Digital EEG
Malcolm Yeh
Introduction
Traditional electroencephalogram (EEG) recordings are typically used for medical diagnosis and neurobiological research. One EEG channel, commonly recorded from a surface electrode attached to the scalp, is a graphical representation of the voltage as it varies with time. When multiple electrodes are used, more than one location on the scalp can be monitored simultaneously to produce a multiple channel EEG recording. In essence, the EEG record is a picture of the electrical activity of the brain. The amplitude of the wave represents the amount of voltage and can be displayed on a paper recorder using a galvanometer-driven pen-writing system or a digital video screen driven by a computer.
Since the invention of the EEG machine in 1929 by Hans Berger, the basic components of an EEG system have remained the same. That includes electrodes (the actual voltage sensors), amplifiers, and output devices. Over the years, there has been continual improvement of each component with the latest improvement being on the output device.
The classical paper-recorded EEG for clinical diagnostic purposes remained in common clinical use for about 45 years before advances in computer technology made it more economical to store EEG recordings digitally. Digital recording technology was first applied commercially in the music industry in the 1960s as a means of reproducing musical data without loss of quality in the recording during the duplication. In the 1970s, clinical neurophysiology began recording digital data in evoked potential recordings. Because evoked potentials are short in duration and resolved with averaging techniques, storage of small static data files was not a problem. However, storage for a continuous stream of data from an EEG recording only became possible when two advancements occurred. The first occurred when computer processors became fast enough to capture the large amount of streaming data from a multichannel EEG system. The second advancement occurred in about 1990, when long-term computer storage media dropped low enough in price to allow economical storage of large amounts of data. At that time, digital EEG systems quickly came on the market to compete with the analog (paper) recording systems. Digital EEG proved to have many advantages including (i) filing and storage of the recording in a much smaller space (i.e., tape, CD, DVD, flash drives, and remote servers), (ii) the ability to reformat the data postrecording with regard to the viewing montages, filter adjustments, adjustments in sensitivity (vertical scaling), and time base settings (horizontal scaling), (iii) more accurate measurements of frequency and timing in regard to phase relationships between channels, and (iv) the ability to quantify EEG characteristics numerically with statistical tools such as power spectrum analysis and topographic voltage maps.1,2 Digitized EEG data certainly provided many more advantages and were a major advancement over the analog recordings.3
However, there were a few pitfalls for the early commercial EEG systems. When the first generation of commercial digital EEG systems appeared in the early 1990s, the recordings were merely analog EEG systems with a low-resolution display attached to magnetic storage media. This first generation of digital EEG recorders offered digital storage as the main advantage. However, reading the EEG could not be done instantaneously as a paper recording could.4 In addition, the technologist’s job of monitoring and making adjustments to optimize the recording was a slower and more complicated process because adjustments were made through tedious keyboard commands. As a result, many technologists at that time found it easier to perform a paper recording. Because of the ease in storing large amounts of data, there is created the problem of accurately analyzing large amounts of continuous EEG data in a timely manner making EEG bedside interpretation impossible. As a result, quantitative EEG (qEEG) analysis has seen the invention of numerous tools to improve the efficiency and reduce the burden of analyzing massive amounts of data. Since the data can be processed mathematically by any number of algorithms, a large array of techniques have come into being to help visualized continuous EEG in a more compact form. Some examples of these techniques include (i) comparing for asymmetries in various amplitudes and frequencies over analogous areas of brain, (ii) assessing for changes in frequencies in the time domain to identify state changes or seizure activity, and (iii) recognizing patterns of potentially epileptiform discharges. Many of these techniques have been included in the EEG reading software, though validation is still needed when more subtle findings are being sought.5
ANALOG EEG (PAPER) RECORDERS
The classic analog pen-writing EEG recorders required dividing the technologist’s attention between the patient and to the many parts of the EEG equipment. This would include intermittently
monitoring the amplitude of the waveforms for pen blocking or extremely low amplitudes that would require adjustments of the sensitivity setting of the amplifiers. Messy mechanical ink pens would occasionally malfunction and require maintenance while the recording was in progress. Maintaining accurate pen alignment was tedious but was required to maintain the time phase relationships between channels. From time to time, there would be noise in the recording that would obscure the EEG, and optimizing the recording of a desired signal would require further adjustments of filters or paper-speed. Finally, observation and notation of the patient’s behavior during the recording were performed by writing directly on the moving paper as it was rolling past the moving EEG pens.
monitoring the amplitude of the waveforms for pen blocking or extremely low amplitudes that would require adjustments of the sensitivity setting of the amplifiers. Messy mechanical ink pens would occasionally malfunction and require maintenance while the recording was in progress. Maintaining accurate pen alignment was tedious but was required to maintain the time phase relationships between channels. From time to time, there would be noise in the recording that would obscure the EEG, and optimizing the recording of a desired signal would require further adjustments of filters or paper-speed. Finally, observation and notation of the patient’s behavior during the recording were performed by writing directly on the moving paper as it was rolling past the moving EEG pens.
DIGITAL EEG RECORDERS
Second-generation digital EEG machines came into the market in the late 1990s, with the main improvement being better display monitors while streamlining the technical aspects of the recording process. From the technologist’s point of view, there were fewer steps and fewer factors that had to be addressed in order to complete an EEG recording. With the digitally recorded EEG, the ink pens have been replaced with a computer display that requires no maintenance. The amplitude range of digital EEG is much larger in comparison to paper-generated records. In effect, this eliminates the need to periodically adjust the gain of the amplifiers. Monitoring and adjusting the amplitude of the signal has become an option for the technologist rather than a necessity. Because digital frequency filters can be applied when the recording is reviewed, applying frequency filters in real time is used to monitor the quality of the recording and to aid the technologist in viewing abnormalities. Timing in regard to phase relationships between channels is now automatic and no longer requires alignment in comparison to the pen-writing system. As a result of these conveniences, more time can be spent monitoring and documenting the patient’s behavior and symptoms.
A schematic of a digital EEG recording system in comparison to the classic pen-writing system is shown in Figure 4-1.
Components of the Digital EEG System
SENSORS
The first component in the recording of biological signals is the sensor, which in the case of EEG would be the scalp electrodes. The electrode is an extremely important element in determining the quality of the recorded signal. It is the technologist’s responsibility to be sure that the recorded signal at the sensor level is adequate; otherwise, junk signals into the system will only generate junk signals out. This means being sure that the electrode is applied in the correct location and that the electrical contact is adequate (impedance <5 kΩ) in addition to keeping external electrical noise to a minimum.
The recorded voltage from the scalp electrode is a continuously varying signal. A signal that is continuous without breaks or discontinuities is, by definition, an analog signal. Nature surrounds us with analog signals. Examples include sound wave amplitudes, intensities of light, and magnitudes of weights to name just a few of the measurable analog properties that can be measured as a continuous analog signal. To recapitulate, the voltage recorded from a scalp electrode is an example of a continuous analog signal that will be compared to a digitized signal later in our discussion.
AMPLIFIERS
The analog amplifier is the second component in the recording pathway. The continuous analog signal is fed into the amplifier that typically boosts the signal so that it can be further processed in the recording system. For a pen-writing system, the signal voltage would be converted to pen displacement that would produce a paper recording.
DATA STORAGE AND PROCESSING
The third component in a digital EEG system has the amplifier sending the amplified signal to a special electrical circuit known as an ADC (analog-to-digital converter) circuit. This is to be contrasted to the analog EEG system, where the third component would be the pen-writing system. The ADC circuit is also called a digitizer because its function is to transform an analog measurement into a number. This circuit is a specialized digital voltmeter with a rapid response time that can make a voltage measurement almost instantaneously. It measures the amplified signal voltage and converts it into a number that is then sent to a computer to process. It is this step that changes the analog signal to a digital signal and allows the signal to be represented as a number that machines can easily process and store on a variety of mediums (i.e., storage tape, CD, DVD).
Analog signals, being continuous by nature, have a continuously changing value over time. However, digitized signals are only a sampling of the original analog signal since only a finite number of values can be stored and processed (Fig. 4-2). When digital EEG recordings were in their infancy, the slower computer processors could not match the quality of the analog recordings because sampling rates were too low. However, second-generation digital EEG systems allow much faster sampling rates and have easily matched the resolution of analog EEG. Typically, computer processors in current digital EEG systems are fast enough that digital data can be stored as well as providing a simultaneous data display to monitor the quality of the recording. One nice aspect of storing data in digitized form is that there is no degradation of the quality of the data no matter how many times the data are copied. This is certainly an advantage over analog recordings, where the information degrades each time it is duplicated.
Factors and Limitations of Digitized Data
Digital recordings have certainly made EEG recording more efficient both in the use of the technologist’s time and in archiving
records. However, because the analog data are digitized, there are additional factors and limitations that ultimately affect the quality and interpretation of the recording. The technologist will need to be aware of the frequency and amplitude of the signal of interest in comparison to the resolution of the digital record in terms of amplitude resolution and time resolution. Amplitude and time factors will determine the types of EEG signals that could be recorded. Some of the pitfalls in recording and interpreting digitized data would include the introduction of virtual signals that occur in the reconstruction process of the original analog signal. These virtual signals are called alias signals (or aliasing), which are easily seen in cyclical recorded signals. An example of this is in the recording of a regular sine wave signal.
records. However, because the analog data are digitized, there are additional factors and limitations that ultimately affect the quality and interpretation of the recording. The technologist will need to be aware of the frequency and amplitude of the signal of interest in comparison to the resolution of the digital record in terms of amplitude resolution and time resolution. Amplitude and time factors will determine the types of EEG signals that could be recorded. Some of the pitfalls in recording and interpreting digitized data would include the introduction of virtual signals that occur in the reconstruction process of the original analog signal. These virtual signals are called alias signals (or aliasing), which are easily seen in cyclical recorded signals. An example of this is in the recording of a regular sine wave signal.
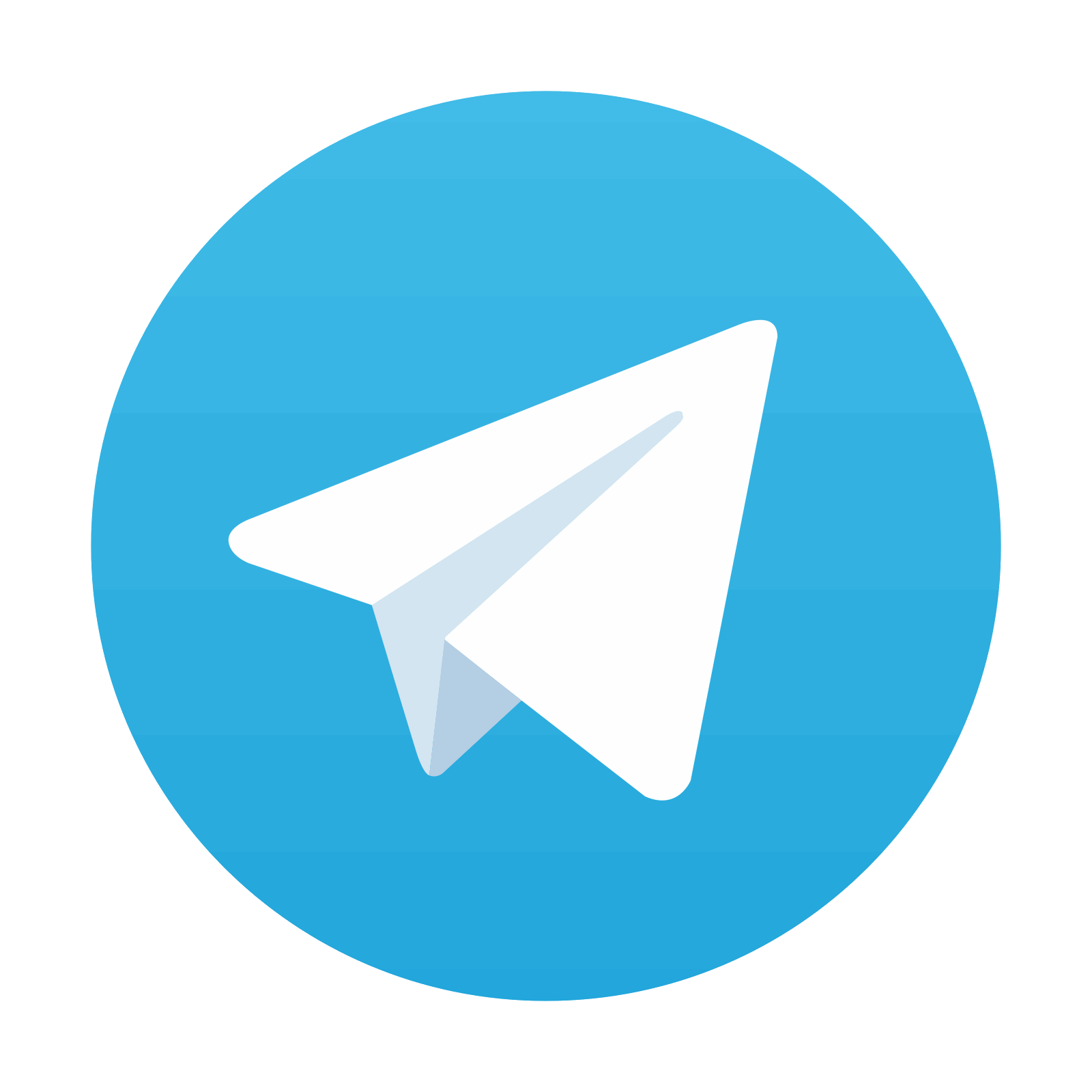
Stay updated, free articles. Join our Telegram channel

Full access? Get Clinical Tree
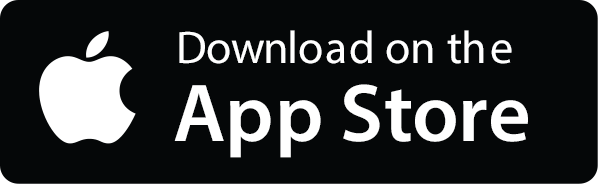
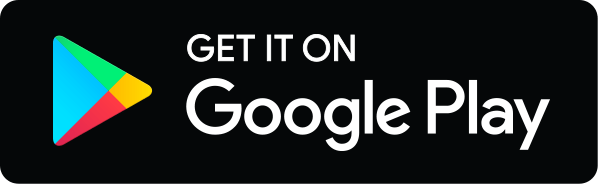