Figure 88.1. Sagittal T1 magnetic resonance image showing an anterior two-thirds callosotomy.
Figure 88.2. Coronal T2 magnetic resonance image showing the position of the craniotomy and the division of the genu into the midline cavum.
Figure 88.3. Coronal T2 magnetic resonance image showing division of the posterior body of the corpus callosum. Note the position of the fornices below the corpus callosum.
Once an unencumbered view of the intrahemispheric fissure is obtained, the medial aspect of the exposed frontal lobe is covered with moist cottonoids, and self-retaining retractors are gently advanced. The falx is followed down the midline until the cingulate gyri are encountered. An error that is sometimes made is to mistake this view of the adherent cingulate gyri for the corpus callosum. The cingulated gyri are separated under magnification in the midline, exposing the corpus callosum and the pericallosal arteries. Once this view is obtained and the retractors are set, a final check of the anterior exposure confirms the exposure of the anterior corpus callosum if the genu is visible.
The actual division of the anterior corpus callosum is done with a microdissection instrument and gentle suction. This should begin in the midline of the callosum just posterior to the genu. Great care is taken to separate, but not disturb, the pericallosal arteries. At this level, certain landmarks, such as the cavum of the septum pellucidum, are visible beneath the corpus callosum, even if it is only a potential space in the individual patient. This midline landmark is valuable, if found, because it confirms the complete transection of the callosal fibers and it allows one to stay out of the lateral ventricles. If the lateral ventricle is entered, intraoperative or postoperative bleeding may cause hydrocephalus. The transection is then carried forward into the genu and the rostrum of the corpus callosum. The disconnection is carried out downward following the A2 branches as they approach the anterior communicating artery complex. The extent of posterior callosal sectioning is decided preoperatively. Some surgeons advise a simple one-half callosal sectioning, which can be measured by comparing the intraoperative transection to the length of the callosum on the preoperative sagittal MRI. This and other techniques, such as intraoperative plain films and stereotaxy, have been described to confirm the length of callosotomy (47). Other authors advocate a three-quarter sectioning, as there is some indication that seizure control may be more complete. Jalilian et al. (39) reported that an upfront complete corpus callosotomy should be considered in children with lower functional status. Bower et al. (48) reported that the majority of patients in their series that underwent an anterior two-thirds callosotomy derived significant benefit without requiring a completion of the callosotomy.
If a complete corpus callosotomy is to be performed, the sectioning may be done with a microdissector or suction aspiration to the splenium. A complete posterior sectioning is confirmed by viewing the arachnoid covered vein of Galen in the posterior midline. If only an anterior transection is planned, an MRI-compatible marker should be placed at the posterior border of the anterior transection in order to see it on imaging studies and to note on reoperation, if necessary, the extent of the first procedure. Hemostasis is obtained, and any entry into a lateral ventricle is covered with Gelfoam. A standard craniotomy closure is performed.
Over the past few years, there have been increasing reports in the use of radiosurgery or gamma knife to perform a corpus callosotomy (49–51). While the numbers in each series were small, efficacy was comparable to traditional surgical callosotomy (49–51). Moreno-Jimenez et al. (52) reported that DTI acquired after callosal radiosurgery showed progressive decrease in fractional anisotropy compared to controls. Falowski and Byrne (53) reported in 2012 CO2 laser suction device versus bipolar electrocautery to improve midline dissection and decrease the likelihood of entering the ventricles.
Complications
Complications unique to corpus callosotomy as a surgical procedure are often neuropsychological in nature. Well-described acute and chronic neuropsychological sequelae are possible after callosotomy (54,55). Varying degrees of acute disconnection syndrome are commonly seen. This syndrome is characterized by a lethargic, apathetic mutism during the first few days after surgery. For most, this is always transient. The predictors of this transient state are related to the extent of callosal sectioning, baseline cognitive impairments, and the amount of traction necessary to gain access to the corpus callosum. Other early complications of the acute syndrome are incontinence, bilateral Babinski sign, and apraxia.
Chronic disconnection syndrome was initially not well recognized when callosotomy was first described (1). Detailed neuropsychological testing reveals deficits that are common after callosotomy but are not usually clinically significant. The majority of neuropsychological alterations, other than mutism, occur with posterior callosotomy. This is caused by disruption of communication between visual and tactile cortical sensory functions and verbal expression. Because of the disconnection between the hemispheres, an object placed only in the left visual field of a left hemisphere–dominant patient will be seen by the right hemisphere, but the information will not be transferred to the left hemisphere for speech production. Thus, the patient recognizes the object but cannot name it. Similarly, an object placed in the left hand, but not seen, may be recognized by its shape and size, but it will not be named. This is interesting but not clinically disabling to the patient because objects are normally seen by both hemispheres and can be felt with either hand. If a patient has bilateral speech representation, dysphasia may be a postoperative complication. This should be considered before complete callosotomy is performed on a patient with mixed speech dominance.
A disturbing complication known as alien hand syndrome has been reported (55). In this syndrome, poor cooperation or even antagonistic behavior between the left and right hand is noted. The verbal-dominant hemisphere may express displeasure with the actions of the ipsilateral extremities. This phenomenon is usually short lived and is usually seen only in the immediate postoperative period; however, on rare occasions, it may persist. Performing only an anterior callosotomy can minimize the likelihood and the extent of these neuropsychological sequelae. If the anterior callosotomy is unsuccessful in controlling seizures, completion of the callosotomy may be performed at a later time.
Other observed complications are related to frontal lobe retraction: cingulate gyrus injury, injury to the pericallosal arteries, bridging veins or superior sagittal sinus, and hydrocephalus following entry into the lateral ventricle. Postoperative hydrocephalus secondary to entry into the ventricular system and a subsequent ventriculitis has been dramatically reduced by using an operative microscope and carefully respecting ventricle boundaries. Transient mutism may be reduced by minimizing the retraction of frontal cortex and retracting the nondominant frontal lobe, if possible. Despite this, mutism may occur transiently in up to 30% of patients.
Spencer et al. (7) reported a meta-analysis of long-term neurologic sequelae of both anterior and complete corpus callosotomy. They found that motor sequelae were reported in 56% of complete and 8% of anterior callosotomy patients; language impairments in 14% and 8%, respectively; and both cognitive impairment and behavioral impairment in 11% and 8%, respectively.
Some authors have suggested certain contraindications to corpus callosotomy. Spencer et al. (7,15) found that patients with severe mental retardation (IQ lower than 45) did not derive any benefit from the procedure. Other studies, however, have not found any relationship between IQ and outcome (32,33,56–58). A relative contraindication has been proposed concerning patients whose hemisphere of language dominance is not that of hand dominance (59). Speech difficulties, with sparing of writing, have been identified in patients who are right hemisphere dominant for speech and are right-handed, and dysgraphia with intact speech has been identified in left-handed patients with a dominant left hemisphere.
In conclusion, corpus callosotomy is an effective surgical technique for the treatment of selected pharmacoresistant epileptic syndromes, particularly certain types of seizure (i.e., atonic seizures). Over the past 10 years, its use has decreased as a result of the introduction of new antiepileptic drugs, especially lamotrigine and topiramate, and a rekindling of interest in the ketogenic diet. The vagus nerve stimulator has clear benefit for atonic/tonic seizures, and cortical stimulation may be beneficial for “drop” seizures, but no conclusive data are yet available. Certain epilepsy centers in the United States are routinely performing VNS before considering corpus callosotomy. In general, anterior corpus callosotomy is still an underutilized procedure, especially for patients with intractable atonic seizures associated with recurrent falls and subsequent head injury. Radiosurgery has been proposed as an alternative to surgical callosotomy by Pendl et al. (60). Although this is a promising approach, several questions about volume–dose analysis and long-term efficacy are yet to be fully answered (61,62).
MULTIPLE SUBPIAL TRANSECTION
Focal-onset medically intractable epilepsy has been surgically treated for 70 years by location of the seizure focus and resection of the involved cortex. A certain proportion of patients who undergo evaluation for possible surgical resection are found to have an epileptogenic zone originating in, or overlapping with, eloquent cortex. These patients traditionally have been denied surgery because resection of primary speech, motor, sensory, or visual cortex would result in unacceptable deficits. MST was developed specifically to address this problem. The purpose of this technique is to disrupt the intracortical horizontal fiber system while preserving the columnar organization of the cortex (i.e., its vertically oriented input and output systems and vascular supply) (63). The transection of horizontal fibers is aimed at preventing the propagation of epileptic discharges, thus averting the synchronous neuronal activation that ultimately results in the development of clinical seizures. The preservation of the columnar organization of the cortex prevents or minimizes the disruption of the functional state of the transected cortex.
The development of this technique was derived from three sets of experiments, each unrelated to the others or to the field of epilepsy surgery. The first set of experiments by Asanuma and Sakata (64), Hubel and Wiesel (65), and Mountcastle (66) demonstrated that the vertically oriented micro- and macrocolumns (with their vertically oriented input, output, and vascular supply) are the organizational unit of functional cortical architecture. The functional role of the intracortical horizontal fiber system is yet to be firmly established. However, this system is composed of fibers responsible for recurrent inhibition and excitation underlying neuronal plasticity. In the second set of experiments, Sperry (67) demonstrated that surgical disruption of the horizontal fiber system in the visual cortex of the cat, while sparing its columnar organization, does not affect its testable functional status. Tharp (68) postulated to the importance of the horizontal fiber system as a “critical component in cortical circuit necessary for generation and elaboration of paroxysmal discharges.” Epileptic activity in the form of spikes or sharp waves requires a synchronous neuronal activation of a contiguous cortical surface of at least 12 to 25 mm2 (68,69). Tharp found that epileptic foci would synchronize their activity if the distance between them was 5 mm or less, and disrupting the neuropil between the foci would desynchronize the epileptic activity.
With this information, Morrell et al. (63,70) hypothesized that sectioning of the intracortical horizontal fibers at 5-mm intervals, while preserving the columnar organization of the cortex, could abolish epileptic activity yet preserve the functional status of the transected cortex. Testing this hypothesis in the monkey, Morrell produced an epileptic focus with aluminum gel lesions in the left precentral motor cortex, which resulted in the development of focal motor seizures. Using a small wire, he disconnected the horizontal fibers at 5-mm intervals throughout the epileptogenic zone. This procedure, the first subpial transection for epilepsy, stopped the seizures, and the monkey suffered no motor deficits from the procedure. To confirm that what he had transected was motor cortex, 1 year later, Morrell surgically removed the transected area, resulting in the expected hemiparesis. With this experimental evidence, Morrell et al. moved forward into the treatment of intractable human neocortical epilepsy arising in or overlapping eloquent cortex.
Indications for Multiple Subpial Transection
MST is indicated in any patient in whom the epileptic zone arises from or overlaps with eloquent cortex. The procedure is performed after a detailed presurgical evaluation, which includes closed-circuit television/electroencephalographic recording of habitual seizures using scalp and intracranial electrodes, mainly subdural grids. In addition, detailed functional mapping to identify eloquent cortex by electrical cortical stimulation and evoked potentials is performed. Neuropsychological testing and intracarotid amobarbital tests, as well as functional neuroimaging studies, all assist in defining the baseline function and risks of the procedure. Magnetoencephalography studies have also been very useful in the evaluation of children with an acquired epileptic aphasia of childhood or Landau–Kleffner syndrome (LKS) (71). It allows more accurate identification of the source of the dipole, especially its depth within a sulcus.
MST can be performed as the sole procedure or in conjunction with resection of noneloquent cortex, depending on the extent to which the epileptogenic zone involves eloquent cortex. Most cases of MST occur in conjunction with a cortical resection. Candidates are typically patients with dominant temporal neocortical epilepsy, dominant frontal lobe epilepsy, or primary sensory, motor, or visual cortex involvement. In patients undergoing resection/transection, resection of noneloquent cortex is performed to within 1.5 cm of the identified eloquent cortex. We recognize that this patient group is problematic for the evaluation of the clinical effectiveness of MST.
Cortical Surgical Anatomy
Human cortex is arranged in a gyral pattern, which is fairly constant between individuals. However, the microgyral patterns of individual gyri may considerably vary. These cortical variations must be taken into account in a procedure where transections are being made perpendicular to the long axis of a gyrus. Thus, careful inspection of each gyrus prior to the procedure is important. Gray matter is, on average, 5-mm thick over the crown of a gyrus. However, the depth of each sulcus differs by individual.
These points are critical in subpial transection procedures because the objective is to divide the neuropil into 5-mm intervals perpendicular to the long axis of the gyrus while preserving the overlying pia with its blood vessels and the underlying white matter tracts and U fibers.
Approximately a quarter of our patients have undergone MST as their primary procedure. These patients mainly had epilepsia partialis continua due to Rasmussen encephalitis or LKS. In the patients with Rasmussen syndrome, the epileptogenic zone arose from primary language and/or motor cortex, whereas in patients with LKS, it involved posterior language cortex.
Operative Procedure
Patients are given preoperative antibiotics and often steroids and are positioned so that the surgical site is at the highest point in the operative field. This makes intraoperative electrocorticography (ECoG), resection, and transection easier. The head is held in Mayfield head fixation, and all pressure points are padded. If the operation is done with the patient awake, the patient’s comfort is especially important.
Anesthesia is accomplished with intravenous methohexital and a generous amount of local anesthesia. Although methohexital has been shown to activate interictal epileptiform activity, such activation does not extend beyond the epileptogenic zone (72). Furthermore, the degree of activation of epileptiform activity can be minimized by lowering the infusion rate of methohexital. At our center, we perform intraoperative ECoG in all cases, even when mapping with subdural grids has been done, to ensure that the initial transections result in the desired abolition of epileptic activity.
Transections
Before performing the transections, careful inspection of the gyri, microgyral pattern, sulci, and vascular supply is carried out. Transections are first performed in the more dependent areas to avoid the problem of subarachnoid blood obscuring the other areas. At the edge of the visible gyrus, in an avascular area, a 20-gauge needle is used to open a hole in the pia. The tip of the subpial transection hook is introduced into the gray matter layer and advanced to the next sulcus in a direction perpendicular to the long axis of the gyrus. The tip of the hook is held upward and is visible immediately beneath the pia. It is important that the pia be left undisturbed to minimize vascular injury and scarring. The transection hook is designed with a handle, a malleable shaft, and a tip that is 4-mm long (paralleling the cortical width) and 1-mm wide. If the 4-mm tip is introduced just below the pia, it should remain in the gray matter layer, leaving the white matter undisturbed. The tip is angled at 105 degrees and is blunt. These two features make snagging or injuring a vessel less likely. However, it is important to avoid crossing a sulcus where buried vessels are unprotected. While this procedure is simple in principle, we have found that to master it requires considerable experience.
After the first transection is completed, bleeding from the pial opening is controlled with small pieces of Gelfoam and a cottonoid. The 4-mm tip is then placed up against the cortex next to the transection so as to select the next transection site 5 mm from the first. This is repeated until the identified epileptogenic zone is transected. Over a few minutes, the lines take on a striped appearance from the petechial hemorrhages along the lines. Minimal bleeding is encountered if the transections are done properly. ECoG is repeated at the conclusion of the transections. The transected area displays a significant attenuation of the background activity with elimination of the spikes. In cases of persistent epileptiform activity, the possibility that activity is coming from the depth of a sulcus or from remote areas must be considered. On rare occasions, when persistent activity is clearly identified as originating in an area that has been transected, transecting down into the sulcus may be done. In order to perform this safely, the tip of the probe should be turned away from the sulcus as the instrument is advanced.
Favorable outcomes using alternative instruments and methods of transection have been described by neurosurgeons (73,74). Ntsambi-Eba et al. (75) reported a large series utilizing radial MST from a single cortical entry point.
Seizure Outcome
Evaluation of seizure outcome should be carried out in patients who underwent MST without additional cortical resection. We have previously reported our series of patients with partial epilepsy with 37.5% of patients becoming seizure-free at 2-year follow-up with an additional 37.5% having a worthwhile outcome (class II–III). However, as has been reported by other centers, there is a late reoccurrence rate in seizures following MST (76). Orbach et al. (77) reported a relapse rate of 18.6% over several years. Schramm et al. reported on the efficacy of MST alone in 20 patients with drug-resistant epilepsy. One patient had a previous temporal resection; there were two cases each of LKS and electrical status epilepticus of sleep (ESES). In this series, 10% had a class I outcome and 45% had a class II–III outcome. They also noted the relapse in seizures over time (78). Zhao et al. reported on 80 patients treated with MST alone as part of his larger series. He reported 51.7% seizure freedom in patients with at least 1-year follow-up (79).
Ntsambi-Eba et al. reported on 62 patients, using radial MST. Twelve patients were MST alone. Reduction in seizures by 50% was seen in 79%. Forty-two became seizure free and 33% of the MST alone group (75).
In a meta-analysis of MST with or without additional cortical resection, Spencer reviewed 211 patients who underwent MST for intractable epilepsy and found an excellent outcome (>95% reduction in seizure frequency) in 87% of patients who had generalized seizures and 68% of patients with simple and complex partial seizures (80). Zhao et al., in the largest series reported to date, reported on 200 patients (80 MST alone) treated with MST for intractable partial epilepsy involving eloquent cortex between 1991 and 2000. They found complete control of seizures in 62.5% with another 20% of patients having a significant reduction (>75%), with 160 patients having at least 1-year follow-up (79).
Pondal-Sordo et al. published the neurosurgical experience of London, Ontario, with perirolandic epileptic foci with cortical resection with or without additional MST. The most common etiology was neoplastic. Average follow-up was 4.2 years. At follow-up, 46% had worthwhile outcome (Engel class I, 31%; Engel class II, 15%). Residual deficits were seen in 50% but were mild in half. Those patients whose postoperative ECoG showed infrequent or no epileptiform activity had better surgical outcomes (81).
In pediatric patients, Shimizu and Maehara (82) analyzed 25 cases where MST was utilized with 10 out of 25 having an Engel class I or II outcome. In 2006, Benifla et al. reviewed two studies of MST efficacy that included 60 patients (10 MST alone). They found that between 33% and 46% of patients in the respective series had Engel class I or II outcomes (83).
MST had been used to treat hippocampal epilepsy and preserve verbal memory. Shimizu and Maehara reported a case series of 21 patients who underwent multiple transections of the pyramidal layer of the hippocampus under the alveus using a modification of the MST procedure. Of the 21 patients, 17 were followed for more than 1 year. Fourteen patients (82%) became seizure-free and two (12%) had rare seizures. Eight patients underwent a full postoperative battery of neuropsychological testing of verbal memory. Verbal memory was completely spared in seven, with one patient having a transient worsening that cleared over 6 months (84). The authors were encouraged with the above results; however, a longer follow-up and greater numbers of patients are required before transection of hippocampus is confirmed to be efficacious and sparing of verbal memory function.
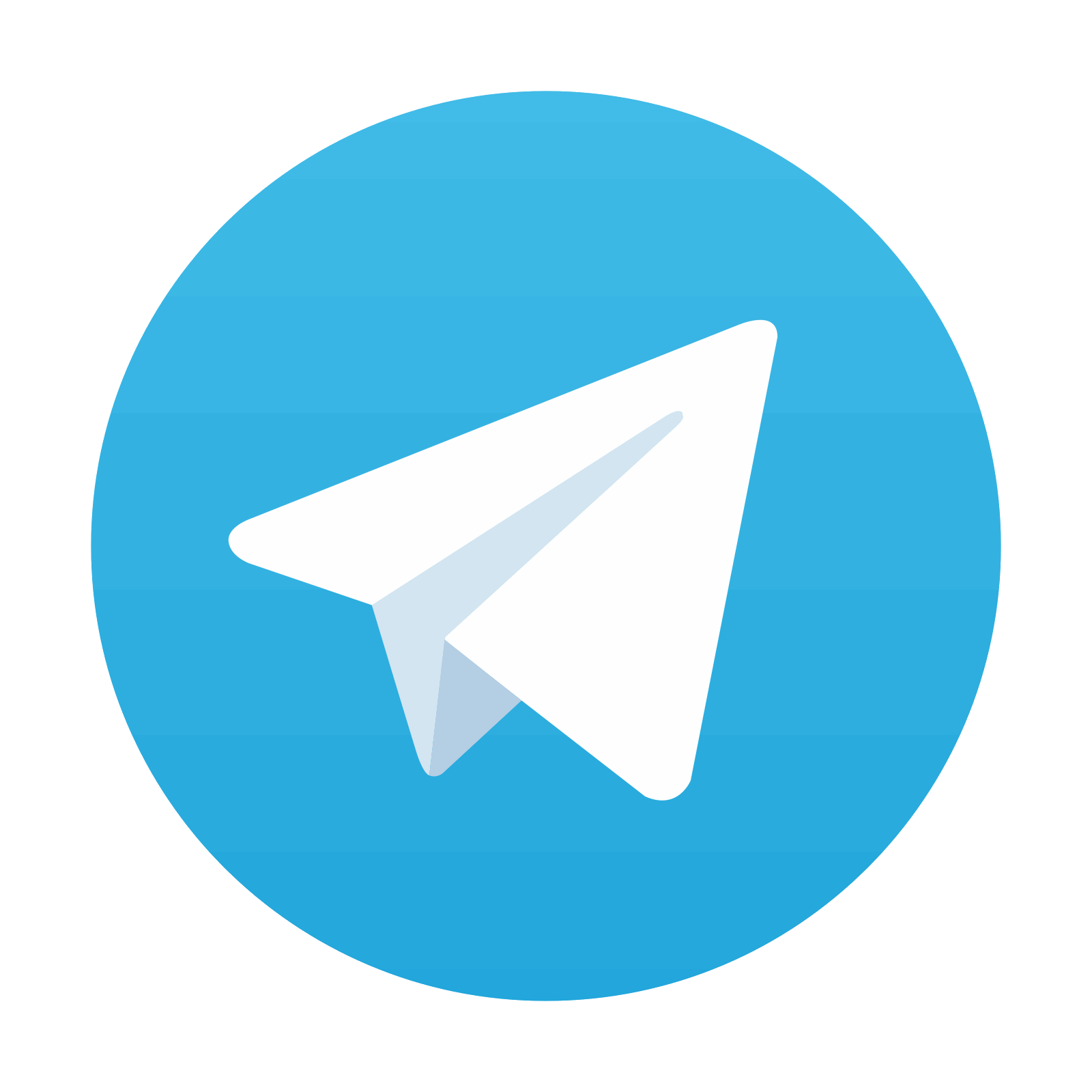
Stay updated, free articles. Join our Telegram channel

Full access? Get Clinical Tree
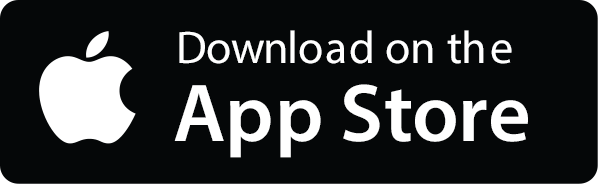
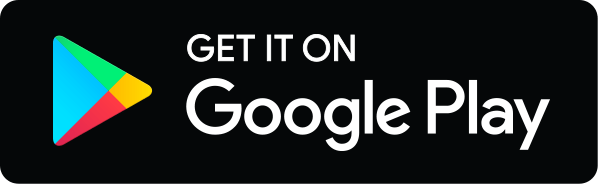