Fig. 2.1
Dorsal view of a chick embryo (8 somite stage) showing the neural tube (NT) that occupies the medial region and the paraxial mesoderm with the cephalic mesoderm (CM) and the somites (arrows)
2.1.2 An Anatomical Lecture of the Development of the Occipital Bone
The classic description of medical anatomists, widespread in all their works, claims that the occipital bone of vertebrates is formed by four independent subunits. These are called: the basioccipital, the exooccipital, the supraoccipital, and the interparietal. A modern reader may reasonably wander about the relevance of this description for which the components of the occipital bone are in fact different morphogenetic elements. To unravel this mystery, we need to appeal to the onset of ossification. Thus, the occipital bone that is one of the chondrocranium arises from a unique cartilaginous scaffold. Within the latter, ossification centers appear. Medical anatomists have therefore proposed that each of these points is actually a primitive bone that merges to create the definitive bones. This argument seems more obsolete now. Indeed, the endochondral ossification process develops late in development and does not prejudge the embryological data. Thus, the basioccipital, the exooccipital, and the supraoccipital cartilage matrix derived from a single primordium while the interparietal is a membranous bone. Sagacious readers, if they observe the primordial cartilage matrix, cannot recognize the different elements that have been described by the anatomists. Thus, it seems much more rigorous and much closer to the true reality of opposing a chondro-occipital (composed by the basioccipital, the exooccipital, and the supraoccipital) which is closely linked to the chondrocranium and an interparietal which is actually a bone of the skull vault the cells of which derived from neural crest.
2.1.3 Towards an Embryological Reading of the Occipital Bone
An avian fate map is available for the skull vault using the quail-chick chimera technique described by Nicole Le Douarin in 1969 [11]. This technique is based on the possibility of recognizing a nucleus of quail from that of chicken. Early studies were based on histochemical staining, the so-called Feulgen-Rossenbeck reaction, allowing to highlight DNA. In the quail, the heterochromatin is condensed and associated with the nucleolus when it is more diffuse in the chicken (Fig. 2.2). However, even if this technique has been very helpful, it generally does not allow immunostaining or in situ hybridization on the same sections. Only some very resistant antigens can produce an immunohistochemical reaction after Feulgen-Rossenbeck staining [12]. Thus, to differentiate the nuclei of quail and chicken, a monoclonal antibody (QCPN) that specifically recognizes quail nuclei but not the chicken is currently used (Fig. 2.3). This technique allows to constructing fate maps of embryonic territories. Consequently, Couly et al. [13] show that the skull has three distinct embryonic origins: the cephalic mesoderm (corresponding to the paraxial mesoderm located rostrally to the first somite) yields the supraoccipital, the postorbital, the orbitosphenoid, and the pleurosphenoid bones. The first somites yield the exo- and the basioccipital. All the rest of the skull derives from the mesencephalic neural crest. In this study, the origin of the interparietal bone (a very tiny structure in birds located between the parietal and the supraoccipital bones) has not been reported. Furthermore, the cells of the mesencephalic neural crest differentiate into meninges, smooth muscle fibers of the media of the brain and cephalic vessels, and dermal cells. This work has been challenged more recently by a second group of embryologists [14]. Although the experimental approach is similar in both groups, the results are discordant. In particular, the exooccipital only derives from the first somite according to Couly et al. while Huang et al. recognize the participation of the first five somites. Similarly, the basioccipital comes from somites 2, 3, and 4 for Couly et al. and from the first five somites for Huang et al. The supraoccipital origin would be the cephalic mesoderm for Couly et al. whereas it is considered to derive from somites 1 and 2 for Huang et al. These conflicting results show the difficulty of interpreting experimental manipulations performed in the embryo. They must invite us to be very cautious in the use of these works for the understanding of human malformations. Indeed, one cannot prefer the results of a group compared to the other. Furthermore, the shape of the occipital bone has changed considerably during evolution of vertebrates, and it is impossible to affirm the total homology between the different parts of this bone in birds and humans.



Fig. 2.2
A quail-chick chimera observed after Feulgen-Rossenbeck reaction. Neural crest cells from a quail embryo have been grafted into a chick host. Neurons of the autonomous ganglion present a concentrated heterochromatin and are thus derived from the quail (arrows). The environment is derived from the chick (arrowheads)

Fig. 2.3
A quail-chick chimera observed after QCPN immunostaining. Tail bud cells from a quail embryo have been grafted into a chick host. The cells of the donor participate into the formation of the neural tube and the mesoderm (arrows)
An alternative way to study the fate of neural crest cells in mouse embryos has been proposed recently [15]. The bacterial gene Cre (coding for the recombinase enzyme) is driven by the promoter sequence of the Wnt1 gene. This gene is expressed by some cells of the neural tube and by neural crest cells. By crossing this transgenic line with the R26R line, it is possible to generate mice in which the lacZ gene (coding for beta galactosidase) is activated only in cells that express Wnt1. Revealing galactosidase activity allows to follow the fate of neural crest cells. In mouse, neural crest cells give rise to the nasal, the alisphenoid, the squamosal, the frontal, and the center of the interparietal bones. In contrast to the results published for birds, parietal bones do not arise from neural crest cells in the mouse.
Some of the discrepancies between the results gained by these studies can be easily explained. The orbitosphenoid participates in the formation of the vault in birds whereas it only gives rise to the lesser wing of the sphenoid in mammals. The postorbital bone does not exist in mammals. The pleurosphenoid is not homologous to the alisphenoid that is the mammalian component of the vault. The alisphenoid derives from the palato-quadrate (the reptilian epipterygoid bone). This structure develops from neural crest cells of the first branchial arch. The main discrepancies between the two studies correspond to the interparietal and the parietal bones. The interparietal bone gives rise to the part of the occipital bone located above the cerebellar tentorium. We can conclude that in mammals the occipital bone derives from both the mesoderm and the neural crest. The parietal bone is thought to arise from neural crest in birds and from mesoderm in mammals. This could represent a difference between these two species. Furthermore, Jiang et al. [15] doubt about the validity of Couly’s results since the histological section Couly showed to prove the neural crest origin of the avian parietal bone does not pass through that bone.
2.1.4 The Genetic Lecture of the Development
The discovery of genes encoding the identity of a region has been gained from embryonic studies on Drosophila melanogaster. From the late nineteenth century, William Bateson observed transformations of a segment of invertebrate in another segment. He proposed the term “homeotic transformation” to account for this phenomenon. These transformations are particularly well known in Drosophila, a model organism in genetics used as early as the early twentieth century. Thus, a Drosophila wearing legs instead of antennae is called Antennapedia. Many other types of such transformations have been described (e.g., a Drosophila having eight legs instead of six, a Drosophila bearing four wings instead of two). The genetic study obtained by crossing these animals showed that only one gene is mutated. Homeotic transformation is actually a homeotic mutation and the causative gene is responsible for segmental identity. With the advent of molecular biology, genes responsible for homeotic mutations have been cloned. These genes encode transcription factors having a binding domain comprising three DNA helixes. This domain was naturally called homeodomain by reference to the term homeotic. The region of DNA that encodes the homeodomain is called the homeobox. These genes are grouped into clusters in the DNA. Homologous genes to the drosophilian ones were found in all vertebrates. In these species, there are four clusters (A, B, C, and D) carried by different chromosomes. Each cluster contains up to 13 genes (rated from 1 for the gene which is located on the more 5′ extremity of the cluster to 13 for the most 3′). Each gene is defined by the term Hox (for Homeobox) a letter and a number. Genes whose location is the 5′ extremity of the cluster are expressed more rostrally whereas those that lie in the 3′ extremity are expressed more caudally. This property was described by Ed Lewis as the law of spatial colinearity.
This chapter has gained more and more importance nowadays. This could be explained by both the development of molecular biology and the development of human genetics. In terms of genetic regulations of morphogenesis, the vault of the skull can be divided into two parts: the supraoccipital bone and the rest of the vault.
2.1.4.1 Hox d4, a Key Gene for Vertebral Identity
The paraxial mesoderm of vertebrates is organized according to the rostrocaudal axis. The most rostral part of this domain represents the cephalic mesoderm (Fig. 2.1). All the rest of the paraxial mesoderm is segmented into somites that represent metameric units extending to the tip of the embryonic tail. The four first somites are called occipital somites since they contribute to the formation of the occipital bone [13, 14]. The fifth somite is the first cervical somite that participates into the formation of the first cervical vertebra.
Hox d4 is normally expressed from the first cervical vertebra caudalwards. If one forces the expression of Hox d4 in more rostral mesoderm, both the supraoccipital and the exooccipital bones are transformed into occipital vertebrae [16]. This experiment indicates that the sole expression of Hox d4 in the paraxial mesoderm is sufficient to induce the identity of a vertebra and to prevent the formation of the supraoccipital bone. It is important to note that the rest of the vault is normal in this experiment.
2.1.4.2 Mhox (Prx–1) Gene, a Key Regulator for the Formation of the Supraoccipital Bone
The Mhox gene codes for a transcription factor. The knockout of this gene leads to a total absence of the supraoccipital bone while the rest of the vault is normally formed [17]. It is interesting to note that the interparietal bone is not affected by this mutation. This result shows that these two bones, which eventually fuse to form the contribution of the occipital bone to the vault, are not regulated by the same genes. Furthermore, other facial bones are involved by this knockout showing that the Mhox gene is not specific for the supraoccipital bone but participates into other patterning programs affecting the development of different bones.
2.2 The Meninges Are Responsible for the Proper Development of the Posterior Fossa Structures
2.2.1 Histological Description of the Meninges in Humans
The meninges form a wrap that surrounds the central nervous system (see [18] for a review). They consist of the dura mater (or pachymeninge) and a complex called arachnoid-pia mater (or leptomeninges). The dura is formed by collagen fibers grouped into dense bundles and fibroblasts. However, one can notice a heterogeneity within this pachymeninge: indeed, the innermost layers are composed of flattened and jointed cells (neurothelium or subdural mesothelium). Neurothelium is limited to the inner side by a basement membrane that separates it from the arachnoid. Neurothelium cells were also called dural border cells.
The arachnoid may be subdivided into two distinct layers. The outermost layer is composed by the arachnoid border cells (flat cells). These cells are joined by both gap junctions and desmosomes. These junctional devices reflect the role of barrier played by the external arachnoid. The innermost layer of the arachnoid consists in trabeculae that unit this layer to the pia mater. Intertrabecular domains form the subarachnoid space in which the CSF.
The pia mater is composed of a single cell layer in rodents and one to three layers in humans. Junctions that bind cells of the pia mater are the same as those that unite the arachnoid cells.
There are no histological criteria for distinguishing arachnoid trabecular cells from pial cells. Thus, modern histologists speak of leptomeningeal cells to define them.
Several authors have described a discontinuous pia mater in human. These postmortem studies are not correct and this discontinuity is actually an artifact. Finally, between the pia mater and the brain surface, lies a space (called subpial space) that contains a few collagen fibers.
2.2.2 Embryonic Origin of the Meninges
The embryological origin of the meninges is a topic that is widely established and demonstrated by developmental biologists. Thus, the old theory, now obsolete, which means that leptomeninges have a different origin as the pachymeninges and going back to the late 1920s is outdated. However, this theory remains in certain articles [19]. It is now well established that this information is obsolete particularly in higher vertebrates.
The origin of the meninges has been reestablished first in birds using the technique described by Nicole Le Douarin. The application of this technique showed that all cells of the spinal meninges are derived from somites [20, 21] and not the neural tube [22]. The cephalic mesoderm that is the rostral extension of the paraxial mesoderm (Fig. 2.1) gives rise to the meninges of the brain stem and cerebellum. In contrast, meningeal forebrain cells derive from neural crest and are generated by the cephalic mesectoderm (that is to say the mesoderm that is formed from the ectoderm) [23]. This origin in mammals has been confirmed by the work of Jiang et al. [15].
In conclusion, cells of the meninges (both pachymeninges and leptomeninges) sitting in the posterior fossa are derived from the cephalic mesoderm, a region of the paraxial mesoderm.
2.2.3 Developmental Interactions Assumed by the Meninges
The meninges are often seen as supporting tissues whose developmental role is relatively low. Even if this assertion is widespread in the medical literature, it is false. First, the meninges induce the formation of the superficial glia limitans. Indeed, at the interface between neural tissue and meningeal region, develops a highly elaborate structure consisting of a variety of elements. The outermost region of the nervous system is composed of astrocytic feet that are joined together by gap junctions and form the glia limitans. This structure is covered with a basal lamina that is composed of laminin and collagen IV [24].
To highlight and demonstrate the role played by the cells of the meninges on the formation of the interface between the central nervous system and the outside, it is possible to selectively destroy the meningeal cells by injecting 6 OH dopamine in the subarachnoid space in the rodent newborn (see [25] for a review). In this case, the destruction of meningeal cells leads to a disappearance of the basement membrane and glia limitans [25].
The situation became even more complex now. These latest features are only the more fascinating because they introduce the problem of regionalization of cells according to the anteroposterior axis. Most medical authors consider that the meninges form a homogeneous tissue along this body axis. However, a new study shatters such insurance. Stephen Goff’s group in La Jolla [26] generated a mouse that has both a conditional deletion of the Abl gene expressed only in neuronal and glial cells on a genetic background deficient in Arg. Abl and Arg are genes that code for two non-receptor tyrosine kinases that are involved in the transduction of the signal from the cell surface to the cytoskeleton. They observed that in this case, there is a severe cerebellar dysplasia with loss of basement membrane, an aspect which is reminiscent of that observed after local destruction of the meninges. Cautious analysis of this mutant shows that the defect is not related to impairment of cerebellar granule cells but to an intrinsic defect of the radial glia and Bergmann cells of the cerebellum. Besides the cognitive interest of such an outcome, it seems important to indicate that this defect only affects the cerebellum and that such topographic specificity could explain the existence of malformative lesions involving only parts of neuraxis in humans or proliferative lesions specifically related to a particular anatomic location.
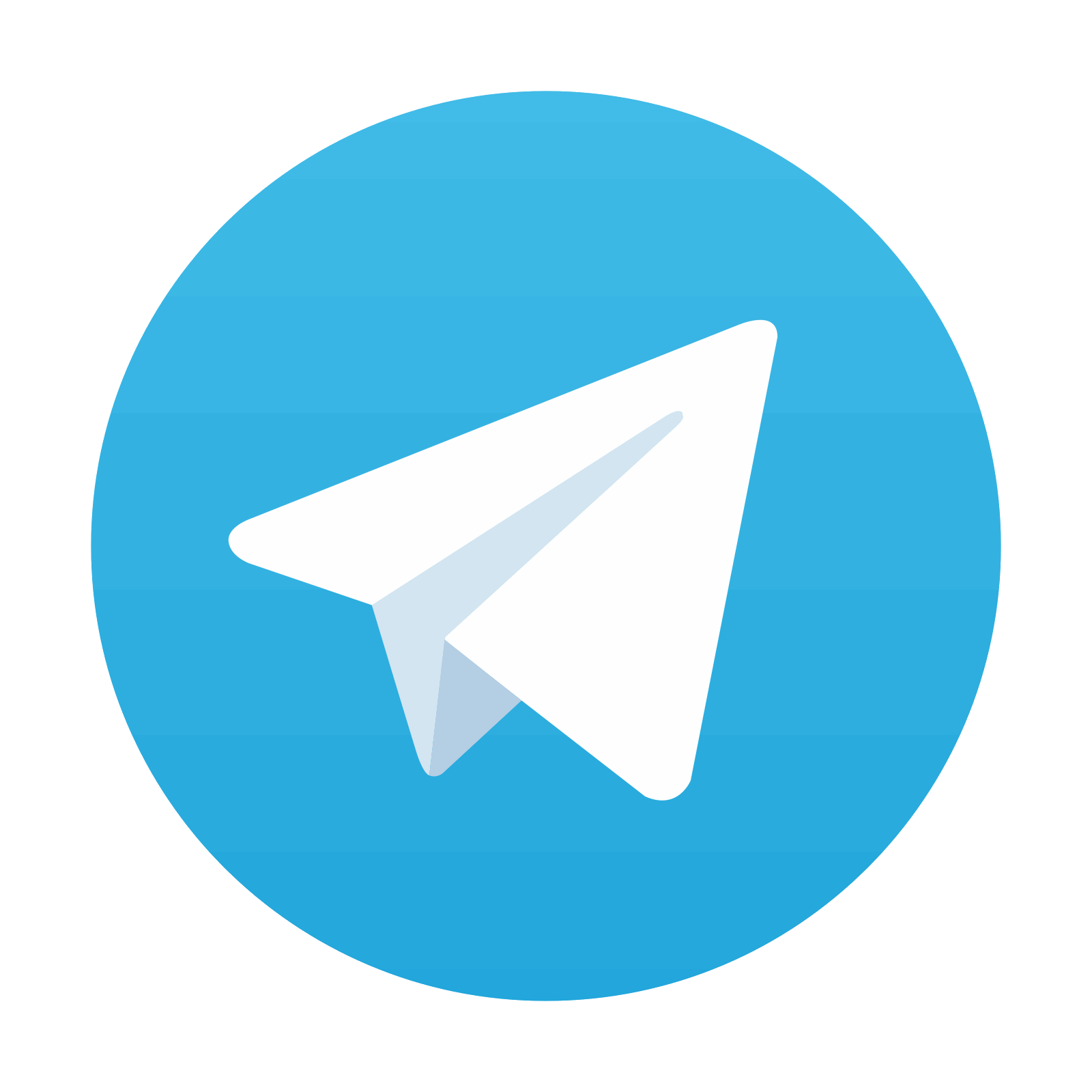
Stay updated, free articles. Join our Telegram channel

Full access? Get Clinical Tree
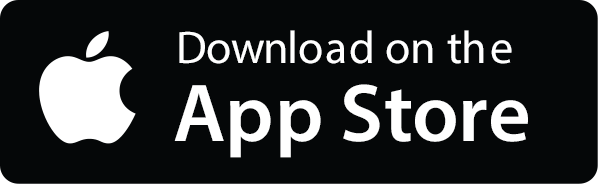
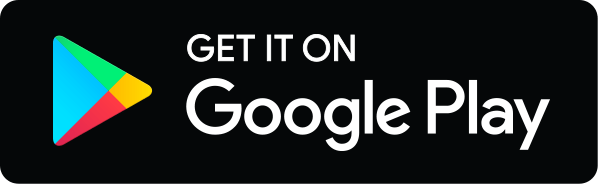