Fig. 1.1
Conceptual relationship between the acupoint, organ, and brain. The acupuncture effect can be evaluated via functional magnetic resonance imaging (fMRI). TCM traditional Chinese medicine
1.2 Principles of fMRI
Functional MRI is a noninvasive and relatively safe technique for the measurement and mapping of brain activity. fMRI is widely applied for the study of the brain and functional disruptions in the brain during pathological disease states.
1.2.1 MRI Contrast
MRI image acquisition relies on the use of a contrast agent. Different tissue characteristics can be visualized with the use of radiofrequency or gradient pulses and variations in the timing of image acquisition. The utility of contrast use in MRI derives from the decay of nuclear magnetization in a process called relaxation; the evaluation of differences in signal decay is specified by a given sequence “relaxation time .” There are three relaxation times that are of primary interest in MRI: T1, T2, and T2*. The T1 constant measures longitudinal relaxation time in the direction of the static magnetic field (B0). The T2 constant measures the transverse relaxation time in the plane perpendicular to the B0 field and notably is affected by molecular interactions and variations in B0. The T2 relaxation process is also affected by the combined time constant T2* (T2 star). T2* is the most relevant relaxation time for the study of the brain using contrast fMRI images (Matthews and Jezzard 2004).
1.2.1.1 Blood-Oxygen-Level-Dependent (BOLD) Contrast
BOLD contrast is employed in most fMRI studies. This method is based on the concept that neural activity affects the relative concentrations of oxygenated and deoxygenated hemoglobin in the local blood supply. Deoxyhemoglobin (dHb) is paramagnetic and therefore alters the MRI signal, while oxyhemoglobin (HbO2) is diamagnetic and has no effect on the MRI signal. To this end, increases in dHb decrease the T2* constant, such that BOLD contrast represents the difference in T2* signal between HbO2 and dHb (Fig. 1.2) (Yassa 2005; Chen and Glover 2015).


Fig. 1.2
Changes of HbO2 and dHb concentrations between the baseline state (a) and the activated state (b) (Reprint with permission from Chen and Glover 2015)
The principle underlying BOLD signal changes in response to brain activity is as follows. After stimulation, neural activation induces the absorption of oxygen from the local blood supply. In theory, this increase in paramagnetic dHb leads to the enhancement of field non-homogeneities and the reduction of BOLD signal. However, increased dHb is also accompanied by a surge in cerebral blood flow to increase the local supply of oxygenated blood and compensate for local deoxygenation. In this case, both the cerebral blood volume and hemoglobin oxygenation increase, and susceptibility-related dephasing decreases, which increases T2* signal and in turn enhances BOLD contrast (Yassa 2005).
1.2.1.2 Neural Basis of BOLD Signal and Neurovascular Coupling
The neuronal processes underlying changes in BOLD signal are related to synaptic inputs in active regions rather than the output level of neurons receiving synaptic inputs. This means that while BOLD signal on fMRI can reflect the synaptic activity that drives neuronal network communication, the information content of the activity is unknown.
Activity in the brain is tightly coupled to local blood flow. To this end, it is known that changes in the metabolic load are directly coupled to synaptic activation. One mechanism coupling vascular responses to synaptic activation involves the synaptic release of glutamate and subsequent Ca2+ alterations in neighboring astrocytes, which lead to the release of vasodilatory molecules. Accordingly, fMRI signals can be applied to explore functional responses in the brain using BOLD signals as a surrogate of synaptic activity (Seiji and Yul-Wan 2007).
1.3 Acupoint Specificity on fMRI
Acupoints are designated according to their functional and locational specificity. The concept of functional specificity purports that stimulation of a given acupoint causes corresponding changes in the BOLD signal in specific brain areas based on the function of the acupoint. Alternatively, locational specificity compares brain responses between a given acupoint and a sham acupoint (non-meridian or non-acupoint locations) or two different acupoints.
1.3.1 Acupoint Functional Specificity on fMRI
The earliest fMRI studies of acupuncture were focused on acupoint functional specificity and accordingly attempted to correlate acupoint stimulation with the activation of specific brain regions (Cho et al. 1998; Gareus et al. 2002; Siedentopf et al. 2002; Wu et al. 2002; Li et al. 2003b). The classic BLOCK experimental model original from psychology was used in these studies (Fig. 1.3). However, the conclusions of these studies have been inconsistent.


Fig. 1.3
The experimental model of early acupuncture fMRI studies. “A” is needle twirling state and “B” is needle retaining state (Reprint with permission from Chen and Glover 2015)
Vision-related acupoints are the most well-studied acupoints in functional specificity studies. Cho and colleagues first applied fMRI to evaluate BOLD signal changes in the brain evoked by the stimulation of vision-related acupoints BL67–BL60 (VA1–VA8) (Cho et al. 1998). Participants were subjected to conventional checkerboard 8-Hz light flash visual stimulation or acupoints stimulation according to the same time-course paradigm, and BOLD signal in the visual cortex was compared between the two stimulation methods. The results showed that visual acupoint stimulation produced cortical activation patterns very similar to those produced by visual light stimulation and that stimulation of BL67 in particular produced notable activation of the visual cortex. In a subsequent study, laser acupuncture and electroacupuncture (EA) were used to stimulate BL67 (Siedentopf et al. 2002; Li et al. 2003b), and the results confirmed the ability of acupoint stimulation to modulate the activity of visual brain areas.
In contrast, another research has refuted the ability of acupoint stimulation to produce activation in vision-related brain areas. Gareus and colleagues studied another vision-related acupoint, GB37, and compared acupuncture stimulation with visual stimulation using fMRI (Gareus et al. 2002). The recruited subjects were randomly divided into three groups and separately received visual stimulation and acupuncture stimulation without twisting the needle to avoid deqi, visual stimulation and acupuncture stimulation with deqi, or only acupuncture stimulation with deqi. The results demonstrated that activation of the visual cortex was only observed in subjects that received visual stimulation, whereas acupuncture stimulation had little impact on changes in BOLD signal in the visual cortex. Moreover, no activation of the visual cortex was detected in subjects that received acupuncture stimulation only (Fig. 1.4). Wu et al. also concluded that activation of the visual cortex was not a specific effect of vision-related acupoints (Wu et al. 2002). Soon after the publication of this study in 2006, Cho and colleagues retracted their functional specificity study on vision-related acupoint stimulation (Cho et al. 2006). In spite of this, the block design model was retained as a standard stimulation paradigm for fMRI studies of acupuncture.


Fig. 1.4
Surface-rendered brain activation evoked by minimal, mock, sham, and real electroacupuncture (EA) stimulation (Reprint with permission from Wu et al. 2002). Minimal EA (a, b), sham EA (c), and real EA (d) all activated the medial occipital cortex (visual cortex). Real EA showed activations in the right medial occipital cortex, primary somatosensory-motor cortex, and hypothalamus and bilateral prefrontal cortex when comparing with sham EA (e)
An fMRI study was also used to evaluate brain responses to language-related acupoint stimulation (Li et al. 2003a). The results showed that, whereas word generation produced significant activation in the bilateral inferior frontal gyrus (IFG) and left superior temporal gyrus (STG) , stimulation of two language-related acupoints (SJ8 and Du15) resulted in significant activation of the right IFG and bilateral STG.
The acupoint GB34 , which is known to be a useful stimulation point for facilitating motor recovery after stroke, was investigated to determine its relationship with sensorimotor area activation (Jeun et al. 2005). Block design acupuncture stimulation at GB34 produced associated changes in the BOLD signal in bilateral sensorimotor areas, leading the authors to suggest that acupuncture-induced activation of sensorimotor areas may serve as a basis for the motor effects of GB34 stimulation.
Acupuncture has also been reported to increase salivary flow in healthy volunteers and patients with xerostomia (dry mouth) (Dawidson et al. 1997; Morganstein 2005). The neural substrates of this effect were explored using fMRI (Deng et al. 2008). LI2 , a point commonly used for the clinical treatment of xerostomia, was selected as a target acupoint, and both BOLD signals and saliva production were measured before and after acupuncture manipulation. Deng and colleagues found that LI2 stimulation primarily produced activation in the bilateral insula and adjacent operculum, and moreover BOLD signal changes were associated with increased saliva production. Accordingly, the efficacy of LI2 stimulation was hypothesized to be related to its ability to produce insular activation.
LI4 is an effective acupoint for the treatment of facial palsy and facial muscle spasms (Xu et al. 2013). In the work by Xu et al., electroacupuncture at L14 produced typical signal deactivation in the bilateral hippocampus, parahippocampal gyrus, amygdala, anterior cingulate cortex (ACC), prefrontal lobe, and occipital lobe. Furthermore, Wang and colleagues found that activation of the precentral gyrus, which represents the movement of orofacial muscles, and cerebellum was related to the therapeutic effects of L14 in facial palsy and facial muscle spasm (Wang et al. 2007). Further studies are necessary to clarify whether these activation patterns represent functionally specific responses to acupoint stimulation or simply correlate with general somatosensory stimulation coupled to acupuncture.
1.3.2 Acupoint Locational Specificity on fMRI
In locational specificity studies, sham acupuncture is usually used as a control to exclude simple placebo effects. Several kinds of sham acupuncture strategies are used in research, including needling at a sham acupoint (Napadow et al. 2009a; Xiong et al. 2012), noninvasive placebo stimulation (e.g., Von Frey filament or Streitberger needle stimulation) at an acupoint site or sham acupoint site (Witt et al. 2005; Brinkhaus et al. 2013), and superficial needling at an acupoint site or sham acupoint site (Huang et al. 2005; Yin et al. 2010). Accordingly, both sham acupoints and sham stimulation have been used in acupuncture fMRI studies.
In 2010, Hui and colleagues hypothesized that acupuncture stimulation could evoke deactivation of the limbic-paralimbic-neocortical network (Hui et al. 2010a, b). This hypothesis was based on the observation that needling at LI4 or ST36 produced prominent deactivation in the nucleus accumbens, amygdala, hippocampus, parahippocampus, hypothalamus, ventral tegmental area, ACC, caudate, temporal pole, and insula, whereas needling produced activation in the somatosensory cortex in subjects who experienced deqi. Additionally, deactivation of the cerebellum was also noted during ST36 stimulation. Given that no decreases in signal were observed in subcortical structures in a superficial tactile stimulation control group, it was concluded that activity of the cerebro-cerebellar and limbic systems evoked by acupuncture needle manipulation might be a typical modulation effect of acupuncture (Fig. 1.5) (Wu et al. 1999; Hui et al. 2000, 2005). In a subsequent study by Fang et al., BOLD signal changes in response to manual acupuncture at LV3 , LV2, and ST44 were investigated, and the results again demonstrated extensive deactivation of the limbic-paralimbic-neocortical network (Fang et al. 2009). Electroacupuncture at GB34, CV4, and CV12 has also been reported to modulate the activity of the limbic system (Wu et al. 2002; Fang et al. 2012). Functional connectivity of limbic-paralimbic-neocortical network was also explored during acupuncture versus tactile stimulation in a 2009 study by Hui and colleagues, and similar results were obtained (Hui et al. 2009). These studies all provided support for the hypothesis of Hui and colleagues that the deactivation of the limbic-paralimbic-neocortical network produced by acupuncture stimulation with deqi sensations might be the fundamental of acupuncture effect on various diseases.


Fig. 1.5
The influence of subjective sensations on fMRI signal changes in the brain during acupuncture (or control stimulation) at ST36 (Reprint with permission from Hui et al. 2005). Regions: 1 frontal pole, 2 subgenual anterior cingulate, 3 ventromedial prefrontal cortex, 4 hypothalamus, 5 posterior cingulate cortex, 6 reticular formation, 7 cerebellar vermis, 8 middle cingulate, and 9 thalamus
Another research has challenged the hypothesis of Hui and colleagues. One review article found that BOLD responses associated with deqi were primarily characterized by activation rather than deactivation (Sun et al. 2013). Indeed, high-quality studies reporting robust BOLD responses to acupuncture stimulation with deqi have mainly highlighted four basic systems: the somatosensory system, motor system, sensory integration system, and special senses (vision, hearing) (Fig. 1.6). The most commonly activated areas in these studies included the secondary somatosensory cortex (SII), insula, primary somatosensory cortex (SI), cerebellum, thalamus, primary motor cortex, STG, visual cortex, IFG, premotor cortex, supplementary motor area (SMA), basal ganglia, medial temporal gyrus, and ACC (Beissner 2011). Moreover, Sun et al. suggested that extensive deactivation mediated by acupuncture on fMRI was a nonspecific pernicious consequence of global normalization (Sun et al. 2012a). Accordingly, the hypothesis of Hui et al. that acupuncture produces deactivation of the limbic-paralimbic-neocortical network has been largely rejected.


Fig. 1.6
Commonly reported areas of activation in fMRI studies of acupuncture (Reprint with permission from Beissner 2011)
Regional changes in brain activity due to acupuncture have been explored in areas such as the cerebellum, periaqueductal gray (PAG) , and default mode network (DMN) . One acupuncture fMRI study compared acupoint, sham acupoint, and tactile stimulation conditions and found that acupuncture manipulation at PC6 specifically activated the cerebellum in the declive, nodulus, uvula of vermis, quadrangular lobule, cerebellar tonsil, and superior semilunar lobule of the cerebellum (Yoo et al. 2004). These alterations might be related to the clinical utility of PC6 for cerebellar vestibular modulation.
Both animal and human studies have demonstrated that the PAG is rich in opioid receptors and mediates analgesic effects via the descending inhibitory pathway of pain processing (Behbehani 1995; Linnman et al. 2012). A previous report by Napadow et al. used brainstem-focused cardiac-gated fMRI to show that longer duration (> 30 min) of electrostimulation at ST36 specifically modulated activity in the substantia nigra, nucleus raphe magnus, locus coeruleus, nucleus cuneiformis, and PAG (Napadow et al. 2009b). PAG activity was also shown to be modulated by needling at LI4 compared to needling at a sham acupoint (Liu et al. 2004). Further, the functional connectivity of the PAG was investigated after electrostimulation at LI4 and a sham acupoint, and it was found that LI4 stimulation specifically increased connectivity between the PAG, left posterior cingulate cortex (PCC), and precuneus and decreased connectivity between the PAG and right anterior insula (Zyloney et al. 2010). Taken together, these studies suggest that acupuncture may modulate brainstem nuclei including the PAG as part of its therapeutic effect, particularly with respect to pain perception.
DMN refers to the brain regions of deactivation during goal-directed tasks but activation during no task by using resting-state functional MRI (Andrews-Hanna et al. 2014). It has been suggested that the DMN instantiates processes that support emotional processing, self-referential mental activity, and the recollection of prior experiences (Raichle 2015). The DMN is also regarded as one of the most important networks for the pain connectome and is thought to play a significant role in persistent pain (Kucyi and Davis 2015). Researchers have attempted to demonstrate the effects of acupuncture on the DMN using resting fMRI data and heart rate variability (HRV) taken before and after true and sham acupuncture. In one study (Dhond et al. 2008), needling at PC6 produced increased DMN connectivity in areas including the ACC, PAG, amygdala, hippocampus, and middle temporal gyrus (MTG). Furthermore, the increased connectivity between DMN and hippocampus was associated with acupuncture-induced increases in parasympathetic tone and decreased sympathetic tone. Another study found that the DMN was subject to different patterns of modulation in response to stimulation at different acupoints or sham acupoints (Liu et al. 2009). Specifically, EA stimulation interrupted connectivity between the PCC and ACC and produced a negative interaction between the orbital prefrontal cortex (OFC) and left MTG. The ability of acupuncture to regulate connectivity within the DMN and enhance connectivity between the DMN and pain inhibitory, memory, and affective brain regional networks might contribute to acupuncture analgesia and other potential therapeutic effects.
The relationship between the autonomic nervous system (ANS) and brain activity during acupuncture was explored using cardiac-gated fMRI (Napadow et al. 2005a). The authors calculated the low frequency-to-high frequency (LF/HF) ratio from simultaneous HRV and used this index to express the activity of the ANS. EA at ST36 caused alterations in the LF/HF ratio that were significantly correlated with BOLD signal activities in the hypothalamus, dorsal raphe nucleus, PAG, and rostroventral medulla. Accordingly, acupuncture has demonstrated the ability to modulate activity in the ANS, and this feature may represent another component of its therapeutic effect.
1.3.3 Comparative Studies of Brain Responses to Acupoint Stimulation on fMRI
According to TCM, each acupoint has a particular location and function. An important question arises from this idea: what are the differences between different acupoints, and can these differences be visualized using fMRI? Acupoints in same meridian may have similar functions, whereas acupoints belonging to the same anatomical segment or existing adjacently have similar locations. Accordingly, new research has evaluated whether these acupoints also have shared neurological substrates. Whereas previous sections of this chapter described acupoint specificity, this next section will focus on studies differentiating between the effects of different acupoints.
Acupoints in the same meridian. The meridian is a concept of TCM that is defined as a pathway of qi and blood circulation. Accordingly, acupoints on the same meridian typically have similar functions. The acupoint pairs LR3/LR6 and ST36/ST43 were chosen to investigate brain responses to acupoints on the same meridian (Li et al. 2008). Stimulation at LR3 and LR6 both belonging to the liver meridian collectively activated the SI, superior parietal lobe (SPL), and cerebellum. Acupuncture at LR3 exclusively activated the medial frontal gyrus, middle frontal gyrus (MFG), MTG, ACC, lentiform nucleus, thalamus, and insula. In contrast, acupuncture at LR6 solely activated the ipsilateral superior frontal gyrus (SFG), middle occipital gyrus (MOG), and lingual gyrus. When stimulating at ST36 or ST43 which pertains to the stomach meridian, the SI, MFG, and cerebellum were the commonly activated regions. The SPL, MOG, and occipital pole were also activated by acupuncture at ST36. The active areas including in the SII, IFG, and thalamus were evoked only by acupuncture at ST43. However, this study lacked a statistical comparison between acupoint fMRI results and accordingly may not have been accurately reported. Another study reported partial overlap between brain activity changes in response to stimulation at PC6 and PC7, although PC6 produced a greater extent of cortical activation than did PC7 (Bai et al. 2010); only the posterior insula exhibited differential deactivation between the two acupoint stimulation conditions. Taken together, it can be concluded that while some overlap has been reported in brain responses to the stimulation of different acupoints in the same meridian, there exists no clear evidence to support the hypothesis that meridian acupoints exclusively produce the same brain responses.
Acupoints in the same nerve segment or anatomical location. Skin and tissue within a nerve segment share the same ascending sensory pathways. HT7 and SI6 are two acupoints located in the same nerve segment that were stimulated to investigate whether acupoints with similar locations elicit similar specific brain responses (Zhong et al. 2010). Acupuncture at HT7 increased BOLD signal in the right postcentral gyrus and left IFG, whereas acupuncture at SI6 increased the signal in the left IPL and right IFG. Another study investigated the specific functional brain networks modulated by another pair of acupoints located in the same segment, GB40 and KI3 (Chen et al. 2012). Post-acupuncture resting-state functional brain network maps were constructed after needling at each acupoint. When comparing resting-state networks before and after KI3 stimulation, increased connectivities between the posttemporal cortex and dorsolateral PFC as well as the posttemporal cortex and ventromedial PFC were identified. These connectivities were related to cognitive functions, consistent with the known function of KI3. In contrast, increased connectivity between the anterior insula and temporal cortex emerged following acupuncture at GB40 relative to the resting state. The abovementioned studies demonstrate that stimulation at different acupoints in the same anatomical segment does not necessarily evoke similar brain responses; rather, specific responses appear to be related to the mechanism of the known therapeutic effects for each acupoint.
Two adjacent acupoints, LR3 and ST44 , and a nearby non-acupoint were selected to investigate relative specificity among different acupoints. Healthy volunteers were recruited and received acupuncture at one of the three points during image scanning. The results revealed that areas commonly activated by LR3 or ST44 stimulation were the contralateral SI and ipsilateral cerebellum. LR3-specific activation sites included the contralateral MOG, ipsilateral medial PFC, SPL, MTG, rostral ACC, lentiform nucleus, insula, and contralateral thalamus. In contrast, ST44-specific activation sites included the ipsilateral SII, contralateral MFG, IFG, lingual gyrus, lentiform nucleus, and bilateral PCC (Fig. 1.7) (Liu et al. 2013). Consistent with the abovementioned studies, Liu et al. demonstrated that stimulation at adjacent acupoints elicited distinct patterns of cerebral activation potentially related to the functional effects of each specific acupoint.


Fig. 1.7
Activation in response to LR3 and ST44 stimulation with respect to stimulation of a nearby non-acupoint (Reprint from Liu et al. 2013). ACC anterior cingulate cortex, IFG inferior frontal gyrus, MFG middle frontal gyrus, MOG middle occipital gyrus, SII secondary somatosensory area, SPL superior parietal lobe
Specificities of acupoints in different meridians. According to TCM theory, it has also been proposed that some acupoints in different meridians might have similar functions. To this end, a few studies have evaluated the ability of acupoints with similar therapeutic effects to produce similar alterations in BOLD signal on fMRI (Fang et al. 2006; Zhong et al. 2010; Claunch et al. 2012). The specificity and commonality of brain responses to acupuncture at LI4, ST36, and LV3, which are acupoints used to treat pain disorders, were evaluated using fMRI (Claunch et al. 2012). Common activated areas included the SII, dorsolateral and dorsomedial PFC, anterior insula, and thalamus. However, differences were observed in the spatial distribution and degree of deactivation of the medial PFC, medial parietal cortex, and medial temporal lobe, suggesting that the three acupoints produced brain responses some relative specificity. Further supporting this point, while LI4 stimulation primarily elicited responses in the pregenual cingulate and hippocampus, ST36 elicited responses in the subgenual cingulate, and LV3 produced changes in the posterior hippocampus and posterior cingulate. Accordingly, these acupoints may modulate the same intrinsic global networks by different mechanisms to produce the same therapeutic effect. The commonality and specificity of brain responses to acupoints in different meridians have been similarly reported for other acupoints (Fang et al. 2006).
In summary, although the results of acupuncture fMRI studies are heterogeneous, multiple studies have demonstrated the ability of acupuncture to modulate activity within specific brain regions, including the somatosensory cortices, limbic system, basal ganglia, brain stem, and cerebellum (Huang et al. 2012). Moreover, several studies support the ability of acupoints to produce brain responses in a manner potentially representing the functional specificity of their therapeutic effects (Bai et al. 2010; Chen et al. 2012; Claunch et al. 2012; Liu et al. 2013). This idea is supported by the fact that acupoints located in the same meridian or in the same anatomical segment can produce different brain responses with relative specificity.
1.4 fMRI Studies of Acupuncture Sensation
Acupuncture sensation or deqi refers to the experience of soreness, numbness, fullness, heaviness, and so forth elicited by needle insertion at a certain depth at a given acupoint. At the same time, the operator may feel heaviness or tension around the needle (Hui et al. 2007; Kong et al. 2007a). Notably, deqi is distinguishable from sharp pain that can sometimes emerge during acupuncture needling, which is regarded as non-deqi and harmful (Hui et al. 2011). Deqi is considered to be an indispensable component of acupuncture treatment, and this idea is supported by several clinical studies (Berman et al. 2004; Witt et al. 2005; Xiong et al. 2012). An understanding of deqi is therefore essential for elucidating the mechanisms and neural substrates of acupuncture.
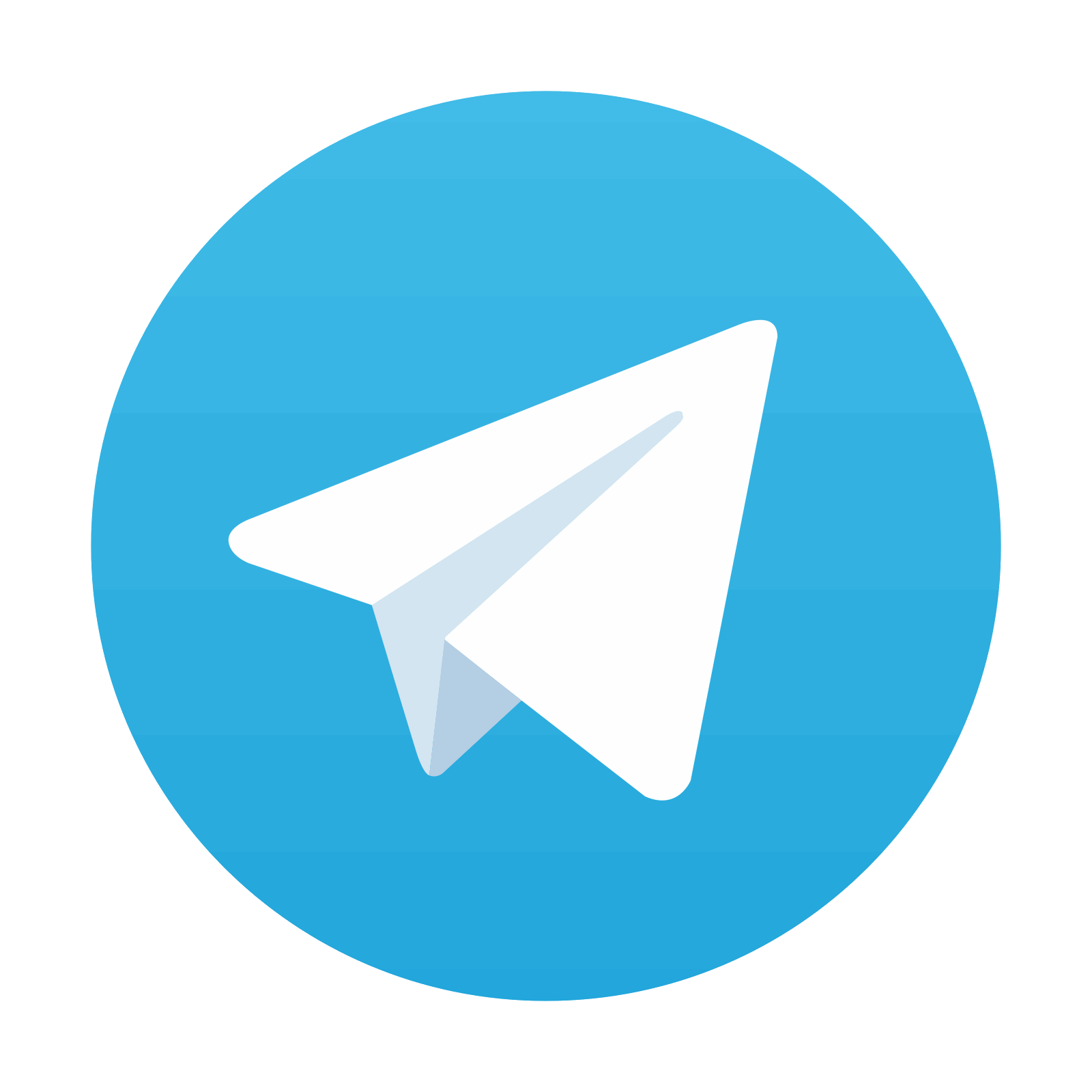
Stay updated, free articles. Join our Telegram channel

Full access? Get Clinical Tree
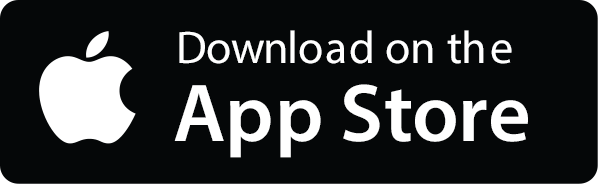
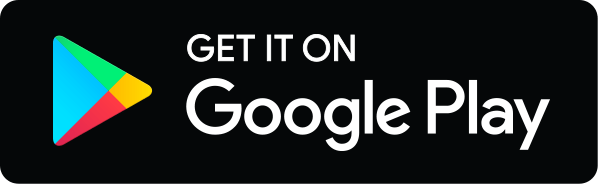