Convection-Enhanced Delivery
As many as 15 to 20% of pediatric central nervous system (CNS) tumors are located in the brainstem.1 These tumors, particularly diffuse infiltrative brainstem gliomas (DIPGs), present a unique management challenge. Due to the infiltrative nature of DIPGs and surrounding eloquent nervous system anatomy, surgical resection is not possible. Although radiation therapy can improve signs and symptoms for a temporary period of time, it is palliative at best. Whereas surgery and radiation are not curative and because these tumors remain uniformly fatal, emerging treatment paradigms have been directed toward investigating and developing targeted glioma-toxic agents. Unfortunately, these putative therapeutic compounds have not been successfully translated into effective treatments as a result of ineffective delivery to the tumor using available drug delivery techniques, including systemic administration, direct injection, and intrathecal/intraventricular delivery.
Systemic delivery is limited by the blood–brain barrier (BBB), as well as systemic dose levels necessary for penetration into the CNS that result in toxicity.2 Local delivery methods for distribution of chemotherapeutic agents have been developed to circumvent both this toxicity as well as the BBB. Direct intratumoral injection, intraventricular or intrathecal infusion, and intracavitary implantation of drug-impregnated wafers have all been attempted for treatment of adult infiltrative malignant gliomas. However, these methods rely on diffusion of drug through tumor and brain. This results in tissue penetration of only 1 to 4 mm and steep drop-off in concentration over this distance,3,4 resulting in small and unpredictable volumes of distribution. To overcome the difficulties associated with these conventional delivery methods, direct convective delivery of infusate through the interstitial spaces using a continuous pressure gradient has been developed and employed.5
Historical Perspective
In 1994, Oldfield′s group5 infused radiolabeled transferrin and sucrose into brain parenchyma of 12 adult cats. Five-centimeter, 23-gauge cannulae were inserted into the bilateral corona radiata of the cats. A pump was used to maintain a constant pressure gradient throughout the infusion. After sacrifice, the infused brains were analyzed with autoradiography, and the volume and the concentrations of infusate were measured at 0, 2, and 24 hours after infusion. Unlike direct interstitial infusion, the early findings of this study indicated that convection-enhanced delivery (CED) allowed for a predictable volume of distribution, a homogeneous tissue concentration described by a “square-shaped” concentration profile with a steep drop-off at the margins of the infusion volume. These and other characteristics allow for precise targeting of intraparenchymal lesions using CED ( Fig. 13.1 ).
Properties of Convection-Enhanced Delivery
The principle of convection describes the movement of molecules within gases and liquids. Convection combines the principles of simple diffusion and bulk flow. Diffusion occurs by random brownian movement of particles, and in fluids it is generally driven by concentration gradients. Bulk flow of fluid is produced by large-scale currents and often driven by pressure gradients. It naturally occurs in brain parenchyma with the spread of vasogenic edema.6 These fluid movements are precisely described by the Navier-Stokes equations, which define general fluid motion and are based on both diffusive and advective forces.7 The concept is simplified in the convection-diffusion equation, which defines convective flux (rate of flow across a given surface):

Convective flux is the sum of diffusive flux (Dᐁ2 u; D is the diffusion coefficient) and advective flux (– v ᐁu; V is the velocity).8
Fluid motion in CED is largely confined to the extracellular compartment ( Fig. 13.2 ); therefore, infusate flow through brain parenchyma or pathological tissue is better defined as flow through a porous medium. This is described by yet another simplification of the Navier-Stokes equations known as Darcy′s law:

Here, flux (Q) is proportional to the product of the permeability of the medium and the pressure gradient vector (ᐁp) divided by the viscosity of the fluid (µ)9 ( Fig. 13.2 ).
Characteristics of Convection-Enhanced Delivery
The properties of convection confer unique advantages to CED in the chemotherapeutic targeting of brain lesions, which are summarized in the following subsections.

Local Delivery
Using stereotactic guidance, putative glioma-toxic agents in CED can be delivered directly to the region of interest. This enables the surgeon both to avoid systemic toxicity and to circumvent the BBB. Systemic toxicity of standard chemotherapeutic agents is often unavoidable to achieve effective concentrations of drug in tissue. As many drugs are designed to be cytotoxic against the rapidly dividing cancer cells, patients often will also experience cytotoxicity against their own rapidly dividing cells, including the skin, gastrointestinal tract, and bone marrow. Local delivery in CED minimizes or eliminates this potential toxicity, as smaller quantities of drug (magnitudes of order less) are required for direct treatment and only a small fraction of the drug is absorbed from the abluminal side of the brain capillaries and enters the systemic circulation. As a result, agents that do not cross the BBB are ideal for CED, because they may remain sequestered on the parenchymal side of the BBB.
Targeted
Because infusate is distributed via a cannula placed in the CNS, CED can be used to target select sites of regions directly for treatment. Distant targets, such as the globus pallidus internus for Parkinson′s disease and the hippocampus for temporal lobe epilepsy have been targeted effectively in vivo.10,11 Further, sites distant from a perfused region can also be affected by anterograde and retrograde projections and transport. Lieberman and colleagues12 demonstrated that wheat germ agglutinin perfused into the striatum was transported by neurons of the substantia nigra in retrograde fashion along the nigrostriatal pathway. Likewise, Kells and colleagues showed that adeno-associated virus 2 (AAV2) encoding for glial cell–derived neurotrophic factor infused into the thalamus resulted in transport of growth factor in anterograde fashion to large connected regions of neocortex ( Table 13.1 ).13

Differences in Gray Versus White Matter
Critical differences in convective distribution exist between gray and white matter. Specifically, although distribution in CNS gray matter is isotropic, distribution in white matter is anisotropic parallel to low-resistance pathways of fiber tracts. By exploiting the convective properties of these white matter fiber tracts, large regions of cortex or spinal cord may be perfused by CED delivered from a single point source (cannula).14,15 Distribution of infusate in gray matter is likewise discrete and can achieve complete filling of cerebral targets including deep brain nuclei.5,11
Volume of Distribution
One of the major limitations of diffusion-based local CNS drug delivery, such as intraventricular polymer implantation or intrathecal and intracavitary delivery, is the limited volume of distribution that is possible with these techniques. Studies of diffusion have shown that as little as 1 to 4 mm of tissue is exposed to drug.4,16 Because CED relies primarily on bulk flow of infusate through interstitial spaces, infused drug is distributed in the extracellular fraction of the CNS tissue, which results in a larger tissue distribution volume (much larger than the absolute volume of infused). This difference is described by the volume of distribution to volume of infusate (V d/V i) ratio. As interstitial space constitutes 15 to 25% of brain parenchymal volume, the V d/V i ratio is generally between 4:1 and 5:1.17
Local delivery avoiding systemic toxicity |
Targeting by anterograde/retrograde transport |
White/gray matter convective differences |
Volume of distribution: greater interstitial volume → larger tissue exposure |
Real-time imaging: can accurately follow delivery of drug |
Tissue concentration |
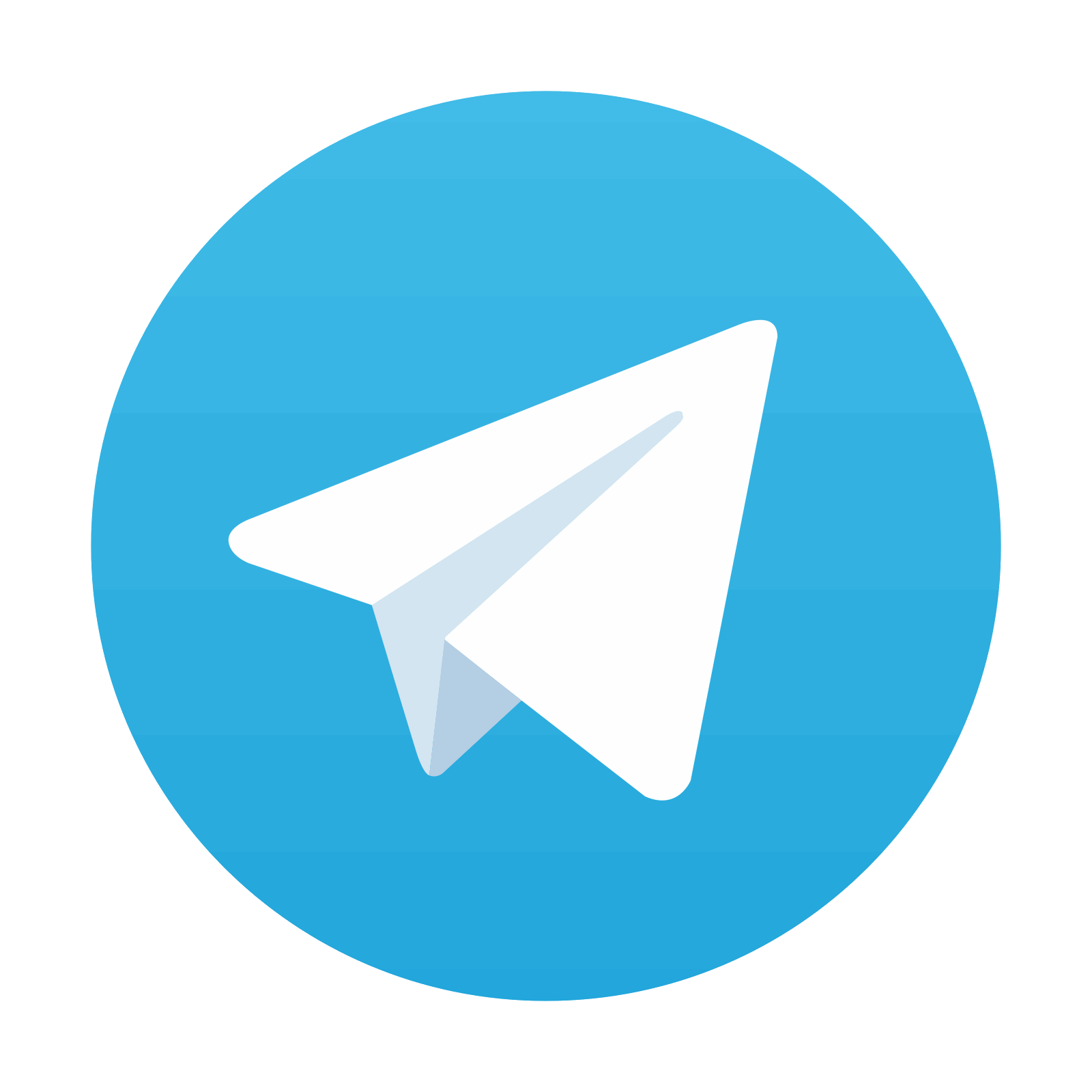
Stay updated, free articles. Join our Telegram channel

Full access? Get Clinical Tree
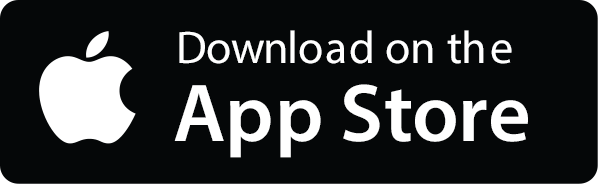
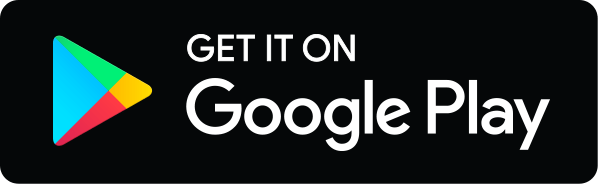
