Fig. 1
Optogenetic stimulation of Hcrt neurons and their downstream targets during NREM result in wakefulness. a Fluorescence micrographs of neurones in the lateral hypothalamus expressing EGFP (green), ChR2-mCherry (red) and merge showing co expression. b Blue-light pulse trains (15 ms per pulse, 20 Hz) evoked reliable firing of ChR2 transfected Hcrt neurons in vitro. Evoked action potentials show high temporal precision to stimulation, despite the presence of basal spontaneous activity. c Time line for the behavioural state dependant simulation of Hcrt neurons in vivo. Stimulation is performed 10 s after the onset of behavioural state and lasts for 10 s. The latency to state transition is recorded. d Schematic showing mouse implanted with EEG/EMG electrodes as well as fibre optics for optical stimulation. e Latencies to wake transitions following optogenetic stimulation of Hcrt neurons during NREM. Data analysis is based on an average of 15 stimulations per frequency per mouse. f Optogenetic stimulation of LC neurons at any frequency reduces the time to wakening from NREM. g Stimulation of the LC increases membrane excitability and enhances Hcrt-mediated sleep-to-wake transitions. [Figure b and e adapted from Adamantidis et al. (2007). Figure f adapted from Carter et al. (2010), Figure g from Carter et al. (2012)] (Color figure online)
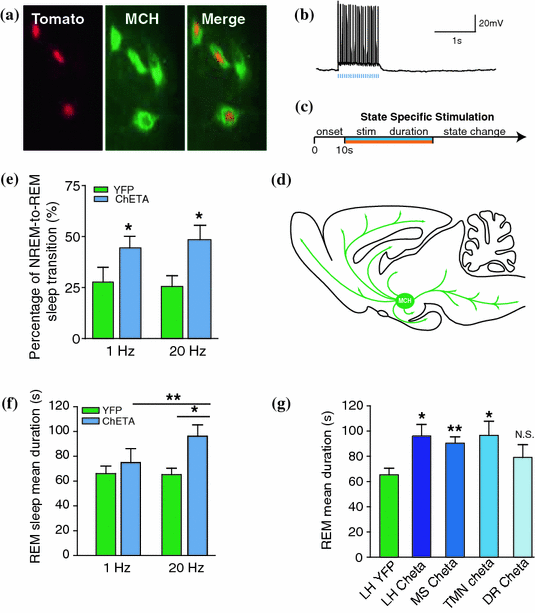
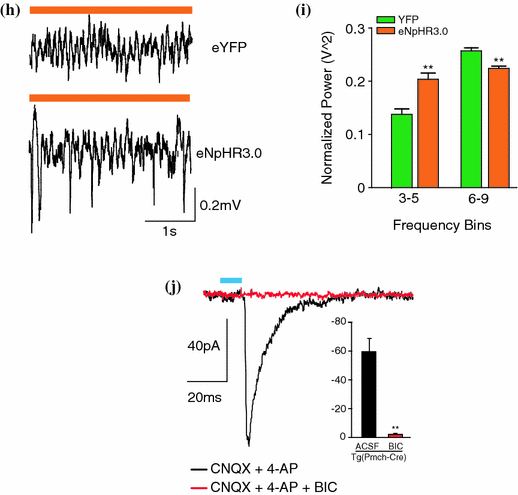
Fig. 2
Optogenetic activation of MCH neurons augments REM sleep. a Fluorescence micrograph of MCH neurones in the lateral hypothalamus showing expression of td-Tomato (red), MCH (green) and merge to show co-expression. b Blue-light pulse trains (5 ms per pulse, 20 Hz) evoked reliable firing of ChETA transfected MCH neurons in vitro. Evoked action potentials show high temporal precision to stimulation, despite the presence of basal spontaneous activity. c Time line for the behavioural state dependant simulation of MCH neurons in vivo. Neurones are either stimulated (blue) or inhibited (orange) until change of behavioural state occurs. d Schematic of projections of MCH neurones throughout the brain. e Percentage of successful NREM-to-REM sleep transitions following optogenetic stimulation during NREM. Data are shown as a percentage of the total number of NREM-to-REM sleep transitions on the total number of stimulations during NREM sleep. f Mean duration of REM sleep episodes following optogenetic stimulation at 1–20 Hz of control and ChETA-EYFP mice. Data analysis is based on an average of at least 15 stimulations per frequency and per mouse during REM sleep episodes. g Mean REM sleep duration of mice stimulated at 20 Hz during REM sleep in the TMN, dorsal raphe or medial septum. The results of lateral hypothalamus stimulation are reported for comparison. h Representative EEG traces during optogenetic silencing of MCH neurones with eNpHr3.0 and eYFP. i Quantification of slow theta oscillations during optogenetic silencing of EYFP and eNpHR3.0-YFP transfected MCH neurons in vivo. j Evoked IPSCs (black) in TMN neurons expressing ChETA-EYFP are blocked with bicuculline. The amplitudes of the currents are shown in the inset. [Figures adapted from Jego et al. (2013)] (Color figure online)
Recent studies suggest a sleep-promoting role for the MCH system. A large number of c-Fos+ cells (marker of neuronal activity) during a sleep rebound induced by total or a selective REM sleep deprivation were found to be immunoreactive for MCH (Modirrousta et al. 2005; Verret et al. 2003). While 60 % of the MCH-containing neurons were c-Fos+ after REM sleep deprivation, Hcrt neurons were not (Modirrousta et al. 2005; Verret et al. 2003). In addition, intracerebroventricular (icv) infusion of MCH in rats causes hypersomnia by dose-dependent increases in SWS (+70 %) and REM sleep (+200 %) (Verret et al. 2003), whereas a MCH-R1 antagonist has the opposite effect. Collectively, these functional data suggested that activation of MCH neurons may promote sleep by inhibiting arousal centers of the brain, whereas the Hcrt system induces arousal by activating them. However, the precise role the MCH system in sleep remains unclear (Adamantidis et al. 2008; Jego and Adamantidis 2013).
In agreement with this hypothesis, we recently demonstrated that acute optogenetic activation of MCH neurons (ChETA, SSFO) at the onset of REM sleep extended the duration of REM, but not non-REM sleep episodes (Jego et al. 2013). In contrast, their acute silencing (eNpHR3.0, ArchT) reduced the frequency and amplitude of hippocampal theta rhythm, without affecting REM sleep duration. In vitro activation of MCH neuron terminals induced GABAA-mediated inhibitory post-synaptic currents (IPSCs) in wake-promoting histaminergic neurons of the tuberomammillary nucleus (TMN), whilst in vivo activation of MCH neuron terminals in TMN or medial septum also extend the duration of REM sleep episodes.
Collectively, these results demonstrated that selective activation of LHMCH neurons promotes and maintains REM sleep, possibly through inhibition of arousal circuits in the mammalian brain.
7 Perspectives
Optogenetics is proving to be a valuable tool in dissecting the components of the sleep-cycle at the hypothalamic level. It has in some instances confirmed previous less specific ablation, pharmacological and genetic studies and also given new insights into the neural circuits involved in the control of brain states during sleep and wakefulness. Different sleep-wake states are characterised by markedly different EEG signatures due to changes in cortical synchronicity. Since long-range cortical networks are regulated by the thalamus, future optogenetic experiments should focus in elucidating circuitry downstream from the hypothalamus, in order to form a unifying theory of brain control of sleep, as well as consciousness.
Acknowledgments
We thank the Tidis Lab members for their technical help and comments. A.A. is supported by the Human Frontier Science Program, Canadian Institute for Health Research (CIHR), the University of Bern, the Inselspital and the Swiss National Funds.
References
Adamantidis A, Carter MC, de Lecea L (2010) Optogenetic deconstruction of sleep-wake circuitry in the brain. Front Mol Neurosci 2:31PubMedCentralCrossRefPubMed
Anaclet C et al (2012) Identification and characterization of a sleep-active cell group in the rostral medullary brainstem. J Neurosci 32:17970–17976PubMedCentralCrossRefPubMed
Appelbaum L et al (2009) Sleep-wake regulation and hypocretin-melatonin interaction in zebrafish. Proc Natl Acad Sci USA 106:21942–21947PubMedCentralCrossRefPubMed
Aston-Jones G, Bloom FE (1981) Activity of norepinephrine-containing locus coeruleus neurons in behaving rats anticipates fluctuations in the sleep-waking cycle. J Neurosci 1:876–886PubMed
Blanco-Centurion C, Gerashchenko D, Shiromani PJ (2007) Effects of saporin-induced lesions of three arousal populations on daily levels of sleep and wake. J Neurosci 27:14041–14048PubMedCentralCrossRefPubMed
Brisbare-Roch C et al (2007) Promotion of sleep by targeting the orexin system in rats, dogs and humans. Nat Med 13:150–155CrossRefPubMed
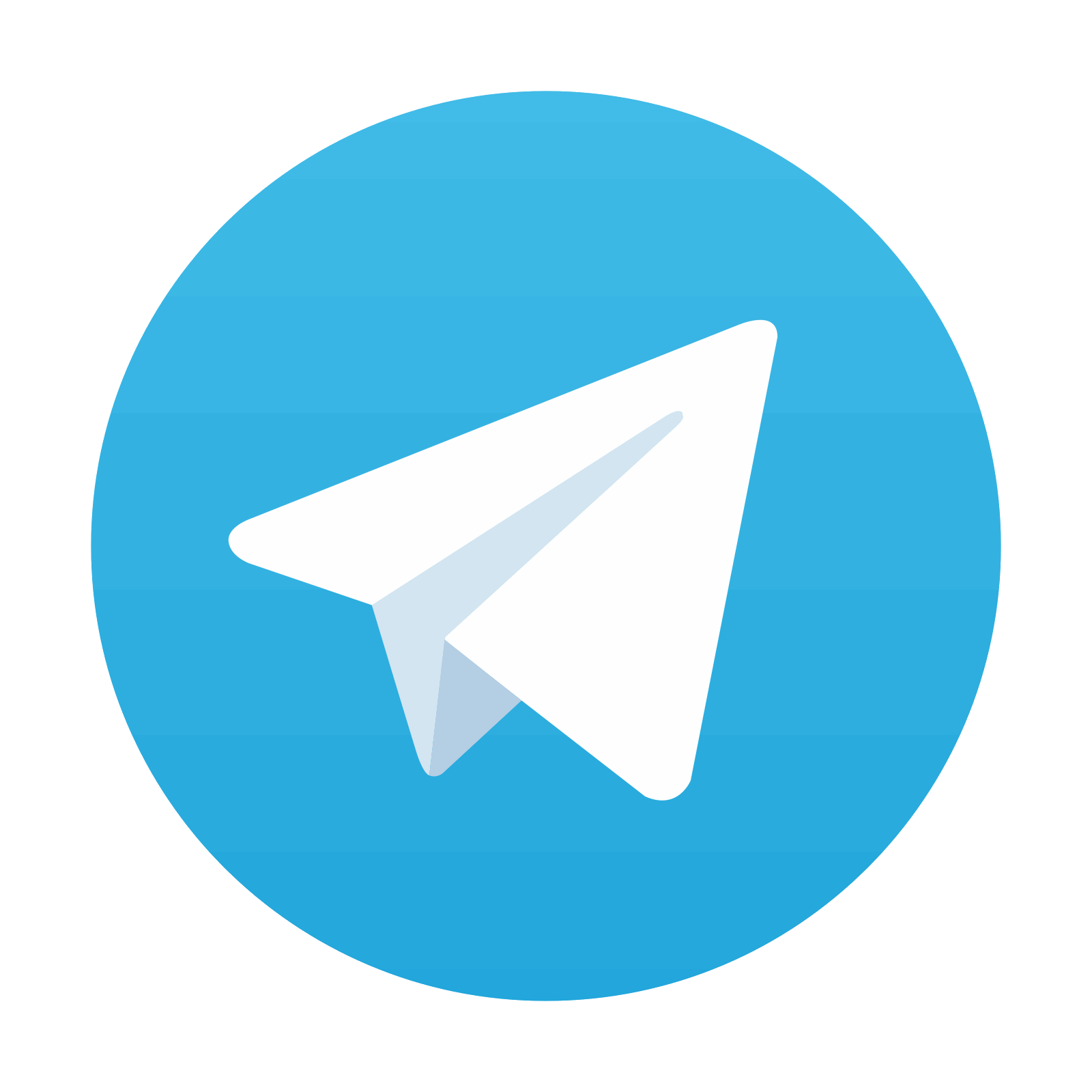
Stay updated, free articles. Join our Telegram channel

Full access? Get Clinical Tree
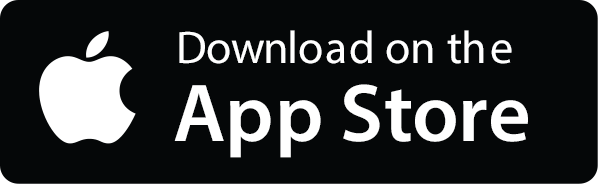
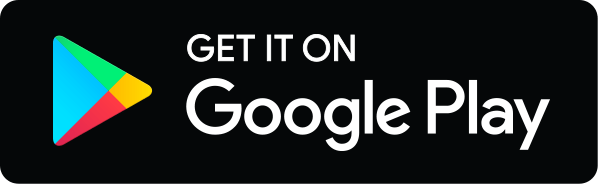