6 Critical Care A. Basics 1. Monro–Kellie doctrine—There is a fixed volume within the skull and any increase in volume of one intracranial component will require a decrease in another component to maintain normal pressure. 2. Cerebral perfusion pressure CPP = mean arterial pressure (MAP) – intracranial pressure (ICP) = (⅔ diastolic blood pressure [BP] + ⅓ pulse pressure [PP]) – ICP. 3. No clear optimal level for CPP, but should be > 50–70 mm Hg 4. Autoregulation a. Cerebral blood flow (CBF) pressure autoregulation is maintained at a constant level despite fluctuation of systolic BP from 50–160 mm Hg. b. Viscosity—alteration of vessel diameter in response to changes in blood viscosity c. Metabolic—CBF responds to brain metabolic demand. d. Blood gas—Hypoxia leads to vasodilatation and Pa CO2 regulates cerebrovascular tone. B. Prevention of secondary injury 1. Cerebral edema—peaks several days following injury, generally due to cytotoxic edema. May increase ICP and decrease CPP. Steroids are of no benefit and are detrimental in traumatic brain injury. 2. Hypotension—results in compensatory vasodilatation and increased ICP. Avoided to maintain CPP. Systolic blood pressure (SBP) < 90 mm Hg is associated with a twofold increase in mortality. 3. Hypoxemia—detrimental from the resultant vasodilatation and ICP increase and lack of O2 to neurons 4. Pyrexia—metabolism increased, resulting in larger energy requirement of brain. 10% increase in metabolism per increased degree centigrade C. Brain oxygen monitoring—may be measured by jugular venous saturation or brain tissue oxygen tension. Jugular venous saturation < 50% or oxygen tension < 15% is threshold to treat D. Brain death 1. Considered in presence of catastrophic central nervous system (CNS) event, no medical conditions and no drug intoxication or poisoning that may confound assessment, temperature > 34°C. 2. Clinical exam—Glasgow Coma Score (GCS) 3, absent pupillary light, corneal, oculocephalic, oculovestibular and gag reflexes, absent cough while suctioning, absent sucking or rooting reflexes and fulfill criteria of apnea test. 3. Apnea test—core body temperature > 36.5°C, SBP > 90 and euvolemia. Absence of respiratory response with PaCO2 > 60 or increase of 20 mm Hg from baseline 4. May supplement with electroencephalogram, somatosensory or brainstem evoked potentials. 5. No CBF may be documented by cerebral angiography, transcranial Doppler or computed tomographic angiography (CTA) and may be necessary when cannot document other features above. A. Narrow complex/supraventricular tachycardia (SVT) (Table 6.1 and Table 6.2)
I. Trauma
II. Cardiac
P Wave Type | Dysrhythmia |
---|---|
Uniform | Sinus tachycardia |
Multiple forms | Multifocal atrial tachycardia |
Inverted | Junctional tachycardia |
Sawtooth | Atrial flutter |
None | Paroxysmal atrial tachycardia, atrial fibrillation |
QRS < 0.12 Seconds (Narrow Complex) Irregular Rhythm | Regular Rhythm | QRS > 0.12 Seconds (Wide Complex) Supraventricular Tachycardia |
---|---|---|
Atrial fibrillation | Sinus tachycardia | SVT with aberrant conduction |
Multifocal atrial tachycardia | Atrial tachycardia | Ventricular tachycardia |
Atrial flutter | Atrial flutter | Torsades de pointes |
| Junctional tachycardia |
|
Abbreviation: SVT, supraventricular tachycardia.
1. SVT includes a rate >100 with QRS complex < 0.12 seconds wide (< small 3 squares), and it implies a supraventricular rhythm (originating at or above the atrioventricular [AV] node) because the His Purkinje system is utilized to allow for fast conduction.
2. Irregular SVT is generally multifocal atrial tachycardia if P waves are present or atrial fibrillation if P waves are not present. Multifocal atrial tachycardia is seen with chronic obstructive pulmonary disease (COPD) and is associated with theophylline. Atrial fibrillation is caused by cardiac disease or hyperthyroidism.
3. Regular SVT has a wider differential, and characterization of P waves may help determine rhythm.
4. If the P wave is normal in rate and morphology then it is likely a sinus tachycardia.
5. If the P waves have a sawtooth pattern with a rate ~250 then it is likely an atrial flutter.
6. If there is no P wave the SVT is likely a junctional tachycardia or a reentrant form.
7. If it is difficult to assess rhythm or there are no visible P waves then you can slow down the rate with vagal maneuvers (i.e., Valsalva or carotid sinus massage) or administer adenosine. If these maneuvers reverse the tachycardia, the underlying condition is likely a reentrant tachycardia.
9. If SVT is in an unstable patient then cardioversion is indicated.
10. If stable then attempt to establish the diagnosis and treat with β-blocker (metoprolol) or Ca2+ channel blocker (verapamil).
B. Wide complex tachycardia
1. Wide complex tachycardia (> 0.12 seconds or 3 small squares QRS duration) is often a ventricular rhythm but may also be an SVT with aberrancy (bundle branch block), a pacemaker, or relating to AV reentrant tachycardia.
2. Ventricular tachycardia (VT) may often be caused by QT prolongation from hypomagnesia, hypokalemia, or iatrogenic (quinidine, digoxin).
3. Polymorphic VT is generally found in an unstable patient following myocardial ischemia but may be seen in Torsades de pointes when a prolonged QT interval is present. May resolve with K+ or Mg2+ replacement.
4. In the stable patient, elective synchronized cardioversion is appropriate treatment for VT. If the patient is unstable, then conscious synchronized cardioversion is recommended; defibrillation if patient is unconscious.
C. Bradycardia
1. Bradycardia—heart rate < 60. If associated P wave with every beat then it is a sinus bradycardia. Sign of increased ICP. Common in athletes or iatrogenic relating to drugs, it may be due to sick sinus syndrome in patients with heart disease.
2. If intermingled with SVT known as tachycardia–bradycardia syndrome.
3. If PR interval longer than 0.2 seconds then it is first degree AV block.
4. Second degree AV block is divided into Wenckebach and Mobitz.
5. Wenckebach is a cyclic progressive blocking of conduction at an AV node, which results in a dropped QRS, characterized by progressive lengthening of the PR interval.
6. Mobitz refers to a series of P waves that cannot conduct through an AV node.
7. Third AV degree block is a complete dissociation of P and QRS waves generally resulting in an escape junctional rhythm.
8. If the patient is symptomatic may treat with atropine, transcutaneous pacer, dopamine, or epinephrine drip. If the patient is not symptomatic but has a Mobitz or third degree AV block may need transvenous pacer.
D. Other electrocardiogram (ECG) findings
1. Digoxin—gradual downward curve of ST segment; causes multiple dysrhythmias and AV blocks
2. Hypocalcemia—increased QT interval
3. Hypokalemia—U-wave
4. Hyperkalemia—peaked T-wave
5. Hypothermia—J-point elevation
6. Hyperthyroidism—atrial fibrillation
7. Quinidine toxicity—prolonged QT interval, notched P wave, wide QRS, ST depression
8. Subendocardial ischemia—ST depression
9. Transmural ischemia—ST elevation
10. Pericarditis—flat or concave ST segment elevation often in all leads
11. Brugada syndrome—right bundle branch block with ST elevation in V1–V3 predisposes to sudden cardiac death
12. Long Q-T syndrome—QT interval is > 50% of cardiac cycle, predisposes to arrhythmias
13. Wellens syndrome—T wave inversion in V2–V3 due to anterior descending coronary artery stenosis
14. Subarachnoid hemorrhage (SAH)—shows peaked T wave and ST depression
15. Pulmonary embolism—Most frequent ECG changes are nonspecific ST and T changes (66%), tachycardia (63%), and rarely the classic “S1Q3T3.”
E. Cardiac medications
1. Antiarrhythmics—class 1 (Na+-channel blockers), class 1A (quinidine and procainamide; may lead to reversible K+-channel blockade resulting in prolonged QT), class 2 (β-blocker), class 3 (K+-channel blocker such as amiodarone, sotalol), class 4 (Ca2+-channel blockers)
2. Adrenergic receptors – α1-receptor stimulation leads to vasoconstriction. β1 receptors increase cardiac rate (chronotropy) and strength of contraction (inotropy). β2 receptors vasodilate.
3. Dopamine receptor—leads to vasodilatation in cerebral, renal, coronary, and mesenteric vasculature
4. Dobutamine—β1 agonist, mild β + α2 agonist, inotropic, causes peripheral vasodilation (reduces afterload), increases cardiac output (CO) with a reflex decrease in systemic vascular resistance (SVR), no change in BP. Side effects include tachycardia if hypovolemic. Contraindications include hypertrophic cardiomyopathy. Dose is 5–15 μg/kg/min up to 40.
5. Dopamine—causes renal, splanchnic, and cerebral vasodilation, increased renal Na+ excretion independent of blood flow and vasoconstriction with higher doses. Useful with cardiogenic or septic shock. Doses are 1–2 μg/kg/min for renal effect and selective vasodilatation. Doses of 5–10 μg/kg/min have more β1 effect leading to higher stroke volume; 10–20 μg/kg/min for α1 and β1 effects. Side effects include tachydysrhythmias.
6. Epinephrine—Strong β1, moderate β2 + α1, drug of choice for anaphylaxis. α1 effects predominate over β2 at higher doses. Dose is 3–5 mL of 1:1000 or 2–4 μg/min. Side effects include myocardial ischemia, dysrhythmia, and acute renal failure.
7. Phenylephrine—Mostly a1 effects with minimal effects on inotropy and chronotropy.
8. Norepinephrine—Agonist for α1 and β1 receptors, may lead to reflex bradycardia following increase in MAP. Drug of choice in septic shock. Dose is 8–70 μg/min. Use with dopamine 1 μg/kg/min for renal protection. Contraindications include renal failure.
9. Vasopressin—noradrenergic pressor, appropriate for late vasodilatory shock
11. Furosemide—a diuretic that increases SVR and decreases CO. Diuresis occurs in 20 minutes–2 hours. Nonsteroidal antiinflammatories (NSAIDs) may block the response. Use for early oliguric renal failure. Dose is 1 mg/kg. Side effects include ototoxicity, hypokalemia, hypomagnesemia, hypochloremia, and metabolic alkalosis. Contraindications include sulfa allergy, hepatorenal syndrome, and edema from nephrotic syndrome.
12. Glucagon—increases heart rate and contractility independent of its β effect. It reverses a β-blocker overdose to help as an inotrope, but not a chronotrope. Consider use with electromechanical dissociation. Dose is 1–5 μg up to 1–20 μg/h mixed with 10 mL saline intravenously (IV). Side effects include hypokalemia and hyperglycemia.
13. Labetalol—blocks α and β receptors to lower BP and does not increase heart rate or change CO. Dose is 2 mg/min or 20–80 mg every 10 minutes up to 300 mg IV.
14. Nitroglycerine—relaxes arteries (> 200 μg/min) and veins (< 50 μg/min) and increases coronary blood flow. Use with myocardial ischemia/infarction (if normotensive), pulmonary hypertension, and heart failure. Dose is 10–200 μg/min. There is decreased tolerance with intermittent infusion (12 hours on/12 hours off) or N-acetylcysteine that replenishes sulfhydryl groups in vessel walls. Side effects include methemoglobinemia that causes cyanosis with a normal blood gas when it is >10% (70% is usually fatal). Treat with methylene blue, 2 mg/kg IV over 10 minutes. Contraindications include increased ICP and closed-angle glaucoma.
15. Sodium nitroprusside—dilates arteries and veins, increases stroke volume, and does not change heart rate. Drug of choice for hypertensive emergencies. Dose is 2–3 μg/kg/min for < 72 hours. Side effects include cyanide toxicity, especially in smokers with decreased thiosulfate. Cyanide is converted to thiocyanate in the liver and is excreted from the kidneys. Symptoms include headache, nausea, vomiting, weakness, hypotension, lactic acidosis, and tolerance to nitroprusside. Keep the cyanide < 5 μg/mL. Prevent toxicity by mixing with 1% thiosulfate solution. Also try to keep the B12 level normal. Thiocyanate toxicity causes acute renal failure, mental status changes and occurs with levels >10 mg/dL. Contraindications include B12 deficiency and renal failure.
F. Cardiopulmonary resuscitation (CPR)—30% survive and 10% recover to baseline. Postresuscitation injury is caused by poor reflow from vasoconstriction (treatment is with Ca2+-channel blockers or Mg2+) and reper-fusion injury by free radicals. Consider giving MgSO4 2 g IV over 20 minutes to prevent “no reflow” from vasoconstriction. Consider Ca2+– channel blockers when not hypotensive. Steroids and barbiturates have not been shown to be of value. Prognosis is related to the ischemic time before and during CPR. 30% of patients in postresuscitation coma regain consciousness. After 48 hours, only 2–7% of patients still in a coma recover; after 7 days, none recover.
G. Air embolism—associated with dyspnea, substernal chest pain, millwheel murmur, focal neurologic deficits, and cardiorespiratory failure. May be diagnosed with echocardiography (presence of air, ventricular dilatation, pulmonary artery hypertension), end tidal CO2 (fall in end-tidal CO2), computed tomography (CT) scan, and pulmonary angiography. The most sensitive monitor is the precordial Doppler. Treated with left lateral decubitus position, nitrogen washout (high flow O2), hyperbaric O2, or aspiration of air from venous circulation. Mortality rate is 15%.
III. Respiratory
A. Oxygenation
1. O2 content = 1.34[Hb g/dL] × O2 saturation + (0.003 × PaO2) = O2 mL/100 mL
2. O2 delivery = O2 content × CO. Normal 520–570 mL/min/m2
3. O2 uptake = CO × (arterial O2 content – venous O2 content). Normal 110–160 mL/min/m2
4. O2 extraction ratio = Uptake/Delivery × 100 (22–32%)
5. If the O2 extraction ratio exceeds 60%, uptake becomes dependent on delivery.
6. All tissues increase O2 extraction in the face of decreased blood flow except the coronary circulation, which is always at its maximal extraction rate (flow dependent).
7. Mixed venous O2 is measured in the pulmonary artery, and normal is 68–77%. It is lower with hypoxemia, increased metabolic rate, decreased CO, and anemia.
8. Lactate increases if the O2 delivery is less than the metabolic demand and the cells are forced to use anaerobic glycolysis. Normal blood level is < 2 mmol/L, and > 4 mmol/L is abnormal.
9. Lactate may be elevated without hypoxemia, but with hepatic insufficiency (decreased clearance), thiamine deficiency (interferes with glucose metabolism), infection (endotoxin release affects glucose metabolism), and respiratory alkalosis.
10. The plateau of the oxygen–hemoglobin dissociation curve begins at PO2 60 mm Hg, and O2 saturation 90%. Below this point, the O2 saturation drops much more quickly with decreases in O2 pressure.
11. Bohr effect
a. Right shift—occurs in tissues and there is decreased O2 affinity. A right shift occurs with increases in H+, CO2, temperature, and 2,3-diphosphoglycerate (DPG).
b. Left shift—occurs in the lung and there is increased O2 affinity. A left shift occurs with decreases in H+, CO2, temperature, and DPG, as well as with banked blood (due to decreased DPG). Hemoglobin has less affinity for O2 in the tissues where there are higher H+ and CO2 levels.
12. Oximetry detects red oxyhemoglobin and infrared deoxyhemoglobin in a photodetector. Pulse oximetry samples only pulsatile vessels (arteries) because it detects volume fluctuations. It is not affected by most skin tissue thickness, pigments, or nail polish. Oximetry becomes less accurate when the O2 saturation is below 70%.
13. Nasal cannula providing 100% O2 at 1–6 L/min produces a FiO2 of 0.21–0.46.
14. The maximum FiO2 that can be achieved with a nasal cannula is 46%. This is with 6 L/min minute ventilation, and it is even less with tachypnea.
15. Standard mask providing 5–10 L/min of 100% O2 can produce a FiO2 of 40–60%. This level is lowered with increased respiratory rates caused by inhalation of ambient air.
16. Partial rebreather mask (no ambient air allowed, rebreathing done into mask) at 5–7 L/min provides a FiO2 of 75%.
17. Nonrebreather mask (no ambient air allowed, exhaled breath is not reinhaled) at 4–10 L/min provides a FiO2 of 100%.
1. Anatomic dead space (= 150 mL) includes the trachea, bronchi, and distal parts of the respiratory tree that are not used for gas exchange.
2. Physiologic dead space includes the alveoli where gas is not equilibrating with the capillary blood.
3. Total dead space normally makes up 20–30% of minute ventilation. In the normal alveoli, the arterial and expired PCO2 are equal because no dead space is present.
4. Increased dead space is caused by overdistended alveoli (COPD and positive end-expiratory pressure [PEEP]), destroyed alveolar–capillary interface (emphysema), or decreased blood flow (congestive heart failure, pulmonary embolus).
5. The O2 pressure in the alveolus— (PA O2) = FiO2 (Pb – Pwater) – (Pa CO2/RQ) = 0.21 (713 mm Hg) – Pa CO2/0.8, where Pb is atmospheric pressure and RQ is the respiratory quotient.
6. The A-a gradient is the alveolar to arterial O2 gradient (PAO2 − PaO2). The PAO2 = FiO2 (Pb – P water) – (Pa CO2/RQ) and is normally 100 mm Hg at FiO2 of 0.21 producing an A-a gradient of 10–20 mm Hg. The normal A-a gradient increases 6 mm Hg for each 10% increase in FiO2 and is 60–70 mm Hg at a FiO2 of 1.0. Increased FiO2 causes an increased A-a gradient because there is less hypoxic vasoconstriction in the poorly ventilated areas.
7. In hypoxemia if the A-a gradient is normal, there is likely no primary cardiopulmonary problem so consider neuromuscular or central nervous system (CNS) causes. If there is an increased A-a gradient and the venous PO2 is > 40 mm Hg, the problem is caused by increased dead space or shunting. If the venous PO2 is < 40, the problem is caused by decreased blood flow or a high metabolic rate.
8. Hypercapnia can be caused by increased production of CO2 from sepsis, trauma, burns, increased carbohydrate intake, and acidosis.
9. RQ = VCO2/VO2 and is the ratio of molecules of CO2 created for each O2 used. Normal is 0.8; carbohydrates are 1, proteins are 0.8, and lipids are 0.7. Therefore, an increased carbohydrate load creates more CO2 molecules to expel.
10. Hypercapnia can also be caused by hypoventilation with a normal A-a gradient as with sleep apnea, myasthenic syndrome, Guillain–Barré syndrome, phrenic nerve injury, peripheral neuropathy, muscle weakness (from decreased phosphate or Mg2+, sepsis, and shock), opiates, and lidocaine.
C. Pulmonary disorders
1. Acute lung injury (ALI)—defined as lung inflammation resulting in increased pulmonary capillary permeability. Characterized by bilateral chest infiltrates with pulmonary capillary wedge pressure (PCWP) < 18 mm Hg (or no suspected heart failure) and PaO2/FiO2 between 201 and 300 mm Hg
2. Acute respiratory distress syndrome (ARDS)—more severe ALI with worsening hypoxia (PaO2/FiO2 < 200 mm Hg). There is diffuse alveolar damage. Protein leaves vascular space losing colloid osmotic pressure gradient, which prevents fluid reabsorption from alveoli. The alveoli fill with inflammatory fluid. Acute onset and may persist for weeks. Causes include sepsis, aspiration, infectious pneumonia, trauma, burns, and pancreatitis. Mortality rate is over 30%.
3. Differentiating ARDS from cardiogenic pulmonary edema—pleural effusions more common in heart failure and PCWP >18 mm Hg. ARDS has high protein levels from bronchioalveolar lavage (Table 6.3).
ARDS | Hydrostatic Edema |
---|---|
Early hypoxemia | Late |
Diffuse infiltrate | Patchy |
Peripheral vascular prominence | Perihilar vascular prominence |
No Kerley B lines | Kerley B lines |
Clear lung bases | Obscured bases |
More protein in fluid | Less protein in fluid |
Associated with sepsis, trauma, and multisystem organ failure | Associated with hypertension, myocardial infarction, and acute renal failure |
Abbreviation: ARDS, acute respiratory distress syndrome.
4. Diuretics are of no benefit in ARDS because the fluid leaking into the alveoli is the result of acute inflammation.
5. CO can be increased (with dobutamine) to increase the O2 delivery.
6. Vasodilators are not indicated because they may increase the pulmonary shunt fraction.
7. Steroids have not been proven to be of benefit.
8. Cheyne–Stokes breathing—gradually increasing and decreasing tidal volume mixed with periods of apnea. Often an ominous sign, the respiratory center does not respond properly to PCO2 or PO2.
D. Respiratory medications
1. β2-agonists—work best when inhaled with a nebulizer such as albuterol 5 mg (0.1 mL of 5%) every 4 hours. Also consider terbutaline, metaproterenol, and isoetharine. At high doses they may stimulate β1-receptors and cause tachycardia, hypokalemia, or tremors. If this happens, decrease the dose. They work best with asthma, but occasionally help with COPD.
2. Theophylline—questionable benefit for asthma. Works by increasing the cyclic adenosine monophosphate (cAMP) level. Side effects include seizures, dysrhythmias, hypokalemia, and hypotension. These are much more likely with a level > 20. Treat overdose with oral charcoal 20 g orally every 2 hours for 6 doses, even if it is already removed from the blood.
3. Anticholinergics—atropine 0.025–0.075 mg/kg inhaled every 3 hours. Ipratropium, 0.02–0.03 mg/kg inhaled every 3 hours (less systemic side effects). They work by decreasing the parasympathetic input that constricts small airways.
4. Steroids—synergistic with β-agonists in the treatment of asthma but not with COPD, ARDS, sepsis, or anaphylaxis. Hydrocortisone 2 mg/kg IV or 0.5 mg/kg hourly; methylprednisolone 40–125 mg IV every 6 hours.
5. Doxapram—stimulates both peripheral chemoreceptors and brainstem respiratory centers. Side effects include hypertension, tachycardia, and seizures. The dose is 1–3 mg/min IV up to 600 mg.
7. Heliox—a combination of helium and O2 that results in a reduction of turbulent air flow. Greatest benefit in acute upper airway obstruction
E. Paralytics, sedatives, muscle relaxants
1. Side effects—decreased ability to clear pulmonary secretions even with suctioning (increased risk of pneumonia and mucous plug) and increased risk of venous thromboemboli
2. Halothane—causes central depression and bronchodilation
3. Vecuronium—a nondepolarizing blocker, has the least histamine release, dose is 0.1 mg/kg, duration is 30 minutes
4. Pancuronium—a nondepolarizing blocker, dose is 0.01 to 0.05 mg/kg every hour, duration is 1 hour, side effects include tachycardia
5. Succinylcholine—a depolarizing blocker, short acting, increases the K+ level; contraindicated with hyperkalemia, hemiplegia, or other neurologic disorders associated with weakness
6. Reversal agents—used to counteract muscle paralysis of competitive acetylcholine receptor blockade. The local acetylcholine levels are increased by acetylcholinesterase inhibitors such as neostig-mine 2.5–5 mg/70 kg. Pretreat with atropine (0.6–1.5 mg/70 kg) to prevent bradycardia from the increased parasympathetic stimulation by the increase in acetylcholine levels. Onset may be delayed 15–30 minutes.
7. Haloperidol—does not cause respiratory depression or hypotension (unless used with propranolol). Dose is 3–5 mg IV up to 10 mg, wait 20 minutes and double the dose. If this is ineffective, change agents or add benzodiazepines. There are less extrapyramidal side effects with the IV route. This condition is associated with neuroleptic malignant syndrome characterized by hyperthermia, muscle rigidity autonomic dysfunction, and confusion. This condition may occasionally be fatal and should be treated with dantrolene. Haloperidol also lowers seizure threshold.
8. Diazepam—1–10 mg IV
9. Lorazepam—0.04–0.05 mg/kg, good amnestic
10. Midazolam—1 mg IV every 3 hours up to 0.15 mg/kg or 0.4 μg/min, good amnestic
11. Morphine—increases histamine release and worsens asthma
K. Mechanical ventilation
1. Four modes—volume-, pressure-, time-, or flow-cycled
2. Volume-cycled—Ventilation is entirely dependent on preset tidal volume, respiratory rate, and inspira-tory flow.
a. Controlled mechanical ventilation—Ventilation is only dependent on rate and tidal volume settings, which is appropriate if the patient is not making respiratory efforts.
c. Intermittent mandatory ventilation—Preset tidal volume and rate is delivered at a regular interval and patient may breathe spontaneously as well.
3. Flow-cycled—pressure support once the patient triggers a breath, a preset pressure is delivered until flow tapers
4. Tidal volume—normally at 5–6 cc/kg in humans
5. Rate—Start at 12 for adults.
6. Sigh—a breath 1.5 × tidal volume, not shown to be of benefit
7. Monitoring lung mechanics—assess without mechanical ventilation. Test lung volume for elastic recoil and expiratory flow rate for resistance.
8. Proximal airway pressure—The peak end-inspiratory pressure is proportional to the inflation volume, the resistance of the airways, and the lung and chest wall compliance. It is proportional to R + 1/C, where R is airway resistance and C is lung and chest wall compliance.
a. At a constant volume, an increased inflation pressure is due to either increased resistance or decreased compliance.
b. The plateau pressure is proportional to 1/C.
c. Increased plateau pressure is due to decreased compliance. Increased peak pressure with no change in plateau pressure is due to increased airway resistance.
d. Increased peak and increased plateau pressures are due to decreased lung/chest wall compliance. Compliance = Volume change/Pressure change = tidal volume/plateau pressure cm H2O. Normal is 0.05–0.07 L/cm H2O.
9. Acute respiratory failure—usually caused by pneumonia, edema, and COPD. These do not respond to steroids or bronchodilators. If the patient is not on a ventilator, check for airway obstruction at the bedside with a peak expiratory flow rate (positive if >15% increase noted). If the patient is on a ventilator, assess the peak inspiratory pressure.
10. If there is a sudden respiratory deterioration:
a. Peak pressure increased with normal plateau pressure—likely increased airway resistance. Treat with suction or bronchodilation.
b. Peak and plateau pressure increased—likely decreased compliance or auto PEEP (with obstructive lung disease). Likely due to pneumothorax, atelectasis, edema, or abdominal distension
c. Peak pressure decreased—assess for air leak
11. PEEP should help increase compliance and decrease plateau pressure. In determining efficacy, be sure to subtract the PEEP setting from the measured plateau pressure to obtain the correct value.
12. Increased PEEP causes decreased preload and decreased contractility (by decreasing coronary blood flow). Determine the O2 delivery and assess if there is decreased plateau pressure (from increased compliance) to find best PEEP setting. The O2 saturation value may be misleading because it may associated with decreased O2 delivery. The relationship between O2 be saturation and O2 delivery depends on the cardiac output (Table 6.4).
O2 Saturation | Cardiac Output | O2 Delivery |
---|---|---|
Increased | No change | Increased |
Increased | Decreased | No change |
Increased | More decreased | Decreased |
13. PEEP is not very effective for localized disease. It works mainly on the normal areas of the lung, over-distending the alveoli and redirecting the blood to areas with poor ventilation. Complications include barotrauma, fluid retention (by atrial compression that increases atrial natriuretic factor secretion), decreased CO, and increased ICP.
14. After extubation, the most frequent respiratory problem is laryngeal edema. When severe, treatment is with reintubation or emergent tracheotomy. Epinephrine and steroids are not proven to be helpful.
15. Tracheotomy
a. benefits—clearing of secretions, decreased laryngeal injury and better patient comfort
b. Complications—5% of cases, include pneumothorax, laryngeal injury, nerve injury, hemorrhage, decannulation, and tracheal stenosis
16. Barotrauma—occurs in 43% of patients if the peak inspiratory pressure is > 70 cm H2O, and in 0% if it is < 40 cm H2O. Assess for subcutaneous emphysema, interstitial emphysema, pneumothorax, and pneumoperitoneum.
17. Weaning from the ventilator—Typical parameters are PO2 > 60 mm Hg with FiO2 < 0.6 without PEEP, tidal volume > 5 mL/kg, vital capacity >10 mL/kg, minute ventilation < 10 L/min, and negative inspira-tory force > 25 mm H2O. 30% that fulfill these parameters still fail, and 30% who do not fulfill them tolerate extubation. Consider a continuous positive airway pressure (CPAP) trial and check arterial blood gases in 30 minutes as opposed to intermittent mandatory ventilation or pressure support weaning.
IV. Renal
A. Creatinine clearance = (140 – age) × weight (kg)/72 × serum creatinine (mg/dL)
B. Fractional excretion = (urine Na+/plasma Na+)/(urine creatinine/plasma creatinine) × 100
C. Oliguria—urine production < 400 mL/24 hours
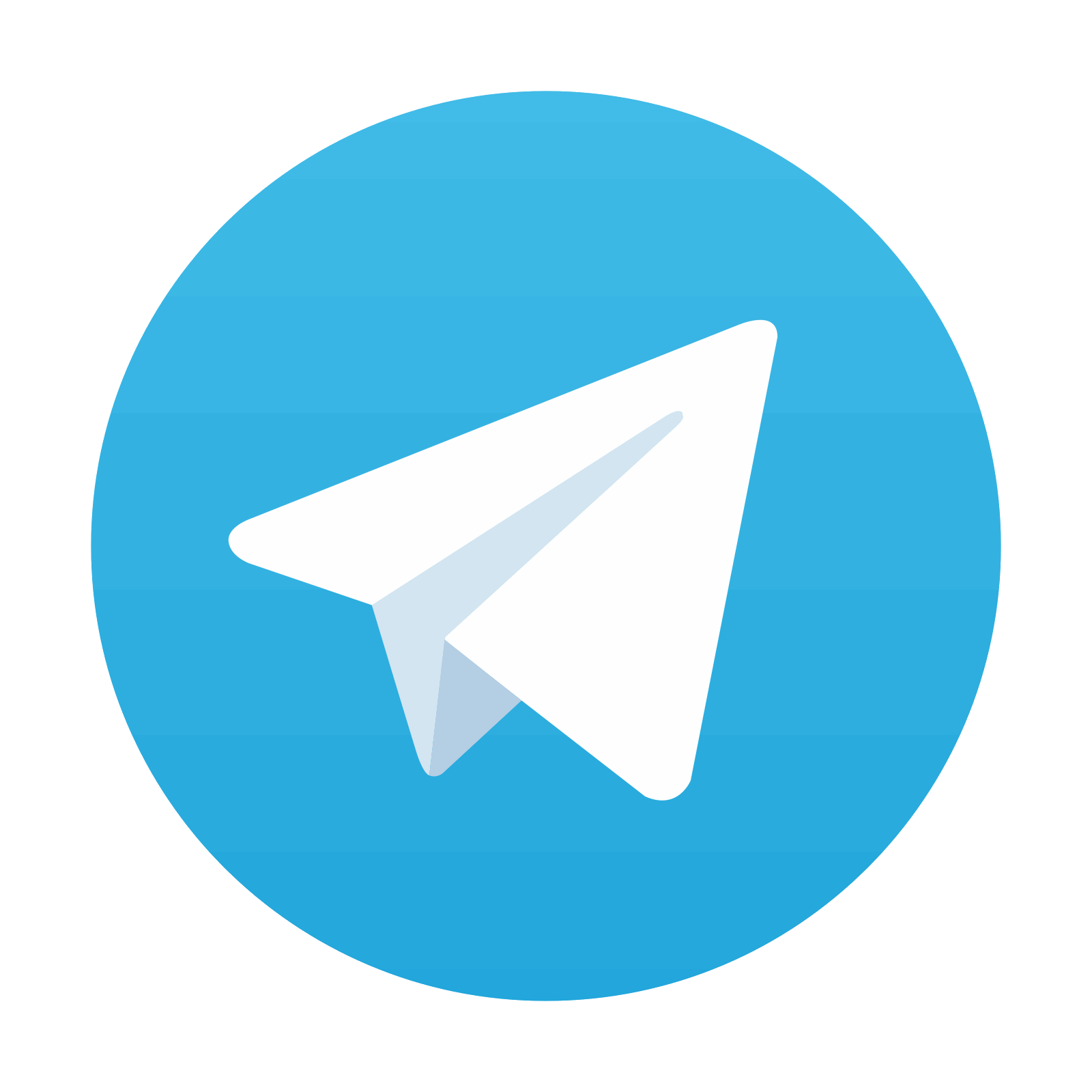
Stay updated, free articles. Join our Telegram channel

Full access? Get Clinical Tree
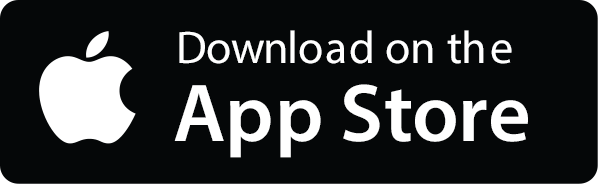
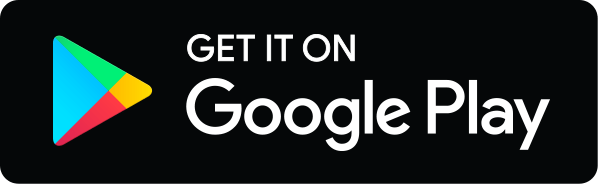