Computed Tomography and Myelography of the Cervical Spine
Christopher G. Ullrich
The first computed tomography (CT) scanner became commercially available in 1972. It was capable of scanning only the head. The machine revolutionized neuroradiology. The first whole-body CT scanner was marketed in 1974. The thin-section capability (1.5 mm) necessary for high-quality cervical spine CT evaluation was introduced in 1977. Two-dimensional multiplanar reconstruction imaging (2D-CT) was developed in the late 1970s.
2D-CT images are produced by reprocessing axial CT image data into other tomographic projections, typically sagittal and coronal views. Creation of curved 2D-CT reconstruction images became possible in the mid-1980s. Threedimensional CT image technology (3D-CT) was pioneered in the 1980s as well. 3D-CT images are computer graphic displays that integrate axial CT image data into lifelike images (Fig. 24.1). This technique is directly related to the prior development of computer-aided design and manufacturing systems for industrial applications. Continuous-rotation CT scanners, commonly called helical or spiral CT scanners, were widely produced in the early 1990s. The first commercial helical 4-channel multidetector CT (MDCT) was introduced in 1998. This was followed by the subsequent development of 8-, 16-, 32-, 40-, 64-, 128-, 256-, and 320-channel MDCT systems. Dual energy source CT scanners that utilize two different kilovoltage x-rays in the same data acquisition have also been developed. These advanced CT systems can image the entire cervical spine in less than 2 seconds, while producing superior-quality isotropic volumetric image data that facilitate very useful 2D and 3D-CT image displays. Real-time image data processing is also a reality today. CT remains a very important modality for cervical spine studies.
Myelography is a term that denotes imaging techniques involving the injection of a contrast agent into the subarachnoid space to provide visualization of the spinal cord and intrathecal nerve roots. Air myelography was introduced in 1920; combined with conventional tomography, it was quite capable of demonstrating the outline of the spinal cord well. Iophendylate (Pantopaque) was developed in 1940. This material was insoluble in spinal fluid and quite dense. It required removal at the end of the imaging procedure, a process that was often unpleasant for the patient. Pantopaque provided a good assessment of the cervical spinal cord and nerve roots and was the preferred myelographic examination agent until the early 1980s. In 1978, metrizamide (Amipaque) became the first water-soluble myelographic agent available. It came in a lyophilized form and had to be reconstituted immediately before the myelogram was performed. Unlike Pantopaque, it did not require removal from the subarachnoid space at the end of the procedure. More important, it was suitable for concurrent use with CT. Iohexol (Omnipaque) and iopamidol (Isovue) are second-generation water-soluble myelogram agents, both introduced in 1986. They are stable in mixed form and less neurotoxic than metrizamide. The combination technique of CT with myelography (CTM) remains a powerful method for evaluating the cervical spine today.
The basic technology of CT imaging and the derivation of 2- and 3D-CT reconstruction images are reviewed in this chapter. Myelography, with emphasis on its combined use with CT, is discussed. Imaging principles are illustrated. A brief review of clinical applications of CT concludes this chapter. The appropriate relationship between magnetic resonance imaging (MRI) and CT is woven into the text of this chapter and the “Magnetic Resonance Imaging of the Cervical Spine and Spinal Cord” chapter, which follows this chapter.
BASIC COMPUTED TOMOGRAPHY TECHNOLOGY
The axial CT image is defined by a cartesian coordinate system called a matrix. Values for x and y, respectively, denote the horizontal and vertical edges of the axial CT matrix. The pixel (picture element) is the basic unit of a matrix. The voxel (volume element) is the volume of tissue defined by the pixel and the CT image thickness. The matrix position of each voxel is mathematically described by x and y coordinate numbers. Based on the detected x-ray attenuation data, the CT scanner calculates and assigns an appropriate Hounsfield number to each voxel. By designating shades ofgray for various Hounsfield number values, the familiar CT video image display is created. The z-axis mathematically describes the movement of the CT
scanner table and therefore defines the spatial relationships among successive axial CT images. A consecutive series of axial CT images may be considered a 3-D array of voxel or Hounsfield number data for which spatial locations are defined by the matrix, the CT image thickness, and the CT table position at which each successive axial CT image is obtained. The computer precisely identifies the spatial location of each voxel by x, y, and, z coordinate numbers.
scanner table and therefore defines the spatial relationships among successive axial CT images. A consecutive series of axial CT images may be considered a 3-D array of voxel or Hounsfield number data for which spatial locations are defined by the matrix, the CT image thickness, and the CT table position at which each successive axial CT image is obtained. The computer precisely identifies the spatial location of each voxel by x, y, and, z coordinate numbers.
Two-dimensional CT multiplanar reconstruction images are produced by selecting the appropriate voxel data from each successive axial CT image to create a “new” tomographic view. Selecting voxel data in the yz-plane produces a sagittal view; xz-plane data represent a coronal view (Fig. 24.1). These images can be no better than the quality of the axial CT image data from which they are derived. “Targeted” algorithms produce the best axial CT images of the cervical spine. The 2D-CT reconstruction images have the same contrast resolution as the original axial CT images; they also retain the spatial resolution of the axial CT images along the x- and y-axes. However, spatial resolution along the z-axis, which is only subtly evident on the axial CT images, is prominently displayed on the 2D-CT reconstruction images. The z-axis spatial resolution, which is directly related to the CT image thickness and the CT table incrementation, is usually inferior to the spatial resolution obtained along the x- and y-axes of the original axial
CT image. As a general principle, the quality of a 2D-CT reconstruction image is dramatically improved by use of thinner axial CT images and smaller CT table increments. If the CT gantry is angled for the axial images, a software correction must be used; otherwise, the reconstruction image will be spatially distorted.
CT image. As a general principle, the quality of a 2D-CT reconstruction image is dramatically improved by use of thinner axial CT images and smaller CT table increments. If the CT gantry is angled for the axial images, a software correction must be used; otherwise, the reconstruction image will be spatially distorted.
Three-dimensional CT images are commonly produced by a surface contour method. Simply described, the voxels in each CT image that define the edge of the structure to be displayed are identified. The computer does this by identifying all voxels that have Hounsfield numbers above or below a threshold value selected by the system operator. By drawing a line connecting each edge-defining voxel, the contour (outline) of the surface anatomy is obtained. Because the relationship between successive axial CT images is also known, a spatial or 3-D array of data describing the anatomical outline is defined. If a reference light source is assumed, shading is introduced to the data, and the visual perception of three dimensions is created (Fig. 24.1C). Unlike 2D-CT images, these contour 3D-CT images provide no density discrimination beyond locating the surface-defining voxels based on the selected threshold Hounsfield number value. Once created, these 3D-CT images may be rotated to allow evaluation from many perspectives (Fig. 24.1D). The images may be sectioned or edited to reveal internal surface details (Fig. 24.2E). Color coding may be used to highlight anatomic relationships. The technical factors that improve 2D-CT reconstruction images have a similar impact on 3D- CT images. The 3-D images add no new information, but they do display certain aspects of the axial CT data to better advantage.
Volumetric 3D-CT image processing classifies tissues into different ranges of CT values. It preserves the range of CT values within the classified tissue. In a sense, it maintains tissue texture rather than merely defining its surface as does contour processing. Volumetric processing requires more complicated computer computations than contour methods. However, it allows a variety of additional image processing techniques to be used, including variable transparency and ray projection imaging. Images resembling conventional radiographs or CT angiograms can be produced from the CT data. If bone surface displays are used, the contour and volumetric techniques yield images of very similar appearance. All the 3D-CT images that illustrate this chapter were produced using volumetric processing. A detailed discussion of this topic is beyond the scope of this chapter.
Until the advent of helical CT scanners, all CT data were acquired as discrete “slices” localized in space by the patient table position where the image was made. Helical CT produces a seamless volume of CT data by combining a continuously rotating CT x-ray source with a smoothly advancing CT patient table. Typically, the CT x-ray tube rotates 360 degrees every second. The CT patient table advances continuously at a constant velocity measured in millimeters per second. Pitch is the term that describes the relationship between the speed of the CT table and the thickness of the acquired CT images. If the image thickness is 3 mm and the table velocity is 3.0 mm/s, the pitch is 1.0. If the image thickness is 3 mm and the table velocity is 6.0 mm/s, the pitch is 2.0. For most cervical spine applications, a pitch of 1.0 or 1.5 is used with image thicknesses of 1.0 to 3.0 mm.
MDCT involves the simultaneous acquisition of up to 320 data channels per CT gantry rotation, thus allowing rapid high-resolution scanning over large anatomic areas. Data acquisition times of less than 2 seconds for the entire cervical spine are possible with 64-channel MDCT systems. Patient motion imaging artifacts are minimized by this rapid data acquisition. Patient radiation exposure reduction strategies are also facilitated by MDCT. This seamless volume of CT data is typically composed of isotropic voxels that have equal spatial resolution in the x, y, and z axes. Each voxel is a very small cube within a 3-D data matrix. The axial, 2D, and 3D images derived from this data basically have equal spatial and contrast resolution in all planes. It is this isotropic image data that enable a variety of sophisticated image analysis and clinical applications. Real-time 2D and 3D image processing is now readily available at a workstation or via a web-based network accessible server. CT angiography is made possible by the ability to differentiate and display bloodvessels in relationship to adjacent structures. Intraoperative image-based guidance is a widely available capability. Intraoperative CT, MR, and angiography are now commonplace. Implants can be preoperatively sized with great accuracy using CT data. Custom implants can be fabricated from CT data. Stereotactic radiation therapy depends on volumetric image data for both treatment planning and guidance. Computer-simulated surgery can be accomplished. Active research and development continues in these areas and on many other new applications. All of these amazing developments are dependent on high-quality CT or magnetic resonance data, powerful computers, and sophisticated software. They are best performed using a team approach, combining the skills of the technologist, radiologist, and the treating physician.
COMPUTED TOMOGRAPHY WITH AND WITHOUT INTRAVENOUS CONTRAST
The primary clinical indications for unenhanced CT of the cervical spine are the evaluation of congenital segmentation anomalies (Fig. 24.2), fractures (Figs. 24.3, 24.4, 24.5, 24.6 and 24.7), bony deformities and destructive lesions, bony fusion integrity, central and neuroforamen spinal stenosis, ossification of the posterior longitudinal ligament, and osteoid osteoma. A CT image thickness of 3 mm or less should be used. Helical CT is frequently used for these studies; excellent studies can also be obtained with conventional CT. Two-dimensional CT reconstruction images are routinely prepared in most cases. Three-dimensional CT reconstruction images are used for complicated anatomic circumstances.
The role of CT in the evaluation of patients with significant cervical spine trauma deserves additional comment. The traditional teaching has been to perform a five-view cervical spine radiographic examination: lateral, anterior-posterior (AP), AP open-mouth, and oblique views. If a fracture or questionable finding was identified, a focused CT study would be undertaken. This approach is time consuming. The radiographs obtained by portable technique are frequently suboptimal. Delays in clearing the cervical spine are common. Important cervical spine fractures are occasionally missed, particularly at C0-C1 and C7-T1. Recognizing these limitations, many trauma centers now omit radiography and routinely perform a complete cervical spine CT. This produces a rapid complete evaluation for cervical fractures and dislocations,
but isolated nondisplaced ligamentous injuries may still go undetected. Disk herniation and epidural hematoma are less reliably detected than fractures but can often be identified. This approach is not only time efficient but also relatively cost-effective.
but isolated nondisplaced ligamentous injuries may still go undetected. Disk herniation and epidural hematoma are less reliably detected than fractures but can often be identified. This approach is not only time efficient but also relatively cost-effective.
C1-C2 instability, subluxation, and fixation can be investigated by performing successive CT studies with the patient in the following positions: head neutral, head turned to the right, and head turned to the left. Three-dimensional CT images (360-degree views at 30-degree intervals) of each position are prepared, along with sagittal and coronal 2D-CT reconstruction images oriented to the C2 vertebral body. In children, up to 95% of the inferior articular facet of C1 can extend forward of the C2 superior articular facet
and be considered normal when the head is turned. In adults, this forward movement of C1 on C2 rarely exceeds 80%. The adult atlantoaxial distance should not exceed 3 mm in any position. Rotational movement to the right and left is expected to be symmetric. Torticollis is the most common indication for doing this type of examination. This examination should only be performed using very low radiation dose imaging techniques.
and be considered normal when the head is turned. In adults, this forward movement of C1 on C2 rarely exceeds 80%. The adult atlantoaxial distance should not exceed 3 mm in any position. Rotational movement to the right and left is expected to be symmetric. Torticollis is the most common indication for doing this type of examination. This examination should only be performed using very low radiation dose imaging techniques.
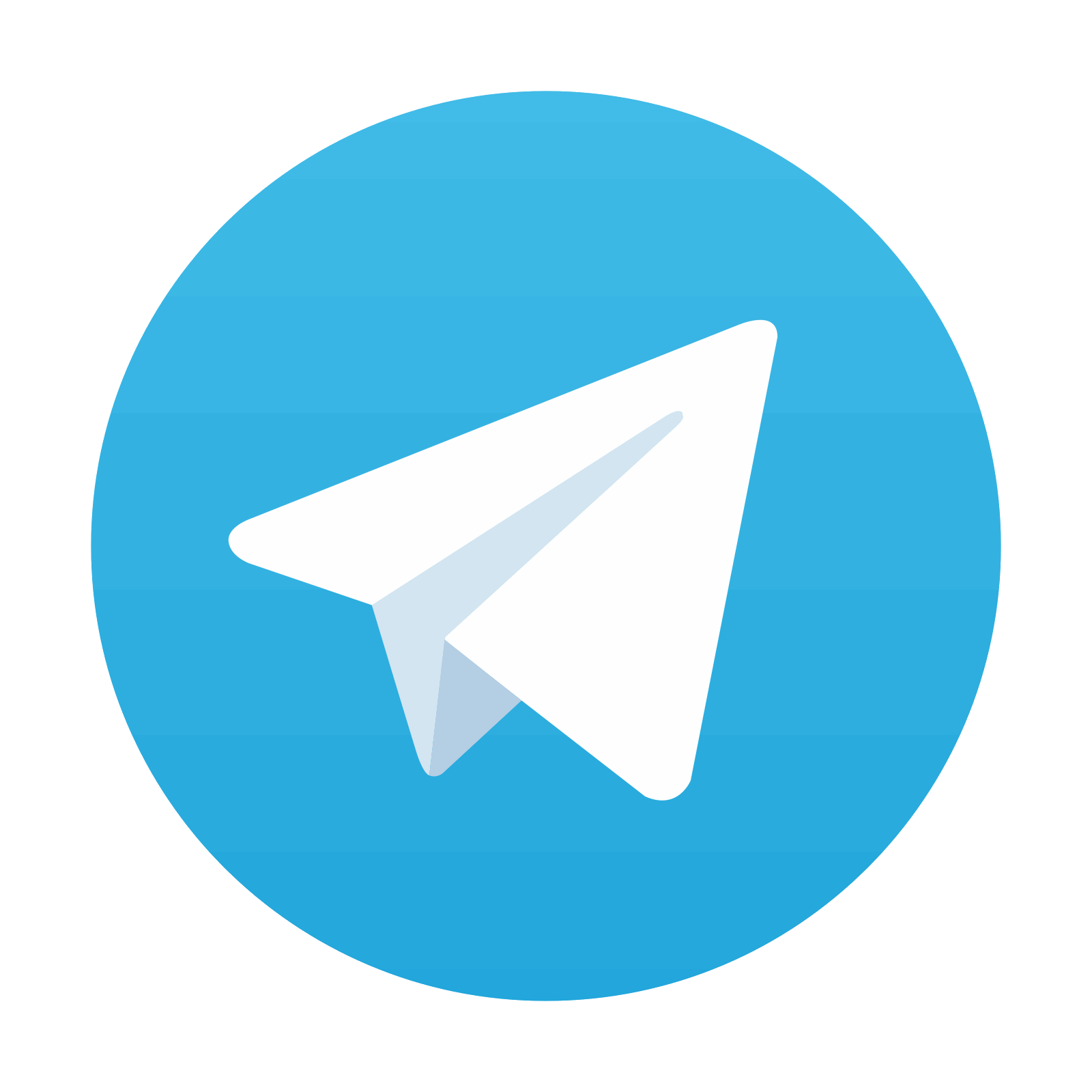
Stay updated, free articles. Join our Telegram channel

Full access? Get Clinical Tree
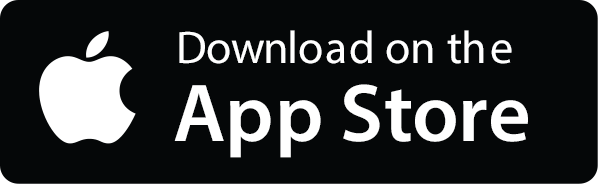
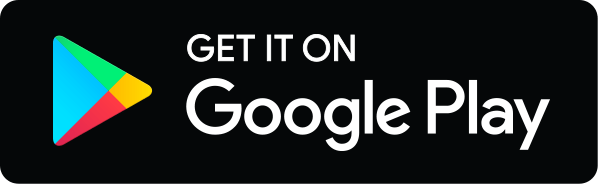