Cranial Arteriovenous Malformation Embolization
Pearls
Cranial arteriovenous malformations (AVMs) are a heterogeneous group of intracranial vascular lesions that require a multidisciplinary team that includes neurosurgical, endovascular, and radiosurgical expertise to optimize the risk-to-benefit ratio of treatment.
The function of endovascular therapy in AVM treatment can be divided into the following categories: presurgical embolization, preradiosurgical embolization, targeted embolization (i.e., securing an associated aneurysm), curative embolization, and palliative embolization.
Superselective angiographic imaging must be reviewed diligently to identify the following characteristics of the AVM angioarchitecture: (1) en passage feeders into normal neural tissue from the pedicle, (2) the first appearance of venous drainage, (3) potential proximal vessels that may be compromised by reflux, and (4) the rate of contrast opacification through the nidus.
The advantage of Onyx over n-butyl cyanoacrylate (nBCA) is that the injection can be stopped periodically to evaluate its progression, the patency of the venous drainage, and the remaining nidal angioarchitecture.
The “plug-and-push” technique can cast additional new areas of nidus, and several milliliters of Onyx are often used for a single pedicle injection. However, the procedure is stopped when adequate Onyx casting is achieved or if retrograde reflux threatens to occlude proximal en passage branches.
Brain arteriovenous malformations (AVMs) are highly complex vascular lesions that account for approximately 2% of all hemorrhagic strokes.1 Although they are relatively rare, AVMs can cause significant long-term morbidity and mortality because most patients are healthy and young. The angioarchitecture of AVMs consists of arterial feeders directly connected to the venous system without an intervening capillary bed, creating high-flow arteriovenous (AV) shunts (Fig. 37.1). AVMs are considered congenital pathologies that occur sporadically, but they can be associated with several hereditary syndromes, such as Rendu-Osler-Weber syndrome and Wyburn-Mason syndrome, and with Sturge-Weber disease. Reports of familial cases are extremely rare.2
Most AVMs are discovered after patients experience an intracranial hemorrhage. Other presentations include headaches, seizures, or a focal neurologic deficit. The natural history of these lesions is poorly understood because most studies are retrospective and biased (i.e., relatively uncommon and heterogeneous lesions are included, and there is a selection bias toward untreatable AVMs). Many investigators estimate an annual risk of hemorrhage for an AVM to be 2 to 4%, with an increased risk of hemorrhage in the first few years after a ruptured presentation of 6 to 18%.3 , 4 The risk then gradually returns to baseline.
The treatment paradigms for cerebral AVMs have evolved into a multidisciplinary strategy with the goal of optimizing the risk-to-benefit ratio after a thorough evaluation of the angioarchitecture of the lesion. The current armamentarium includes microvascular neurosurgery, radiosurgery, and endovascular embolization. Based on prospective studies, the Spetzler and Martin grading system (Table 37.1) reasonably predicts the surgical morbidity and mortality rates associated with cerebral AVMs.5 Multiple investigators have reported low rates of surgical morbidity and mortality for grade I through III AVMs. The risk of hemorrhage associated with grade I and II AVMs typically outweighs that of microsurgical resection. Smaller AVMs are more prone to rupture than larger ones. The inverse relationship between AVM size and feeding artery pressure suggests that smaller AVMs have a significantly higher rate of hemorrhage. Usually, the risk associated with preoperative embolization of grade I AVMs may exceed the surgical risk. Grade II AVMs in highly eloquent regions may be treated with stereotactic radiosurgery rather than surgical resection.

Grade III AVMs are the most complex and heterogeneous group. Lawton6 subcategorized grade III lesions into four types based on size (S), venous drainage (V), and eloquent location (E), and determined the relative risks of surgical morbidity and mortality. The surgical risk for small AVMs (S1, V1, E1), medium and deep AVMs (S2, V1, E0), and medium/eloquent AVMs (S2, V0, E1) was 2.9%, 7.1%, and 14.8%, respectively. Presurgical embolization is often required to occlude deep arterial feeders that can be difficult to visualize during microsurgery, thus minimizing intraoperative blood loss. Furthermore, embolized arterial vessels can be used as surgical landmarks.
The surgical excision of grade IV and V AVMs is associated with a high rate of major and minor perioperative deficits, which reach 31% and 50%, respectively. Han et al7 examined the outcomes of 73 consecutive patients with grade IV and V AVMs and found that the risk of hemorrhage (1% per year) in these two subgroups was lower than the risk of surgical complications. They recommended observing these lesions rather than intervening. Other publications have reported that partial treatment may increase the risk of future hemorrhage.7 , 8 Current recommendations reserve treatment of these high-grade AVMs for patients with progressive or disabling neurologic symptoms such as repeated hemorrhage, intractable seizures, venous hypertension, or vascular steal syndromes (Fig. 37.2). These data suggest the need for judicious selection of patients for the appropriate treatment algorithm to maximize the risk-to-benefit ratio.
♦ Endovascular Therapies
The treatment goals for cerebral AVMs dictate the role of endovascular interventions. The function of endovascular therapy in AVM treatment can be divided into the following categories: presurgical embolization, preradiosugical embolization, curative embolization, targeted embolization (i.e., securing an associated aneurysm), and palliative embolization. We discuss the first two categories in the following subsections.
Presurgical Embolization
At many medical centers, microvascular surgery remains the treatment of choice for cerebral AVMs. Preoperative embolization often has little or no role in the therapeutic management of superficial grade I or II cerebral AVMs because the additional risks of embolization may be unwarranted. An exception is the presence of deep arterial feeders that are surgically inaccessible. Grade III or higher cerebral AVMs are often embolized before microsurgical resection (Figs. 37.3 and 37.4). By occluding deep or surgically inaccessible arterial feeders, embolization can improve surgical outcomes. Surgery for a cerebral AVM with deep or surgically inaccessible feeders, which was once considered perilous, can be made a safer operation with preoperative embolization. Furthermore, embolization can decrease the size of the nidus and the amount of blood flow, which minimizes blood loss and decreases operative time. Embolized vessels can be identified easily during surgery, thereby helping to delineate a surgical plane and preventing resection of en passage vessels to adjacent eloquent cortex. Grade IV and V AVMs considered for surgical resection often require staged embolization because of their size and the additional risk of normal perfusion pressure breakthrough (NPPB) from the hemodynamic changes that occur within the lesion. Embolization of high-risk angioarchitectural features, such as the feeding vessel and intranidal aneurysms, may stabilize the nidus before surgery and decrease the risk of intraoperative hemorrhage.9




Preradiosurgical Embolization
Stereotactic radiosurgery is a common therapeutic option for AVMs that are not appropriate for surgical resection, such as those located in eloquent or deep-seated regions. The radiosurgical obliteration response of an AVM is highly dependent on dose and volume. Small cerebral AVMs that are less than 3 cm in diameter can be cured in 3 years with radiosurgery, with a documented rate of success in the range of 70 to 95% after a single session.10 , 11 One role of endovascular embolization is to shrink a large AVM to a smaller single target that is amenable to stereotactic radiosurgery. This strategy allows the delivery of a higher dose of radiation, thus minimizing the side effects of radiation to surrounding tissues and improving the rate of radiosurgical cure. In some cases, high-dose radiation is not possible. Staged volume radiosurgery can be an option when two or more distinct regions of residual nidus are present after embolization.12 Other high-risk AVM features such as intranidal or feeding vessel aneurysms can be embolized before radiotherapy (Fig. 37.5). High-flow arteriovenous (AV) fistulas are often refractory to radiosurgery and are treated by endovascular embolization. Persistent residual AVMs after radiosurgery are also potential lesions that can be embolized or removed surgically, depending on their angioarchitecture.13 , 14

♦ Technical Nuances
Whether patients should undergo general anesthesia for AVM embolization has been debated extensively. Some argue that provocative testing with a short-acting barbiturate (amobarbital) while the patient undergoes a continuous neurologic examination helps avoid ischemic complications.15 Proponents of general anesthesia suggest that complete suppression of patient motion during embolization16 is a safer option and eliminates the need for the continuous neurologic evaluation. This group argues that thorough examination of the angioarchitecture and microcatheter position may be more reliable for avoiding ischemic complications than provocative testing.
In our practice, all patients undergo general anesthesia with neurophysiologic monitoring (both somatosensory evoked potentials and electroencephalography). A 6-French (F) sheath is placed into the common femoral artery, regardless of the agent used. A baseline activated coagulation time (ACT) is obtained, and heparin is administered to patients to achieve a targeted ACT of 200 to 250 seconds throughout the procedure. We believe that the risk of rebleeding with periprocedural anticoagulation therapy is low compared with the risks of embolic complications. A 6F guide catheter, either an Envoy (Codman, Miami Lakes, FL) or a Neuron (Penumbra, Alameda, CA) depending on the tortuosity of the vessel, is placed in the distal primary targeted vessel.
Arterial pedicles are identified on initial angiographic images. Flow-directed microcatheters are often used based on their preferential selectivity toward the vessel with the higher flow, which is usually a feeder into the AVM. The microwire is usually confined within the microcatheter to provide proximal structural support. Occasionally, the microwire is needed to redirect the microcatheter if it repeatedly flows toward a nontargeted vessel or if it must be maneuvered through tortuous feeding vessels. When the microcatheter has reached its pre-nidal or perianeurysmal position, it is confirmed with a gentle contrast injection on a blank roadmap. If the pedicle is a potential vessel for embolization, a superselective angiogram is obtained.
In the presence of high-flow AV shunting, adaptive changes occur in vessels in adjacent neural tissues. As the pedicles supplying the AVM become more exclusively dedicated to the lesion, collateral pathways are opened to supply blood to the surrounding parenchyma. Over an extended period, these collateral vessels can undergo “angiomatous change” that results in markedly dilated and abnormal vessels in the immediate vicinity of the AVM.17 When significant AV shunting is present, high frame rates (5 to 6/sec) may improve delineation of the angioarchitecture.
Superselective angiographic imaging must be reviewed diligently to identify the following characteristics: (1) en passage feeders into normal neural tissue from the pedicle, (2) the first appearance of venous drainage, (3) potential proximal vessels that may be compromised by reflux, and (4) the rate of contrast opacification through the nidus.
Typically, pedicles that contain arterial feeders to normal parenchyma are not embolized. To protect normal parenchyma, coils can be deployed distally in branches that supply the nidus over a long segment and then continue to normal tissue. The branches can be occluded proximally with liquid embolysates. This approach requires the presence of retrograde flow within the distal aspect of the branch, presumably from leptomeningeal contribution, to the normal neural tissue.
The position of the microcatheter in relation to the orientation of the pedicle and nidus is a crucial factor for success. The microcatheter should not overlap with either the nidus or draining veins in at least one image intensifier. This configuration allows early visualization of embolysate reflux to minimize the risk of gluing the microcatheter with n-butyl cyanoacrylate (nBCA) or occluding proximal feeders into normal tissues.
Contrast transit time through a nidus provides an estimate of the rate of AV shunting. This information translates into formulating the optimal composition of the nBCA-to-Ethiodol mixtures and initial injection rate. The transit time also determines which Onyx (ev3, Plymouth, MN) concentration should be used. Typically, Onyx-18 (6%) is used for most embolizations, whereas Onyx-34 (8%) is used for the high-flow fistulous portions of AVMs.
Before infusing liquid embolics, interventionists must be aware of the location of the venous drainage. The venous structures should be clearly delineated on both image intensifiers to identify the first sign of embolysates passing through the nidus and approaching the draining veins. It is helpful to use the angiographic image that demonstrates the pedicle and the first visualization of venous drainage on an in-suite monitor as a reference image to guide the injection periodically. It also can be helpful to outline the nidus and draining vein of complicated angioarchitecture during infusion.
Infusion of nBCA requires two experienced operators working in tandem. Before the nBCA is infused, it is appropriate for the neuroanesthesiologist to readminister paralytics if necessary and to reduce the mean arterial pressure by 20 to 30% to slow the AV shunting. The microcatheter is cleared with 5% dextrose in water to flush all ionic catalysts from the lumen. In a wedged catheter position without arterial contamination, the forward flow is controlled by the rate of injection. This procedure allows a slow, more controlled injection of a dilute nBCA-to-Ethiodol mixture.

Under continuous subtracted fluoroscopic roadmap, the nBCA mixture is then injected slowly into the nidus to obtain a solid cast. The injection is paused for several seconds if a drop of nBCA is observed in the draining vein. The infusion is continued if additional casting of the nidus is noted.
The procedure is terminated when one of three observations is noted: (1) the desired nidal filling is achieved, (2) proximal reflux occurs, or (3) the nBCA penetrates into the draining vein. The microcatheter is gently aspirated and briskly pulled from the patient. A postembolization angiogram is obtained to evaluate the status of the nidus and remaining arterial feeders, the patency of draining veins, and the presence of any complications (e.g., parent vessel injuries, thromboembolic events, extravasation).
In a nonwedged position, a lower concentration of nBCA-to-Ethiodol mixture is used because it flows more rapidly. The injection rate is faster, and the total period of injection is shorter compared with when the microcatheter is in a wedge position. In the setting of extremely high-flow fistulas, coil deployment is used as a strategy to impede the flow rate and to provide a structural lattice for nBCA adherence. A higher concentration of nBCA mixture is also infused to achieve the desired polymerization and casting within the fistulas.
The technique of infusing Onyx is completely different from that of injecting nBCA. Before it is used, the Onyx solution must be shaken vigorously for 20 minutes to fully suspend the tantalum powder. Otherwise, sedimentation of the tantalum causes inadequate opacification during infusion. Once the microcatheter is in satisfactory position as described previously, the dead space of the microcatheter is slowly purged with dimethylsulfoxide (DMSO, 0.25 mL/90 s). The Onyx is drawn into a DMSO-compatible 1-mL syringe and connected to the microcatheter. Next, the Onyx is injected slowly over 120 seconds to displace the DMSO, and subtracted fluoroscopic roadmap is initiated just before the injection is completed. The slow, steady injection should be approximately 0.1 mL/min and usually should not exceed 0.25 mL/min to avoid the angiotoxicity of the solvent.
The advantage of Onyx over nBCA is that the injection can be stopped periodically to evaluate its progression, the patency of the venous drainage, and the remaining nidal angioarchitecture. When reflux occurs proximally, the infusion is stopped for as long as 2 minutes to allow the Onyx to solidify around the catheter. This strategy allows a plug to form at the catheter tip, thereby increasing the probability of antegrade flow. Each time, a subtracted fluoroscopic roadmap is refreshed to prevent confusion about the progression of the Onyx. This process may need to be repeated multiple times. The goal is to establish forward flow into the AVM nidus.
If there is antegrade infusion into the draining veins, the infusion is also halted to allow the Onyx to solidify to prevent venous occlusion. This “plug-and-push” technique can cast additional new areas of nidus, and several milliliters of Onyx are often used for a single pedicle injection. However, the procedure is stopped when adequate Onyx casting is achieved or if retrograde reflux threatens to occlude proximal en passage branches.
Retrieving the microcatheter after Onyx injection requires patience, gentle aspiration, and constant tension. Deflection of the Onyx cast is common, and gentle traction should be maintained as tension is slowly increased over several minutes. If the microcatheter cannot be removed, it is cut at the groin sheath. Breakaway tip microcatheters are in the research and development phase and currently in use outside the United States. In the future, their use should facilitate removal and help avoid potential complications.
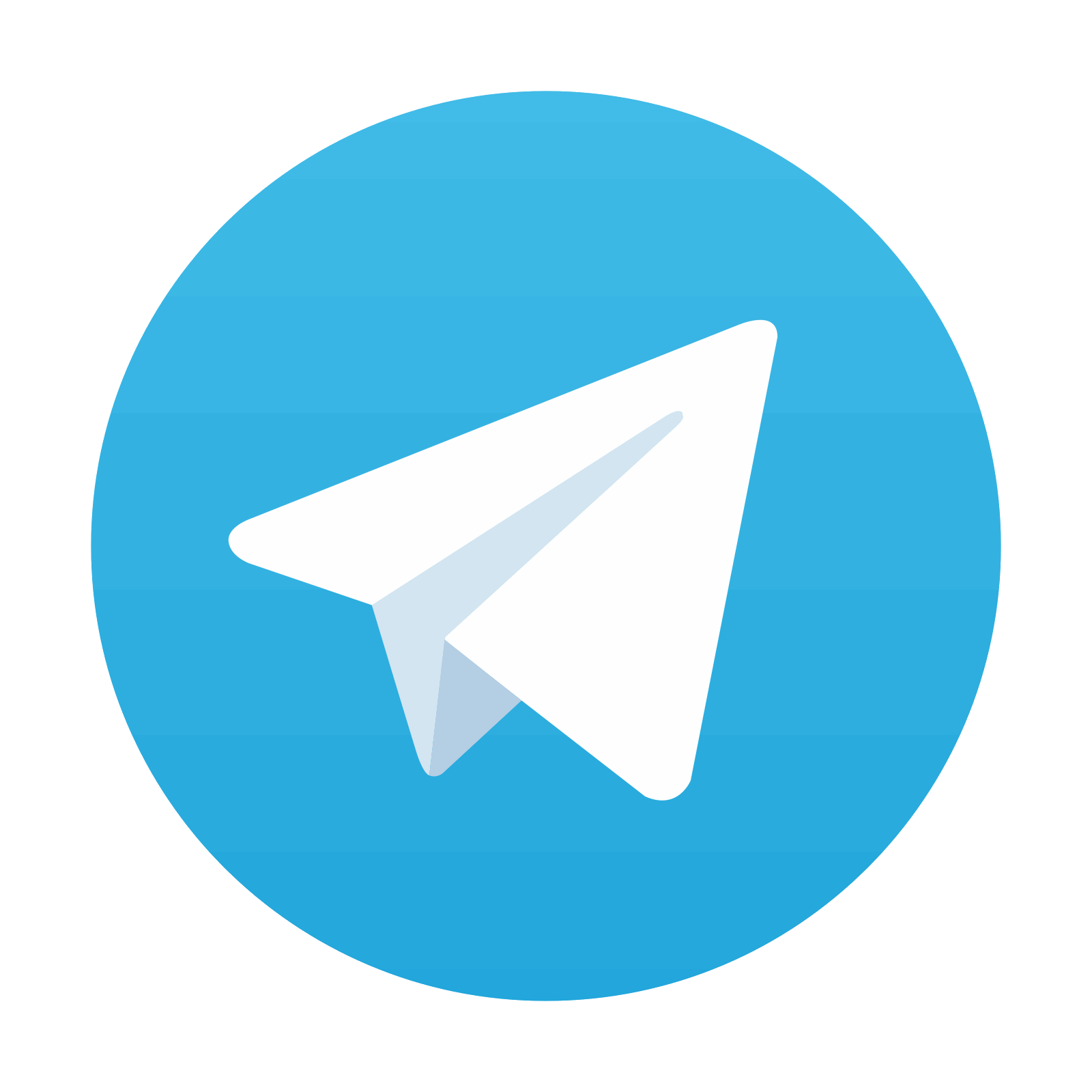
Stay updated, free articles. Join our Telegram channel

Full access? Get Clinical Tree
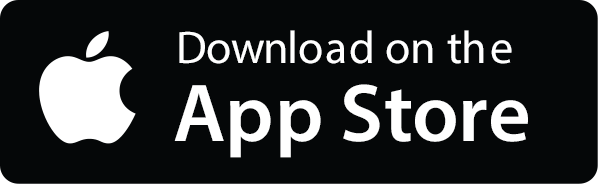
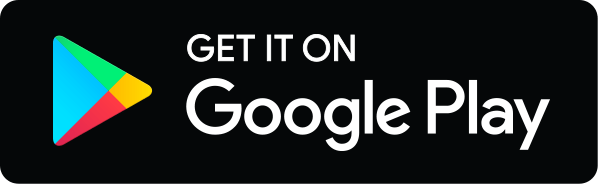