Cytogenetic/Molecular Biology: Recent Advances in the Cytogenetics and Molecular Biology of Pediatric Brain Tumors
Brain tumors are the most common pediatric solid tumors, accounting for approximately 15 to 30% of all childhood cancers.1 Despite the improvement in diagnostic procedures and the introduction of multimodal treatment strategies, the complete cure of these tumors continues to be a tremendous challenge. Brain tumors have remained the major cause of tumor-related mortality in the pediatric population,2 with survivors commonly suffering from treatment-related sequelae.3
Recent advances in molecular biology have contributed to increasing our understanding of the molecular events associated with the tumorigenesis and pathogenesis of brain tumors during the past several decades.4 In particular, the last decade can be characterized by the widespread application of novel technology in the molecular biology of brain tumors. Although our understanding of the molecular mechanisms underlying pediatric brain tumor pathogenesis is not yet complete, the application of these next-generation laboratory-based strategies to pediatric brain tumors is rapidly closing the gap to improving the survival of patients and their quality of life in a tangible manner. This chapter summarizes the recent advances in techniques used in genomics, epigenomics, transcriptomics, and proteomics during the last several years, and discusses their application with regard to therapeutic strategies in pediatric brain tumors including medulloblastomas, ependymomas, and pediatric gliomas ( Fig. 6.1 ).
Analysis of the Cancer Genome
Cancers, including malignant pediatric brain tumors, are essentially a disease of the human genome. Structurally, the human genome consists of 3 billion base pairs of DNA that encode an estimated 25,000 unique proteins. During the course of tumori-genesis, the DNA undergoes numerous alterations ranging from somatic base pair mutations that are characterized by single base pair substitutions as well as insertions or deletions of short DNA segments. In addition, the DNA can undergo more dramatic structural changes including translocations, duplications, inversions, and deletions. Conservative estimates based on large-scale genome-wide sequencing projects indicate that a given tumor type may accumulate 40 to 100 somatic mutations; however, this does not include structural or copy number changes.5,6 Important consideration must be paid to discriminating between which of the identified mutations and alterations are “drivers” and which are “passengers.” The cancer genome is highly unstable, and not all of the genome alterations are either causative or required for maintenance of a given tumor type. To distinguish which mutations provide a growth advantage (i.e., drivers) from those that do not (i.e., passengers), studies must incorporate data from multiple integrated approaches into a comprehensive study including expression, copy number, sequencing, and functional validation.
Historically, genomic characterization of tumors involved gross chromosomal alterations revealed through techniques such as karyotype analysis or comparative genomic hybridization (CGH). An alternate technique, with similar resolution, is fluorescent in-situ hybridization (FISH), which is used to detect or localize specific DNA sequences and is frequently used in candidate screening approaches or in validation procedures. For example, Fig. 6.2 is a representative image depicting amplification of the EGFR gene in a pediatric glioma sample (Stefan Pfister, unpublished data). Karyotype analysis of medulloblastoma by several groups identified the isochromosome of the long arm of chromosome 17 (isochromosome 17q), and it is now recognized that this aberration is the most common isochromosome in cancer.7–10
Studies utilizing CGH on medulloblastoma samples revealed several oncogenes such as erbb2, mycc, and mycn as well as tumor suppressor genes such as PTCH1,11–13 and subsequent studies focused on individual gene loci in a candidate approach to identify molecular defects in pediatric brain tumors.
Evolving Technologies in Pediatric Brain Tumor Genomics
Numerous new technologies have advanced the genomic investigation of brain tumors during the past 5 years as high-resolution genomic technologies have become available for mainstream utilization. In particular, one of the most significant events has been the application of DNA microarrays to genomics including array comparative genomic hybridization (aCGH) and high-resolution single nucleotide polymorphism (SNP) arrays. aCGH enables a genome-wide search for prospective tumorrelated gene candidates with a resolution from 103 to 106 base pairs compared with a 10-fold lower resolution with conventional CGH. A seminal study by Mendrzyk et al14 employed aCGH on 47 medulloblastoma samples; the authors identified a recurrent amplification of the CDK6 proto-oncogene. They validated their findings with FISH and established a negative correlation between high CDK6 expression and overall survival using a medulloblastoma tissue microarray (TMA).14 Since this initial study, multiple genes such as FOXG1, MYCL1, PDGFRA, KIT, MYC, and MYCN have been identified in medulloblastoma using aCGH.15–17 aCGH has also been used to characterize ependymoma, where amplicons containing the oncogenes VAV1 and hTERT were identified and were able to refine a previously reported deletion on chromosome 9 to a 100-kilobase (kb) region that corresponds to the INK4A/ARF loci.18–20 Perhaps one of the most pivotal discoveries in the study of pediatric low-grade gliomas was the application of aCGH by Pfister et al,21 who conducted genome-wide profiling of 66 low-grade pediatric astrocytomas and identified copy number gains at the BRAF locus.21 Given that BRAF activating mutations have been detected in other cancers, and that BRAF can be targeted therapeutically with inhibitors, this study provides exciting therapeutic opportunities for the subset of pediatric patients with low-grade astrocytoma harboring BRAF amplifications.22


The high-resolution SNP array has been developed more recently, and similarly utilizes microarray technology to detect loss of heterozygosity (LOH) and copy number alterations across the genome.23,24 Notably the use of SNP arrays has profoundly increased the resolution of detection. In comparison to aCGH, which has an estimated resolution of 500 kb, the resolution of current SNP arrays approaches 2.5 kb. Typically the data are represented as gains and losses on each chromosome as shown in Fig. 6.3 , which details the copy number variations in medulloblastoma samples for chromosome 17. Currently the largest survey of the medulloblastoma genome by SNP array analysis was conducted by Northcott et al25; over 200 primary medulloblastoma samples and cell lines were analyzed by high-resolution SNP array, and the authors identified 191 high-level amplifications and 159 homozygous deletions that were previously unreported. SNP array profiling has also been used on other pediatric brain tumors. Wong et al26 compared the genome-wide profiles of 14 high-grade to 14 low-grade pediatric gliomas and observed in the low-grade gliomas a relatively balanced genome that appeared, in large part, normal. In contrast, the high-grade glioma profiles exhibited amplifications in EGFR and PDGFR as well as loss of heterozygosity affecting numerous loci on different chromosomes.26 Remarkably, SNP array profiling has also been recently applied to the study of diffuse intrinsic pontine gliomas (DIPGs), which are among the most devastating pediatric brain tumors; they diffusely involve the brainstem, rendering them refractory to surgical resection. Zarghooni et al27 obtained 11 tumor samples from nine postmortem and two pretreatment surgical patients and identified and validated recurrent changes that were distinct from high-grade astrocytomas including copy number gains at PDGFRA and adenosine diphosphate (ADP)-ribose polymerase (PARP)-1 loci. Although aCGH and SNP array profiling have provided essential information about the genomes of pediatric brain tumors, recent breakthroughs in DNA sequencing technologies will likely replace array-based techniques in the near future.
One of the most significant advances in genomics in recent years is the development of advanced DNA sequencing techniques, so-called next-generation sequence (NGS) or second-generation sequence techniques. There are several second-generation platforms currently available on the market, namely 454 (Roche; Branford, CT),28 Gene Analyzer (Illumina; San Diego, CA),29,30 SOLiD (Applied Biosystem; Carlsbad, CA),31 Heliscope (Helicos; Cambridge, MA),32 and Polonator (Dover Systems; Salem, NH).33 In contrast to the conventional sequencing method utilizing polymerase chain reaction (PCR)-based Sanger sequencing, second-generation sequence methods employ new sequencing theories such as pyrosequencing, sequencing by synthesis, and sequencing by ligation.34 Despite differences in the techniques, the second-generation sequencing methods have commonly achieved several hundred times more processivity than the conventional sequence method based on Sanger sequencing, thus allowing much higher throughput of analysis in a single experiment with a lower per base cost. Furthermore, they can detect copy number alteration events and chromosomal rearrangements at single base pair resolution.5,35,36 Currently, mainstream utilization of these technologies is hampered by technology access and cost-associated limitations. However, advances in whole-cancer genome sequencing will undoubtedly accelerate the understanding of the molecular biology underlying each of the pediatric brain cancers, and improve diagnosis and treatment of these cancers.
Elucidation of the Cancer Epigenome
In addition to the genomic modifications that arise in cancers, epigenetic factors can also invoke oncogene activation or tumor suppressor gene silencing. The main epigenetic mechanisms involved in gene regulation include DNA methylation, histone modifications, and alterations in microRNA (miRNA) expression. These mechanisms can control gene expression in a heritable fashion without any changes to the DNA sequence itself. Promoter CpG dinucleotide-rich region (“CpG island”) methylation is generally associated with transcriptional repression through the conversion of DNA to a closed heterochromatin state, the prevention of transcription factor binding, or the recruitment of transcriptional repressor molecules.37 Various posttranslational histone modifications such as acetylation and methylation of the N-terminal tails of histone proteins have been referred to as the “histone code.” In general terms, acetylation is associated with a transcriptionally competent euchromatin structure, whereas deacetylation results in a transcriptionally repressed heterochromatic configuration. Histone methylation is associated with changes in chromosomal organization, efficiency of DNA repair and gene expression, or silencing, depending on the specific histone protein and lysine residues targeted by this modification.38,39 MicroRNAs are a group of small, noncoding RNAs regulating a variety of cellular processes including development, differentiation, proliferation, and apoptosis.40,41 The short nature of mature miRNAs and the somewhat liberal requirements for target recognition make it possible for a single miRNA to potentially regulate hundreds of distinct messenger RNAs (mRNAs).42 Although miRNAs are probably best described for their role in developmental processes, several reports implicate that deregulation of miRNAs contributes to the pathogenesis of human disease, particularly cancer.43–45

Emerging Techniques in Pediatric Brain Tumor Epigenomics
Several techniques have been developed to exploit the differences in epigenetic modifications between normal and brain tumor samples. One of the earliest studies to use an unbiased whole-genome screening approach was by Fruhwald et al,46 who utilized restriction landmark genomic scanning to identify DNA methylation patterns in 22 medulloblastoma samples and observed the association of poor prognosis with hypermethylation. Microarray technology has recently been adapted to identify differences in global DNA methylation. This technique involves sequential enzymatic digestions with methylation-insensitive and methylation-sensitive enzymes. PCR amplification of uncut fragments (i.e., methylated segments) is performed before hybridization to a microarray encoded for CpG islands of the genome. Using this technique, Waha et al47 determined that the SCGNE1 (secretory granule neuroendocrine protein 1) gene was hypermethylated in 70% of 23 primary medulloblastomas, which correlated with loss of expression. Expression could be restored in medulloblastoma cell lines following treatment with the demethylating agent 5-aza (5-aza-2ʺ-deoxycytidine), which resulted in growth suppression and reduced colony formation, suggesting that this gene played a potential tumor suppressive role in medulloblastoma. Subsequently, the 5-aza compound has been used by several groups to uncover novel tumor suppressors in medulloblastoma. Anderton et al48 used three medulloblastoma cell lines and compared treatment with 5-aza to untreated cells by microarray. This approach, followed by gene expression validation, identified COLIA2 as an epigenetically silenced gene in medulloblastoma. In a similar study, Kongkham et al49 screened a larger cohort of nine medulloblastoma cell lines for comparative re-expression following 5-aza treatment. In addition, this study integrated multiple selection criteria for the identification of candidates for further analysis including greater than twofold upregulation in at least two cell lines, requirement for a defined promoter CpG island, and candidates that were simultaneously identified as targets of LOH through SNP genotyping.25 Kongkham et al identified SPINT2, a negative regulator of the HGF/cMET signaling pathway, as a validated novel tumor suppressor gene that is epigenetically modified in medulloblastoma.
Whole-genome epigenetic screens have also been used to identify differential methylation patterns in pediatric glioma and ependymoma. Vladimirova et al50 used microarray-based differential hybridization to compare high-grade pediatric gliomas to low-grade or normal samples and identified frequent hypermethylation at two CpG sites in the promotor of the Limhomobox 9 (LHX9) gene and determined that re-expression of this gene resulted in decreased migration and invasion. In a separate study, Xie et al51 reported a study that utilized high-throughput bisulfite sequencing to generate methylation profiles for Alu repeats and identified a defined set of CpG sites that either gain or lose methylation in ependymoma samples compared with normal brain, and determined that aberrant methylation of several loci was associated with aggressive and recurrent tumors.
In the last few years there has been abundant evidence to support miRNAs acting as oncogenes and tumor suppressors.52–55 In medulloblastoma, there have also been several reports about the role of miRNAs in tumorigenesis, differentiation, cellular proliferation, biological activity, and clinical features through modulating hedgehog signaling, Notch/HES1 signaling, and over-expression of Her2 and c-Myc.52,56,57
Clearly epigenetic modifications play a significant role in the pathogenesis of pediatric brain tumors. Currently, emerging techniques in epigenetics involve the coupling of protocols to enrich for epigenetic modifications such as methylation-dependent immunoprecipitation (MeDIP) or chromatin immunoprecipitation (ChIP) to high-resolution microarrays (called MeDIP-chip or ChIP-chip) and next-generation sequencing applications (ChIP-Seq, MeDIP-seq).
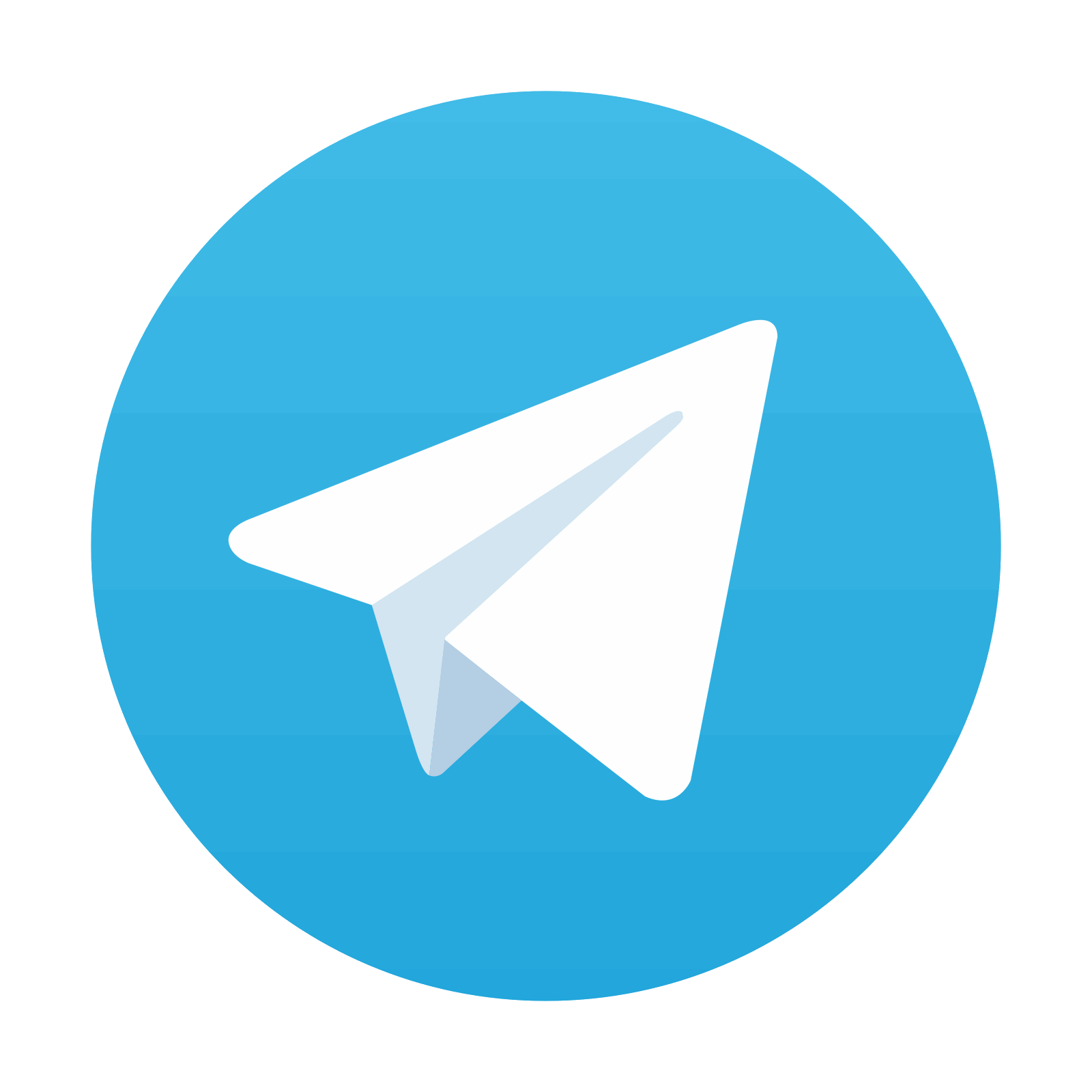
Stay updated, free articles. Join our Telegram channel

Full access? Get Clinical Tree
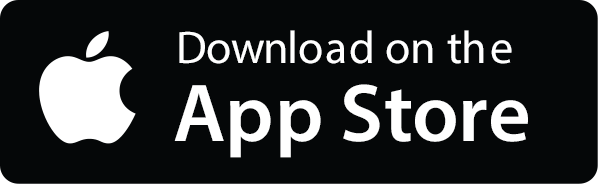
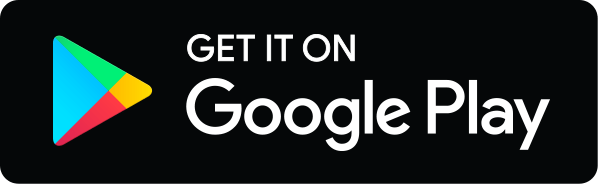
