Cooperative Group Trials for Brain Tumors: Organization and Current Directions
The management of children with primary central nervous system tumors has become increasingly complex. There is general acceptance that optimum management, especially for children with malignant tumors, is multidisciplinary, and that the long-term outcome of children with aggressive tumors is improved by treatment at a tertiary referral (usually “academic”) center.1,2 As therapies evolve, the utilization of multiple modalities of therapy for an individual patient has become commonplace. In addition, as survival rates rise, the needs for evaluation of the quality of life of survivors and the long-term effects of treatment increase. As newer forms of treatment are purported to improve survival and quality of life, it is necessary to determine rigorously if a new or different treatment is more efficacious than conventional treatments or causes fewer sequelae but has equal efficacy. These types of decisions often require more patients than can be gathered at one institution or, for that matter, at multiple institutions working in concert. This has led to larger national or international studies evaluating patients in a standardized fashion.
Since the 1960s, multi-institution groups in North America and Europe have evaluated children with cancer.1,2 Initially, these groups focused on leukemia and other lymphoreticular malignancies. Soon thereafter, when the benefits of such group studies became evident, other pediatric tumors were evaluated. Trials for children with brain tumors have been coordinated by these cooperative groups since the mid- to late 1970s, although the involvement of such cooperative groups in pediatric brain tumor studies lagged behind activities for other pediatric cancers. The reasons for the relative delay of multi-institution study groups being involved in childhood brain tumor studies are manifold and include (1) the standard practice, in the past, of brain tumors to be considered the treatment domain of the neurosurgeon or less commonly another single caregiver (such as a neurologist); 2) the nihilistic mindset of many taking care of children with brain tumors, often derived from the experience of caring for adults with brain tumors, concluding that therapy, other than surgery, is usually futile and only adds to the discomfort of the patient; (3) the lack of expertise within the cooperative group of physicians who are knowledgeable about the nuances of primary central nervous system tumors, and the central nervous system, in general; and (4) the reluctance of oncologists and other cancer specialists to focus on brain tumors because of their relative rarity, as regards individual tumor types, and the lack of effective treatments. Recently, many of these obstacles have been overcome, as the multidisciplinary needs of the patients and encouraging survival rates in some subsets of patients have led to the wider willingness to referral patients to academic cancer centers ( Table 45.1 ). In many medical centers, and for most insurers in the United States, clinical trials are now considered the standard of care. The cooperative groups have greatly expanded their expertise in pediatric brain tumors and have actively recruited neurosurgeons, neurologists, psychologists, and other neuroscientists to join the group and participate in the creation of treatment protocols. Also, there is a new generation of pediatric oncologists whose primary academic pursuit is neuro-oncology.
The potential power of such groups is highlighted by the patterns of referral of children with cancer. Although cancers in patients older than 21 years of age greatly outnumber those occurring in pediatric patients, nearly 20% of all patients entered in cooperative treatment trials in the United States are of the pediatric age.1,2 In 1996, pediatric accruals on National Cancer Institute–sponsored trials comprised 18.6% of all patient studies, whereas pediatric cancers represented less than 2% of all cancers. Nearly 95% of children with cancer are referred to an academic cancer center participating with one of the two major cooperative groups within the United States. It is estimated that over 80% of these patients seen at an institution that is affiliated with one of the cooperative groups will be eligible for entry in an appropriate study. Over the past decade, there has been an exponential rise in the number of patients with brain tumors entered in cooperative group studies. These studies, which initially focused on the more common childhood brain tumors such as medulloblastoma, have increasingly encompassed other “rarer” forms of brain tumor. Initially, the cooperative groups were reluctant to evaluate patients with benign tumors, such as low-grade gliomas; but more recently, these studies have been developed and successfully completed. Probably even more importantly, it has been accepted that a major reason for the lack of progress in the management of childhood brain tumors is the lack of understanding of the basic neurobiological underpinnings of these tumors. Given the histological heterogeneity of childhood brain tumors, individual institutions are hard pressed to gather a significant number of specimens, from well-characterized and homogeneously treated patients, on which to perform biological studies. Centralized reference or resource laboratories have been developed by the cooperative groups to promote and facilitate neurobiological studies of childhood tumors.
More important than the other concerns are the potential benefits for individual patients of entry in cooperative group protocols. Studies in children with leukemia, lymphoma, Wilms tumor, rhabdomyosarcoma, and medulloblastoma have shown that stepwise increases in survival result from well-formed, sequential studies.1,2 Children with such tumors have a significant survival advantage when treated at tertiary care centers and enrolled in well-defined protocols, compared with pediatric patients not enrolled in protocols and not treated at pediatric cancer centers.1,2 These results are not to detract from the important information that can be gained from single-institution or smaller multi-institution studies. Smaller studies are an important avenue for new approaches to be tested, and, because of the complexity and availability of resources and of new technologies, some treatment approaches can be performed only by a group of dedicated investigators and are probably inappropriate, especially in initial testing, for cooperative group trials. Even this is changing, as cooperative groups, focusing on innovative, complex, and technologically challenging studies have been formed. Also, cooperative group studies, by design, are often compromise studies, and if treatments are performed on a group-wide basis, there may be the tendency to make the study less “cutting edge” or complex, so as to allow more uniform group participation. An alternative view of the need to make studies performable by the majority is that this creates studies that are potentially beneficial to more patients than are smaller, new technology-based approaches.
Strengths |
Multi-institutional |
Multidisciplinary |
Larger numbers (can do phase III studies) |
Statistical office (funded) |
Data monitoring in place |
Ability to do central reviews |
Rapid incorporation of oncological advances |
Orderly review of studies |
Increased interest in brain tumors in the oncologic community |
Weaknesses |
Variability in expertise of institutions |
Loss of individual institution control |
Need for central review |
Design of studies to (lowest?) common denominator of institutions |
Slower incorporation of new techniques not widely available |
Somewhat cumbersome in study initiation (delay?) |
Somewhat less interest in less common brain tumor |
A major focus of group studies is the development of treatments that can be compared with standard treatments and proven, in a statistical fashion, to be superior or inferior to previous treatments. This, in some sense, limits the type of studies that can be undertaken, and leads to the belief that extremely rare tumors, for which large enough numbers probably cannot be accrued to result in statistical differences in overall survival, are better not evaluated by groups. However, even in these rarer tumors, there is increased pressure by investigators to attempt multi-institution studies, as this may be the only means to accrue enough patients to address management issues.
A major asset of cooperative group studies, as financial resources for academic studies shrink in the United States and probably around the world, is the ability of the groups to provide data management and statistical support to address the important management issues in pediatric brain tumors.
Groups and Group Structure
General Aspects (Table 45.2)
In North America, two major nationally funded pediatric cooperative groups, the Children′s Oncology Group (COG) and the Pediatric Brain Tumor Consortium (PBTC), have been in place since the mid- to late 1990s. The COG, which was created by a merger of the Children′s Cancer Group (CCG) and Pediatric Oncology Group (POG), is larger, and focuses predominantly, but not exclusively, on the treatment of newly diagnosed patients. The PBTC is smaller and is predominantly focused on the use of innovative agents, often biological therapies, for children with recurrent brain tumors and for some tumor types, in subsets of patients with newly diagnosed tumors. The PBTC, in many cases, is designed as a feeder for new studies through the COG.
The International Society of Paediatric Oncology (Société Internationale d′Oncologie Pédiatrique [SIOP]) was initially primarily composed of European centers, but more recently has become an international organization, which acts as a professional society for those individuals involved in the management of childhood cancer. SIOP has been involved for many years in the development of studies of children with brain tumors and helps organize multi-institution and multinational trials, depending primarily on the cancer groups already in place in different countries. For this reason, strong national cancer groups occur across Europe including the United Kingdom (Children′s Cancer and Leukaemia Study Group [CCLG; formerly UKCCSG]), Germany, France, and Italy. The bulk of funding for SIOP studies comes from individual national cancer groups rather than from a centrally funded government organization, as is the case with COG or PBTC studies. Most recently, the European Network for Cancer in Childhood and Adolescents (ENCCA) has been established to promote networks of excellence across Europe based on further developing the capacity to deliver clinical trials and associated platforms of scientific and outcomes research.
Other cooperative groups have been formed and have organized trials for children with cancer outside of North America and Europe, although, with the international reach of COG and SIOP, countries outside of Europe often are involved in studies with these two larger groups. Study groups in Japan and in countries in South America have developed important clinical trials, with the Japanese group taking the lead in the design and completion of studies of children with germ cell tumors. However, these other study groups are newer and tend to have fewer resources than do COG and SIOP.
Children′s Oncology Group
The COG is an international research organization, primarily funded by the National Cancer Institute, which was founded in 1955 as the Children′s Cancer Group. The COG was formed by a merger of the Pediatric Oncology Group and the Children′s Cancer Group in the late 1990s. The CCG was known as the Children′s Cancer Study Group between 1965 and 1990. The COG consists of academic cancer centers throughout the United States and Canada, with 120 international associate members in South America, Australia, the Middle East, and throughout the world. COG members include over 5,230 pediatric cancer specialists in 13 disciplines located in 203 full member institutions. Its primary statistical and operations office is located in Los Angeles, with a group chair′s office in Washington, DC. POG, like CCG, was multi-institutional, with centers in both the United States and Canada. It was younger than CCG, submitting its first grant request in 1991 and began as part of the Southwest Cancer Chemotherapy Study Group and later the pediatric divisions of the Southwest Oncology Group. Prior to the creation of COG, both CCG and POG had an independent multidisciplinary brain tumor strategy group. Both also had strong neuroscience committees that included neurosurgeons and neurologists, as well as oncologists, radiation oncologists, neuroradiologists, neuropathologists, and psychologists.
As the COG has grown, so has its mission. Initially designed to perform clinical and research trials to define optimal treatments for children and adolescents with cancer, the COG also focuses on laboratory research that will translate into more effective treatments, on the late effects of the treatments and their impact on the quality of life of survivors, and on studies that identify the causes of childhood brain tumors.
The PBTC, formed in the mid- to late 1990s, was conceptualized by the National Cancer Institute and the National Institutes of Health as a consortium of institutions in the United States that would perform technologically challenging, translational trials for children with primary central nervous system tumors. The primary focus of the PBTC is on investigating the use of agents that would be difficult to initially evaluate on a larger national or international basis. This would include evaluating novel chemotherapeutic agents, biologic agents, drug combinations, and neurosurgical techniques. Given the initial charge of the PBTC, the majority of the studies that have been performed to date have been phase I studies, testing predominantly, but not exclusively, biologic agents. However, in selected disease types, especially brainstem gliomas and infantile brain tumors, larger phase II studies have been undertaken by the group. A major responsibility of the PBTC was to develop studies that then could be tested in larger cohorts of patients more efficiently through larger groups like the COG. At times, there is blurring of the missions of COG and PBTC, especially in decisions of what studies should move ahead to COG studies and what PBTC studies should be continued as PBTC phase II studies. In addition, within COG there is a competitively chosen phase I consortium that performs studies similar to those usually undertaken by the PBTC. The COG and PBTC consortiums have joint committees attempting to clarify any differences in opinion concerning the ultimate best means to answer important questions in pediatric neuro-oncology. The PBTC, however, has not focused on the late effects of childhood brain tumors or their treatments.
International Society of Paediatric Oncology
As stated previously, SIOP is both a professional society and a cooperative group that coordinates multi-institutional and, by design, multi-national studies. Because of this overall design, the coordination of the studies performed by groups under the SIOP umbrella is more flexible, as national cooperative groups can choose to participate in studies as they wish. This is primarily due to the lack of centralized funding and the need for countries to be essentially self-supporting in the performance of these studies. Most of the countries in Europe have well- organized cancer study groups that may or may not participate in SIOP studies and perform brain tumor investigations. Cooperative groups that have been especially prominent in the performance of clinical trials for children with brain tumors in collaboration with SIOP include the United Kingdom′s CCLG, the French Pediatric Oncology Group (Société Française d′Oncologie Pédiatrique [SFOP]), and the German Pediatric Oncology Group (Ge sell schaft für Päd ia tri sche On ko lo gie und Hä ma to lo gie [GPOH]). The German group was formerly a West German organization but has evolved into a study group accruing patients from all of Germany (including old East Germany) and Austria. The involvement of Italian groups within SIOP has been somewhat more fragmented, as regional geographic study groups are more prominent in Italy.
The exact mechanism of study development for SIOP varies depending on the tumor type being studied and the interests of individual investigators. SIOP does have a subcommittee whose primary responsibility is the development of clinical trials for children with central nervous system cancer. Researchers from each of the individual study groups involved in the development of the national studies bring new ideas and approaches to the meeting, and then a decision is made about who will participate and who will lead the clinical research effort. SIOP does not have a central resource laboratory accruing specimens from studies (however, some national groups have well-developed resource laboratories that fulfill this role), nor does it have a statistical office to coordinate all studies.
Children′s Cancer and Leukaemia Group
The CCLG, the U.K. cooperative trials group, was originally established in 1977 and combined its trials program with a national cancer registry (Children′s Cancer Research Group), thereby permitting trial recruitment to be expressed as a percentage of the national cancer registrations registry. The group′s funding came initially from cancer charities—Cancer Research Campaign, Cancer Research UK—and later the brain tumor program was supported by the Samantha Dickson Brain Tumour Trust. This charity funding was terminated in 2008, and the CCLG now has reorganized funding from government sources for the trials network and clinical studies groups as part of the National Institute of Health Services Research (NIHR)-funded National Cancer Research Network (NCRN).
Clinical Trial Structure
Study Types
Clinical trials, which are the major effort of the groups and are designed to identify more beneficial treatments, are typically are classified as phase I, II, or III. Each phase has a different primary objective. Cooperative groups often also develop and conduct nontherapeutic studies addressing other objectives, such as biological and epidemiological research. Phase I and II trials are used to screen new treatments for toxicity and feasibility (phase I) and effectiveness (phase II) before they are studied comparing different treatments in larger phase III trials. In practice, the classification of trials as phase I, II, or III is not so straightforward. A trial can address phase I and phase II or phase II and phase III objectives simultaneously. It is nevertheless worthwhile understanding the differences among phase I, II, and III trials ( Table 45.3 ).
Phase I Trials
Phase I trials are used to evaluate toxicity and feasibility of new treatments or treatment components in a small number of relapsed or refractory patients.3 Often these trials employ a statistical rule to determine a maximum tolerated dose (MTD). An important part of the design of these trials is the definition of dose-limiting toxicity (DLT). These can be one or a constellation of toxicities whose occurrence in a large proportion of patients would make the treatment unacceptable. In a standard design, several increasing dose levels are used, and patients are recruited in cohorts of three starting with the lowest dose level. Patient entry is suspended until the toxicity evaluation of all patients in the cohort is complete. If no DLTs occur, three patients are recruited at the next highest level; if one DLT occurs, three more are recruited to the current level; and if two or more DLTs occur, the dose level is rejected as too toxic. Six patients must be treated at the final dose level, and a level is acceptable only if no more than one DLT has occurred in the six patients. The MTD is defined as the highest dose level with no more than one DLT occurring in the six patients.
Although this is a reasonable rule in the first investigation of a treatment, only a small number of patients is involved, and hence the true toxicity of the selected MTD is variable. For example, with six dose levels with true DLT rates of 2%, 5%, 10%, 20%, 30%, 40%, respectively, dose level No. 4 is the most likely to be selected, with a DLT rate of 20%. It is almost as likely that level No. 3 or level No. 5 would also be selected, with DLT rates of 10% and 30%, respectively, and it is possible (7% chance) that level No. 6 will be selected, with a 40% DLT rate. Although the standard phase I design provides a crude screen for treatments that are excessively toxic, continued monitoring of toxicities in subsequent studies is essential.
Phase II Trials
The objective of phase II trial is to estimate the effectiveness of a new treatment as reflected in acutely measurable disease outcomes, such as tumor response rate. As in phase I trials, usually small numbers of relapsed or refractory patients are used; however, phase II trials also may be performed in newly diagnosed patients. In a modified Chang design currently used in some CCG studies, 10 patients initially are recruited.4 If no responses are observed, the treatment is called ineffective, and if six are observed, the treatment is called effective enough for further study. If one to five responses are observed, 10 more patients are recruited. In these 20 patients, at least four responses are required to accept the treatment for further study. With this rule, ineffective treatments will be rejected, and moderately effective treatments (response rate of 20 to 30%) will be accepted, with high probability. As for phase I trials, the results of a single phase II trial can be variable. A treatment giving eight responses in 20 patients will have an estimated response rate of 40% ± 11%, or a 95% confidence interval of roughly 18% to 61%. Hence, the data suggest only that the treatment is not totally ineffective, but does not give a precise idea of the true efficacy as measured by response rate, nor guarantee a high response rate.
For the Phase II design above, treatments with a low target response level (20 to 30%) in selected groups of patients with resistant disease are potentially valuable additions to modern multiagent, multimodality treatment for newly diagnosed patients. On the other hand, a treatment with response rates of 20 to 30% in previously untreated tumors would not be expected to affect a large improvement in outcome in diseases that have been shown to respond to other therapies. Thus, it is important to consider the context in which a particular statistical rule is being applied. The exception would be for disease in which no treatment has yet proved effective.
Phase III Trials
Phase III trials compare two or more treatments in sufficient numbers of patients to estimate with precision differences in treatment effectiveness.5 For the purposes of this discussion, phase III trials will include historical control trials, concurrent nonrandomized control trials, and randomized control trials, although typically only the last type is considered a true phase III trial.
Historical control trials use as a control group a cohort of patients treated at a previous time, comparing them to a cohort of patients treated with a new treatment. In these trials, it is prudent to assume that there are inequalities in the two patient cohorts in important prognostic factors, resulting in bias in the comparison. One tries to control for this by a posteriori adjustment during statistical analysis, but it may be impossible to adjust adequately for these imbalances. Because the old and new cohorts were treated at different times, there can be unquantified differences between the care received, the histological or stage definition of disease, and the quality of the data, which make unbiased comparison between treatments impossible. A good example is the study of medulloblastoma in the Children′s Cancer Group. If one were to mount an historically controlled trial in high-risk (metastatic or > 1.5-cc residual disease) medulloblastoma, there are one of two possible cohort of patients that could be identified: study CCG-942, performed in the late 1970s and early 1980s; and study CCG-921, performed in the late 1980s and early 1990s.6,7 Both studies provided data about two important prognostic factors—degree of dissemination (M-stage) and extent of tumor resection—that can be used to define the control group. In fact the meaning of these data has changed with time. During CCG-942, computed tomography (CT) was not universally available, so that staging was based primarily on surgical impression, cerebrospinal fluid (CSF) analysis, and myelogram. Because this trial was open to all medulloblastoma patients, CSF cytology and myelogram were not strict eligibility criteria, and hence were not performed in a proportion of patients classified as M0 on data flow sheets. During CCG-921, CT scan was available but magnetic resonance imaging (MRI) was not universally available. Today′s minimally acceptable staging includes brain and spine MRI and lumbar CSF for these patients. Further, given the dramatic improvements in neurosurgery and imaging since the late 1970s, it is clear that patients with a total resection in 1978 must include those who today would be considered incompletely resected, and that patients with an incomplete resection today may have on average smaller residual tumor volume than similarly classified patients in 1978. There usually is no way to correct for these real biases in analysis, because these qualitative differences in staging and treatment were never captured as part of the original study data. Conclusions drawn from historically controlled treatment comparisons may not be convincing for these reasons, unless the observed differences in efficacy are far larger than could be attributed to bias.
The second type of phase III trial is a concurrently controlled nonrandomized trial. Here the treatment is selected by the patient and physician. Although the time biases discussed above are not an issue, there will still be unquantified differences between the treatment groups. Information that only the treating physician sees and appreciates, such as poor performance status or degrees of disease spread not reflected in the collected data, will affect outcome and can also influence which treatment is used. Because these differences are unknown to the study investigators and therefore not available at the time of analysis, concurrent control nonrandomized studies share many of the problems of interpretability as historically controlled trials.
The last type of phase III trial, the randomized concurrent control trial, has become an accepted standard for comparing treatments, because the problems noted above with historical or nonrandomized uncontrolled trials are largely avoided. Treatments are assigned randomly to patients who are willing and able to accept either experimental or control treatments. It is assumed at the start of a randomized trial that there is no convincing scientific evidence that one treatment is superior to the other. Such information will accumulate during the trial, and thus all randomized clinical trials should be designed with interim analysis rules to stop the trial when convincing evidence of a difference between treatments exists, or for that matter when convincing evidence that there will be no difference exists.8 Because of the random assignment, treatment groups will most likely be comparable with respect to unquantified prognostic factors. The randomization does not guarantee comparability, but the chance of important differences between groups is known and is expressed as the p value in the reports of these comparisons. For example, when a difference in outcome between groups is reported with p < 0.05, this means that, if in fact the two treatments are equally effective, it is unlikely (< 5% chance) that the difference would have been observed; p < 0.05 means there is no guarantee that the observed difference is due to the treatment and not due to chance. However, if one uses this criterion in judging evidence in all trials, only 5% of trials involving equally effective treatments will show erroneously significant results.
It is important in phase III trials also to consider the sample size of the trial. Large trials are necessary, if they can be performed in a reasonable time, because this ensures that if there is a difference in treatment effectiveness, the trial will likely provide evidence of it at the level of p < 0.05. Small trials are unconvincing when they yield negative results, because moderate but important improvements in treatment effectiveness are not likely to be significant.
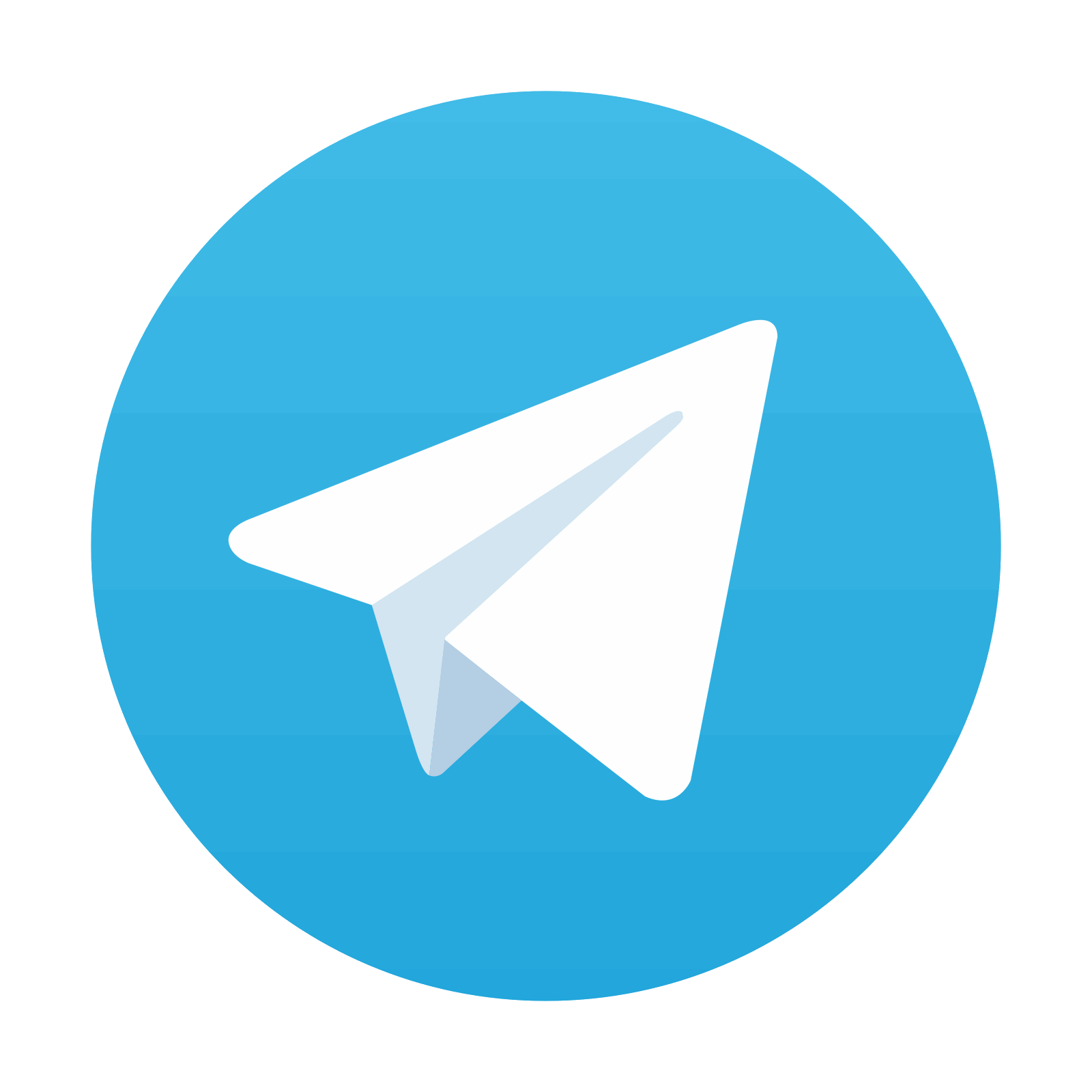
Stay updated, free articles. Join our Telegram channel

Full access? Get Clinical Tree
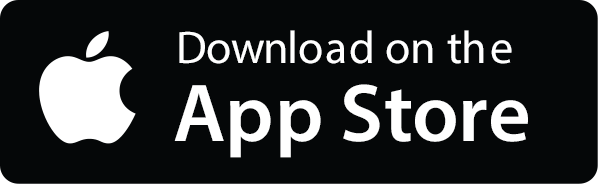
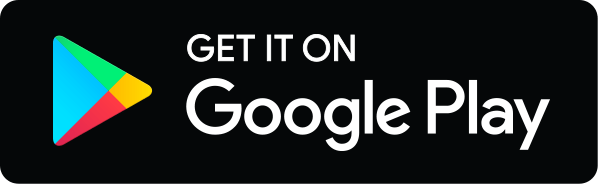
