspheres) oscillate in shape and can also cavitate releasing more energy along tissues exposed to ultrasound. The resultant momentum of bubble movement can increase residual flow through the thrombus, thus promoting recanalization. Molina et al. published their safety trial of galactose-based microbubbles, 2-MHz ultrasound and rt-PA [22]. In their trial, three different arms were tested in 0–3 h MCA strokes (n = 111): rt-PA alone; rt-PA + ultrasound and rt-PA + ultrasound + microbubbles. The microbubble arm proved to be safe (no increase in sICH) and resulted in a significantly higher 2-h complete recanalization rate of 54 %. The TUCSON (Transcranial Ultrasound in Clinical SONothrombolysis) trial also tested the safety of rt-PA, ultrasound and escalating doses of intravenous microspheres using a lipid-based shell which is more consistent in size and resistant to degradation during transpulmonary passage [23]. The study was terminated in the second dose tier after the occurrence of three sICH (27 %). However, tier one (one vial of microspheres) experienced no safety issues and a rate of 67 % complete recanalization at 2-h.
Tsivgoulis et al., published a comprehensive review and meta-analysis which identified six randomized (n = 224) and three non-randomized (n = 192) sonothrombolysis stroke studies [24]. When compared to IV rt-PA treatment alone, the pooled rate of sICH in randomized studies did not demonstrate a bleeding risk. Complete recanalization rates were higher in patients receiving combination of TCD with rt-PA, 37.2 % (95 % CI, 26.5–47.9 %) compared with patients treated with rt-PA alone 17.2 % (95 % CI, 9.5–24.9 %). After excluding the low-frequency sonothrombolysis trials, the authors studied the eight remaining studies of high-frequency (i.e., 2-MHz) ultrasound-enhanced thrombolysis. Ultrasound with or without microspheres was associated with a higher likelihood of complete recanalization (pooled OR, 2.99; 95 % CI, 1.70–5.25; P = 0.0001) when compared to IV rt-PA alone. Results continue to justify scientific rationale for continued study of sonothrombolysis in acute ischemic stroke.
To address a frequent concern regarding TCD ultrasound enhanced lysis, namely its operator-dependence, an operator-independent device has been developed. Through NIH-funding, the safety of a novel, hands-free device has been tested in stroke-free volunteers as well as acute ischemic stroke patients. The device requires no ultrasound training and is placed on the head following anatomical landmarks. Eighteen different 2-MHz probes (six on each TCD skull window) are sequentially activated at the same energy level as the original CLOTBUST study and targeted at the proximal intracranial vessels.
A total of 15 stroke-free volunteers underwent clinical and MRI permeability imaging before and after 2-h of hands-free device insonation. All subjects were safely insonated with no adverse events or abnormalities of the blood brain barrier demonstrated [25]. In a small, phase II study, 20 acute stroke patients receiving IV rt-PA with intracranial occlusions (mean age = 63; median baseline NIHSS = 15) also received 2-h of TCD ultrasound using the hands-free device [26]. All patients underwent repeat vascular imaging (TCD or CT-Angiogram) after removal of the device. No cases of sICH occurred. Rate of 2-h complete recanalization was 40 %, but with wide 95 % confidence intervals (19–64). At 90 days, 25 % (95 % CI 7–49) of the patients had a mRS of 0–1. Successful operator-independent ultrasound delivery makes a large phase III efficacy trial of sonothrombolysis feasible (Fig. 3.2). In fact, such a trial is currently underway in a large, 830 patient trial [27].
Combination Intravenous Thrombolysis with Endovascular Therapy
As stroke follows in the footsteps of interventional cardiology, endovascular procedures to reperfuse the brain have undergone dynamic technological changes in a short period of time. The success of endovascular procedures reflect what is known about IV rt-PA—time is brain. In a review of data from the IMS-1 and 2 studies, Khatri and colleagues clearly show that the longer the procedure duration, the worse the outcomes [28]. The progress of device advancement shows how the technology has improved recanalization effectiveness (from Merci™, to Penumbra™, to now stent-assisted reperfusion): 56 %, 83 %, and 100 % respectively [29].
The IMS-3 (Interventional Management of Stroke) trial planned to randomize (2:1 ratio) 900 patients to either 0.6 mg/kg IV rt-PA + endovascular therapy or IV rt-PA alone (0.9 mg/kg) [30]. The trial was halted after 656 patients were enrolled due to futility. The bridging IV-IA approach unfortunately did not yield any better outcomes compared to IV rt-PA alone: the absolute difference between groups was 1.5 % (95 % CI: −6.1 to 9.1 %), adjusted for baseline severity as measured with the NIHSS. Further review of endovascular approaches for acute ischemic stroke are described in greater detail in Chap. 7.
Rationale for Combination Thrombolysis and Cytoprotection
The past decade has seen the emergence of the concept of the “neurovascular unit”, or the integrated function of blood elements, endothelium, glia and neurons, as a major target of ischemic injury. With the failures in clinical trials of drugs that targeted mainly neuronal perturbations after ischemia, the field is now turning to therapeutic approaches that target the neurovascular unit and its multiple cell lines. These therapies are therefore more appropriately called “cytoprotective”.
Reperfusion Injury
Although their overall effect is positive, drugs that produce reperfusion, especially thrombolytics, have well-known adverse effects. These include bleeding into ischemic regions or aggravation of infarct-related cerebral edema, both likely the result of increased hydrostatic pressure applied to a damaged blood-brain barrier and leakage of toxic serum components into ischemic brain. Plasminogen activators themselves may also increase vascular and neuronal injury through up-regulation of matrix metalloproteinases (MMPs) [31]. These side effects of thrombolysis might be prevented or reduced by therapies that protect the integrity of the vascular endothelium such as free radical scavengers or anti-adhesion molecules, which prevent leukocyte-mediated injury, or MMP inhibitors.
A less well-understood adverse consequence of reperfusion is cytotoxicity associated with reperfusion of moderately injured brain tissue. There are several explanations for this so-called “reperfusion injury”. First, it has been shown that reperfusion is associated with a secondary wave of glutamate and other neurotransmitter release and consequent movement of calcium intracellularly resulting in excitotoxicity [32]. Second, restoration of blood flow may be sufficient to allow synthesis of damaging proteins and other cytokines. Third, reperfusion may supply oxygen to ischemic regions which provides a substrate for peroxidation of lipids and free radical formation [33].
We have been able to demonstrate reperfusion injury in a rat model of moderate ischemia produced by tandem occlusion of the ipsilateral common carotid and middle cerebral arteries (MCA) [34]. Opening the MCA and allowing reperfusion following 2–5 h of MCA occlusion is associated with substantial infarction when measured after 24 h, whereas leaving the MCA permanently occluded was associated with very little damage even if histological evaluation was deferred up to a week to allow for the appearance of delayed cell death. Reperfusion injury may have an inflammatory basis and combining reperfusion with appropriate neuroprotective agents, which achieve therapeutic levels by the time the artery recanalizes, might improve the outcome beyond what can be achieved by thrombolysis alone.
Laboratory Studies of Combined Neuroprotection and Thrombolysis
Studies directly examining the role of combining neuroprotection with thrombolysis have been limited by the paucity of autologous clot animal models in which to test thrombolytic drugs. One of the first such studies was carried out by Zivin and colleagues [35] in the rabbit embolism model. The rabbit model involves a simple cutdown in the neck, occlusion of the external carotid artery, and implanting a cannula in the common carotid artery to inject particles. After the surgery is complete, the animal is awake and unanesthetized, so many aspects of physiology that might influence neuroprotection, such as a blood pressure, body temperature, and blood gases, are under normal physiologic regulation.
To study ischemic stroke, blood is taken from a donor animal, allowed to form clots, and injected into the carotid circulation. This injection produces clots in end vessels in the brain. Tracer amounts of radioactive microspheres are injected with the clots and are used to measure the specific activity of the brain and to determine how many clots lodge in the brain. The result is small infarcts in random locations throughout the brain.
Treating with rt-PA at 15, 45, and 30 min after clots were injected demonstrated that treated animals tolerated about three times more clots than the control animals. However, if treatment was delayed for 1 h, the curve was not significantly different from the control curve. This was the first demonstration in a clinically applicable model that rtPA reduced neurologic damage.
This model was used in a study of rt-PA and MK-801, which is a glutamate antagonist [36]. MK-801 was given at 1 mg/kg 5 min after embolization. rt-PA was given at 1 mg/kg at various later times. MK-801 alone had no significant effect. rt-PA alone at 60 min after embolization nearly doubled the amount of clots the animal could tolerate. Giving both drugs at 60 min was more effective than rt-PA alone, even though MK-801 was ineffective alone. Delaying rt-PA treatment to 90 min did not produce a therapeutic effect.
This model was also used to study combination therapy with an anti-ICAM-1 monoclonal antibody plus rt-PA [37]. Whereas the rt-PA/MK-801 study was designed to increase the amount of clot the animal could tolerate, the t-PA/anti-ICAM-1 study attempted to increase the window of time until start of treatment. Data showed that the combination could extend the time window to 2 h, whereas neither drug alone was effective when treatment was initiated at 2 h. This is evidence for a positive interaction of the combination.
Zhang and colleagues [38] also developed an autologous clot occlusion of the MCA in rats. Treatment with rt-PA alone improved neurologic deficits and reduced mean infarct volume compared to controls when the drug was started within 2 h of arterial occlusion, but had no benefit when treatment was delayed out to 4 h. The anti-CD18 antibody, which presumably prevents white blood cell adhesion to ischemic endothelium and consequent inflammatory damage to surrounding brain regions, had no effect when administered alone at either time point. However, when both rt-PA and the anti-CD18 antibody were given together, there was substantial reduction of neurologic deficit even when combined treatment was delayed out to 4 h. Thus, the synergism of this particular combination was especially notable in extending the time window of effective therapy.
One common feature of virtually all preclinical neuroprotection studies, however, is the overall brief time window for efficacy, whether or not combined with thrombolysis. This argues for simultaneous administration of any cytoprotective therapy with rt-PA within the approved 3 h time window, or even better, pre-clinical treatment [39].
Clinical Studies of Combined Neuroprotection and Thrombolysis
To date, only three clinical studies specifically designed to evaluate the effect of combining thrombolysis and neuroprotection in acute stroke patients have been completed, and none were positive. Lubeluzole was the first potentially neuroprotective agent to be evaluated in a dedicated combination trial with rt-PA. Patients who qualified for and received intravenous rt-PA within 3 h of symptom onset were randomly allocated 1:1 to lubeluzole (7.5 mg IV over 1 h, then continuous 5 day infusion of 10 mg/day) or placebo [40]. Lubeluzole infusion was started before the end of the 1-h rtPA infusion so that therapeutic blood levels would be achieved by the time reperfusion from the rtPA was likely to occur. The study was designed to evaluate the safety of the combination, particularly to ensure that the incidence of hemorrhage was not increased (and perhaps decreased), and served as a companion to a larger evaluation of lubeluzole given as monotherapy 8 h following stroke onset. Eighty-nine patients were enrolled prior to the early trial termination based on the negative results of the concurrent lubeluzole phase 3 trial. In the enrolled patients (45 % of the planned population), rt-PA and study drug were administered at a mean of 2.5 and 3.2 h from symptom onset, respectively. There were no significant differences in mortality (26 %), intracerebral hemorrhage (10 %), serious adverse events (51 %) or functional outcomes (Barthel Index) between lubeluzole and placebo. These results demonstrated the safety and feasibility of linking ultra-early neuroprotection with thrombolysis, however the premature stoppage of enrollment led to a study underpowered to detect efficacy.
The second trial to determine whether the effect of thrombolysis may be augmented by pharmacologic neuroprotection evaluated the use of a GABA agonist, clomethiazole (CLASS-T) trial [41]. Whereas a large study of clomethiazole monotherapy compared to placebo was conducted, this smaller pilot study was designed to test the combination of rtPA given within 3 h, and clomethiazole given within 12 h of stroke onset. This study randomized patients in a double-blind fashion to receive rt-PA and either 68 mg/kg clomethiazole or placebo over 24 h. The study included 97 patients given clomethiazole and 93 patients given placebo; all patients received rt-PA first according to NINDS protocol. Overall, there was no benefit seen. Since administration of clomethiazole was not required prior to completion of rt-PA infusion, many patients received clomethiazole several hours after thrombolysis. This limitation highlights the need to require linkage of rt-PA and neuroprotectant administration in future trial design of combination approaches.
The most recently completed combination trial was ARTIST + (AMPA Receptor Antagonist Treatment in Stroke plus rt-PA). This multicenter, randomized, double-blind, placebo-controlled trial was designed to compare the efficacy of YM872 plus rt-PA to that of placebo plus rt-PA. The planned enrollment was 600 patients and over 400 patients were enrolled. The drug was started before the end of rt-PA infusion and continued for 24 h. Enrollment was terminated prematurely based on an interim futility analysis. The results have never been published.
Numerous other neuroprotective trials were carried out that allowed treatment with rt-PA if clinically indicated, so that some patients in these studies received combination therapy. One example of such a trial is the GAIN Americas study of gavestinel, a novel glycine site antagonist at the NMDA receptor complex [42]. The GAIN Americas trial randomized 1,367 patients within 6 h of stroke onset and concomitant treatment with intravenous rt-PA was allowed in eligible patients. No statistically significant difference in mortality or 3-month outcome measures (Barthel Index, mRS or NIHSS score) was found between the groups. Trials of other neuroprotectant agents (NXY-059, ONO-2506, Albumin, and Granulocyte Colony Stimulating Factor among others) that allowed but did not require rt-PA administration were also negative, but in these studies the experimental agent was rarely given within the first 2–3 h after stroke onset [39].
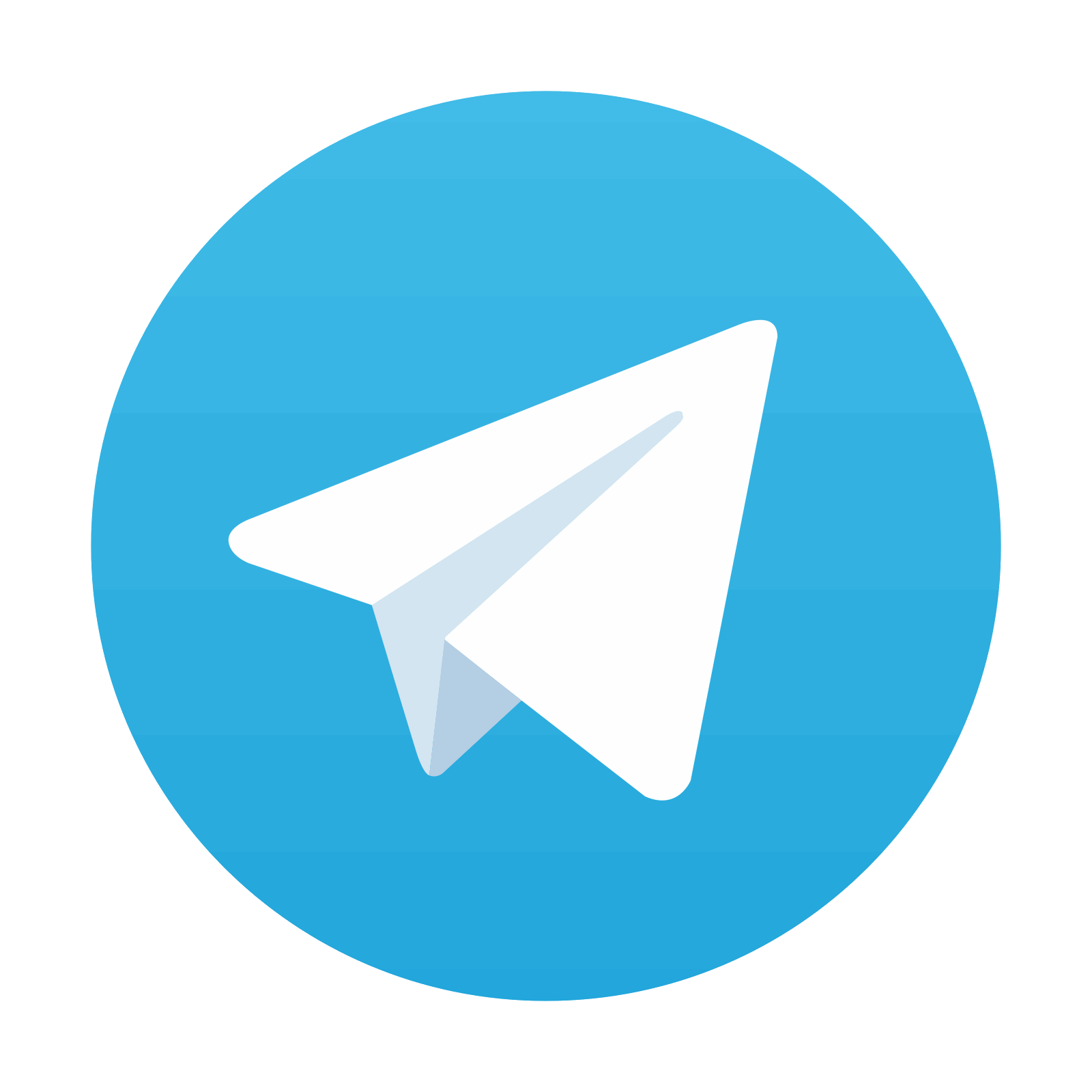
Stay updated, free articles. Join our Telegram channel

Full access? Get Clinical Tree
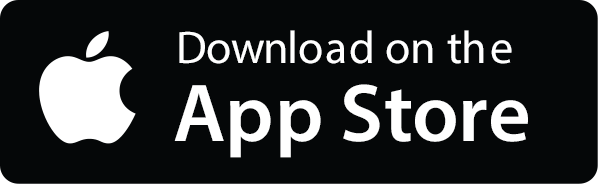
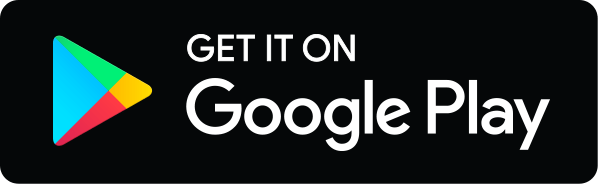