Embryology of the Craniovertebral Junction and Congenital Malformations in the Region
Dachling Pang
Dominic N.P. Thompson
The bony craniovertebral junction (CVJ) can be conceptually divided into two component parts with respect to the governance of intersegmental movements and functional space for the nervous system. The first is the central pivot made up of the dens and C2 vertebral body. The second are two ringed structures surrounding the central pivot, albeit eccentrically. They are the foramen magnum ring, comprising the basiocciput (clivus) and exocciput, including the occipital condyles: and the atlantal ring, with its anterior and posterior arches and lateral masses. These two superimposing rings transmit the lower brainstem and upper cervical spinal cord, while permitting limited rotatory and flexion-extension motions upon each other and around the dental pivot. Straddling these two rings and anchoring upon them are the stabilizing ligaments: the alar and apical dental ligaments at the upside of the pivot, the transverse atlantal ligament (TAL) across the main dental shaft, and the arching mantle of the tectorial membrane and cruciate ligament, strapping the clivus to the whole of the dens-axis assembly.
In keeping with the functional anatomy of the CVJ, the two main themes of clinical interests are instability and neural compression. Clinically significant developmental anomalies affecting, respectively, the “pivot” and “rings” also happen to follow this thematic division. Anomalies of the central pivot usually lead to instability, although basilar impression and a retroflexed dens can cause neural impingement. Anomalies of the surrounding rings result in deformity and crowding, but hypoplasia and aplasia of component parts can result in a weakened frame and loss of ligamentous anchorage. This chapter deals first with the normal development and genetic control of this region, followed by the embryogenesis, clinical relevance, and treatment of congenital malformations of the CVJ.
EMBRYOLOGY OF THE CRANIOVERTEBRAL JUNCTION
THE PRESOMITIC STAGE
At gastrulation, epiblastic cells from the embryonic plate caudal to the head process invaginate through the primitive streak to form mesoderm on each side of the neural plate, while cells from both sides of the dorsal lip of Hensen’s node migrate through the primitive pit to integrate into the midline notochord. The embryonic plate thus elongates by new additions to its rear (caudal) aspect (1). The anterior-posterior (rostrocaudal) polarity of the embryo is determined very early during gastrulation. The prechordal mesoderm rostral to the otic vesicle (and notochord) forms most of the bones and muscles of the head and face without ever developing somites. Caudal to this tissue is the somitic region that extends along the body axis down to the tip of the tail (Fig. 28.1). The anterior somitic region from the otic vesicle to the blastopore (future anus) corresponds to the future body axis from the occiput to the anus. Here, the epiblastic cells condense to form the parachordal mesoderm on each side of the notochord following systematic ingression movements through the primitive streak. This initially homogenous column of cells, also called presomitic mesoderm (PSM) or segmental plate, subsequently segregates into segmental clusters called somites, which will eventually give rise to the smooth muscle of the dermis, the axial musculature, the vertebral column, and support structures of the peripheral nervous system. After blastopore closure and complete regression of the primitive streak, the gastrulating region for the tail is restricted to a small region called the tail bud, located at the caudal tip of the primitive streak. The tail bud functions as a blastema of undifferentiated cells (2,3). The presomitic mesoderm and later somites of the caudal and tail regions are therefore not formed by epiblastic ingression but by progenitor condensation in situ.
PRIMARY SEGMENTATION: SOMITOGENESIS
Prior to the appearance of somites, the PSM remains a loose mesenchyme without specific cellular polarity or stratification pattern alongside the lengthening notochord and neural plate (4). During somitogenesis, the loose mesenchymal cells of the PSM undergo transformation into tightly apposed epithelial cells with definite polarity and orientation. A newly formed somite is a compact epithelial sphere (“somitomere”) composed of a single layer of radially arranged cells with apices pointing towards a central lumen (somitocoele), which contains a few mesenchymal cells (5) (Fig. 28.2). The epithelial cells have polarized Golgi zones, basally aligned nuclei, and actin molecules near the luminal border, the entire sphere being enveloped by a collagen-containing basal lamina (6). This epithelial conversion of the PSM is aided by an increase in cell-cell adhesion mediated by a sharp but transient rise in the levels of the calcium-dependent adhesion molecule N-cadherin, of fibronectin, and possibly cytotactin (7).
Somitogenesis begins soon after internalization of the prochordal (head) mesoderm and continues through subsequent production of the body axis. The first somite forms immediately caudal to the otic vesicle (8), followed by sequential transformation such that a new pair of somites is regularly added in a rostrocaudal direction until a fixed species-specific number of somites is reached. Thus, depending on the stage of gastrulation, the growing column of body mesoderm consists of a rostral section of mature somites already undergoing differentiation into sclerotomes and dermomyotomes, a middle section of new predifferentiated epithelial somites, and a caudal section of presomitic mesoderm just rostral to the remaining primitive streak, the whole enterprise necessarily evolving in parallel with the neurulating neural plates (Fig. 28.3).
![]() Figure 28.2. Somite with radially arranged and polarized epithelial cells surrounding a central cavity, the somitocoele (SC) and enveloped by a basal lamina (BL). |
Current thinking regarding the mechanism of metameric transformation of the PSM follows what is called the “Clock and Wavefront” model, first proposed by Cooke and Zeeman in 1976. In this model, PSM cells oscillate between a permissive and a nonpermissive state for somite formation. These oscillations are phase-linked and controlled cell-autonomously by a “segmentation clock.” Somitic formation is triggered when cells of the rostral PSM, while in the permissive phase of the clock, are hit by a wavefront of maturation (or determination) that slowly moves caudally along the embryonic axis. Thus, the clock generates a temporal periodicity that is translated spatially into the periodic boundaries of the somites (4).
At the molecular level, the phasic oscillation of the segmentation clock is reflected by rhythmic expressions of a class of genes called cycling genes (9). These genes include the c-hairy1 family in fish, chick, frog, and mouse, which encode transcription factors such as the split (HES) family and the glycosyltransferase Lunatic Fringe (10,11), all tightly involved in the notch signaling pathway and its ligands, suggesting that periodic notch activation plays a critical role in the oscillator. Also, these genes oscillate largely in synchrony in the PSM, suggesting they are downstream of a common cycling activator.
In chick and mouse embryos, the maturation or determination wave is generated by the expression of genes, which include the fibroblast growth factor gene fgf8 (12). It is known that the caudal domain of the PSM is very high in the factor FGF8, which seems to actively maintain the mesenchymal identity of caudal PSM cells. The FGF8 gradient decreases toward the rostral axis, so that at the rostral domain of the PSM where somitogenesis is taking place, FGF8 level is very low (Fig. 28.3). Also, overexpression of fgf8 in this region results in inhibition of somitogenesis. Thus, the activation of epithelization of PSM cells is negatively regulated by FGF8, whose absence allows the PSM cells to become competent to respond to the clock signal and initiate somitic boundary formation (13). The limit between the two domains of high (in the caudal PSM) and low (in the rostral PSM) fgf8 expression therefore marks the determination wavefront of somitogenesis (4).
Due to the constant caudal elongation of the body axis during gastrulation and the addition of new PSM cells with strong fgf8 expression to the rear, the FGF8 gradient is continuously displaced caudally. Accordingly, the determination wavefront also moves slowly in a caudal direction along the body axis. This ensures that the old and new metameric boundaries, at both ends of a new somite, are separated by a distance corresponding to the caudal displacement of the determination wavefront during one period of oscillation of the segmentation clock (9). The speed of new somite production is thus linked to the clock period, which is species specific: 30 minutes for zebra fish, 90 minutes for chick, and 120 minutes for mouse (14) (Fig. 28.3).
DIFFERENTIATION OF THE SOMITIC MESENCHYME
Within hours after its formation, each somite starts differentiating along its dorsoventral axis. Cells from its ventromedial part lose their epithelial arrangement and migrate toward the notochord to form, with the luminal cells, the mesenchymal sclerotomes (5,15). Together with the notochord, the sclerotomes provide exclusive material for the vertebral column. Cells from the dorsolateral part of the somite retain their epithelial arrangement to produce the dermomyotomes (Fig. 28.4A and B). The dermomyotomes later subdivide into the dorsal dermatome immediately beneath the ectoderm to give rise to the dermis and its smooth muscles, while the disaggregated cells between the dermatome and the sclerotomes remain closely packed as the myotome, forming ultimately the axial skeletal muscles (1,15,16) (Fig. 28.4 C and D).
While specification of the anterior-posterior pattern of the somite appears to be determined very early (17, 18 and 19), the dorsoventral values are not intrinsic to the somites. Thus, if the somite were to be surgically rotated dorsoventrally by 180°, sclerotomes still develop in the ventromedial position while the dermomyotomes remain dorsolateral. Moreover, a dorsally implanted notochord represses dermatomal but encourages sclerotomal formation, while notochord ablation completely prevents sclerotomal appearance (1). These findings suggest that the dorsoventral differentiations of the somite depend on appositional instructions from the notochord, very possibly through sonic hedgehog (Shh) expression (20).
“RESEGMENTATION” OF THE SCLEROTOME
The term “resegmentation” was originated by Remak in 1855 (“Neugliederung”) (21) and remains still a subject of controversies (1,22). It refers to the fact that the early metameric boundaries between the somites are once again changed and “reshuffled” during the development of the sclerotome, so that the later boundaries between the vertebral bodies do not match up with the original intersomitic clefts (15).
During somitic differentiation, the ventromedial cells destined to become sclerotome subdivide into paired lateral clusters flanking the ventral aspects of the neural tube and an unpaired median (axial) cluster surrounding the midline notochord (Fig. 28.5). The formation of the vertebral column takes place first in the lateral sclerotome, where a conspicuous subdivision into a densely packed caudal half and a more loosely cellular cranial half soon appear, separated by a fissure (of von Ebner (23)). The loose cranial half attracts, promotes, and supports the growth and expansion of peripheral nervous tissue from the neural tube and neural crests but itself never chondrifies into vertebral parts (Fig. 28.6, middle). In contrast, the caudal densely packed mesenchyme of the lateral scleroderma soon takes on a triangular shape. The side of the triangle facing the axial perichordal sclerotome, which will become the future vertebral body, gives rise to the pedicle. The side facing dorsolaterally away from the axial sclerotome forms rudiments of the neural arch, and the side of the triangle facing ventrolaterally becomes the costal process (Fig. 28.4D).
Shortly after the specifications of the lateral sclerotome into its arcual, costal, and pedicular components, the initially loosely meshed mesenchyme within the perichordal axial sclerotome also begins to show compartmentalization. A cell-dense zone develops at the same level as the dense caudal half of each lateral sclerotome, partly due to medial expansion of the lateral band of condensed tissues from the lateral sclerotome. The axial sclerotome in between these median dense zones remains loosely cellular. Later, the cranial-most layer of the axial dense zone, in line with von Ebner’s fissure in the lateral sclerotome, becomes even more tightly packed and forms the intervertebral boundary zone (IBZ) (Fig. 28.6, middle). This intervertebral boundary mesenchyme (IBM) ultimately forms the ring-like annulus fibrosus of the intervertebral disk, permanently enclosing a looser central core of nucleus pulposus made partly of notochordal remnants. The vertebral body itself is mainly made from chondrogenesis in the loose-cell zone of the perichordal sclerotome, now called the prevertebra (1,15,24,25), although contributions from the condensed tissue adjacent to the IBZ have been observed (22,26). As a final step, the pedicular anlagen from the lateral sclerotomes fuse with the chondrifying prevertebra of the axial sclerotome, while the neural arches surround the neural tube to complete the vertebral ring (Fig. 28.6, right). The costal component becomes the future transverse process but only in the thoracic region is it predestined to form ribs.
Thus, the neural arch of the vertebra is derived from the caudal-lateral part of a single somite (22). However, labeling and transplantation experiments of half somites have repeatedly demonstrated that each vertebral body is made up of cells from the axial zones of two adjacent somites (27,28). Although the exact boundary of individual somite participation is not known (15), the juxta-positioning of axial and lateral sclerotomal components suggests that each vertebra probably comes from the caudal half of one somite and the rostral half of the somite below (25). This will explain the slightly off-step registration between the levels of the somite and the resegmented sclerotome (15,25,29) on the embryonic axis such that the middle of the resegmented sclerotome lines up with the original intersomitic cleft (Fig. 28.6, left and middle). Also, since the dense portion of the lateral sclerotome is in line with the dense zone of the axial sclerotome adjacent to the IBZ, it makes perfect sense that the mature pedicle is joined to the cranial and not the caudal half of the vertebral body (15). It also follows that the spinal nerve, ganglion, and blood vessel from the corresponding somitic segment, being associated with the loose cranial half of the lateral sclerotome, must cross above its own neural arch, and that the corresponding segment of the spinal cord is always slightly more rostral to its companion vertebral body (Fig. 28.6, right). Given there are eight cervical somites but only seven resegmented axial sclerotomes and consequently seven cervical vertebrae, the C1 nerve root emerges above the C1 neural arch and the C8 nerve root comes through below the C7 neural arch and above the T1 neural arch, which in fact is derived from the C8 somite. Finally, resegmentation of the sclerotome explains why the original “pre-resegmented” intersomitic vessel ultimately enters the midpoint of the vertebral body as the segmental nutrient artery.
SPECIAL DEVELOPMENTAL FEATURES OF THE CVJ
The CVJ is the product of the occipital somites and the first three cervical somites. There is controversy regarding the proper number of occipital somites in vertebrates. Wilting et al. and others (30, 31 and 32) thought there were five occipital somites in chick and mouse but conceded that the first somite either disappeared early or was an insignificant clan of cells that lacked sclerotomal lineage. Müller and O’Rahilly (25) studied staged human embryos and concluded that, in humans, there are only four occipital somites that participate in formation of the skull base. The transitional zone between the skull and the cervical spine is thus taken to be between the 4th and 5th somites. During the 4th week of gestation, there are consequently 4 occipital, 8 cervical, 12 thoracic, 5 lumbar, 5 sacral, and 8 to 10 coccygeal somites, 42 pairs all told.
OCCIPITAL SOMITES (SOMITES ONE TO FOUR) AND THE PROATLAS
Following the general schema, the first three occipital somites give rise to an axial perichordal sclerotome and a lateral sclerotome, but no resegmentation takes place here. The axial sclerotomes never subdivide into dense and loose zones, and therefore no IBM exists. They all eventually fuse into a unit, which later chondrifies to become the rostral basioccipital (25). The first three lateral occipital sclerotomes, like the vertebral sclerotomes, form dense and loose zones, and the loose zones of the second and third lateral occipital sclerotomes foster expansion of the upper and lower hypoglossal nerve roots and artery, while the corresponding dense zones form the bony hypoglossal canal.
Unlike the first three occipital somites, the fourth occipital (O4) somite does show resegmentation. Its caudal dense zone combines with the cranial loose half of the first cervical somite to produce the transitional sclerotome called the proatlas (25,29,33, 34, 35 and 36) (Fig. 28.7, left and middle). The cranial region of the axial sclerotome of the proatlas soon fuses with the other three axial occipital sclerotomes to become the basion of the basioccipital (36), but its most caudal portion, probably derived from the first cervical somite (somite five), forms the anlage for the apical segment of the dens. Late in resegmentation, a boundary zone appears between this apical dental centrum and the loosely cellular prevertebra of the basioccipital, and the former soon detaches from the basioccipital and eventually becomes joined to the basal segment of the dens to complete the dental pivot (see below) (25,32) (Fig. 28.7). Herein lies the most unique feature of the transitional zone of the CVJ between somites four and five: Unlike other IBZs that form intervertebral disks, downstream activity of the proatlas’ IBZ includes a physical severance of cells from the immediately adjacent loose perichordal zone of the basioccipital. This action, no doubt mediated by special cleavage genes, allows not only the skull to become independent from the vertebral column but also the final installation of the dens-axis assembly.
The lateral dense region of the proatlas becomes the two exoccipitals, which later form the two occipital condyles and the remainder of the anterolateral rim of the foramen magnum (Fig. 28.7). The lateral loose region promotes emergence of the C1 nerve root. In humans, an additional arcuate cluster of dense proatlas cells ventral to the notochord, aptly called the hypochordal bow, gives rise to the bony anterior clival tubercle on the ventral surface of the basioccipital (25,36) (Fig. 28.7).
FIRST THREE CERVICAL SOMITES (SOMITES FIVE TO SEVEN)
Axial Sclerotomes
During resegmentation, the caudal half of somite five and the cranial half of somite six combine to produce the first cervical sclerotome; likewise the second cervical sclerotome is made up of corresponding parts of somites six and seven. In the axial region of these sclerotomes destined to form vertebral centra, dense and loose zones appear in regular succession as in the lower cervical sclerotomes. The loose prevertebral zone of the first cervical sclerotome gives rise to the basal segment of the dens, and that of the second cervical sclerotome becomes the body of the axis (Fig. 28.7). Unlike in the more caudal sclerotomes, however, where the dense IBZ ultimately becomes the annulus and nucleus pulposus of an intervertebral disk, the dense zones in the first two cervical sclerotomes do not form true intervertebral disks and soon disappear (25). Their IBM gradually turns into the upper and lower dental synchondroses that ultimately cement the apical to the basal dens and the basal dens to the body of C2, respectively (Fig. 28.7).
Thus, after resegmentation, the human membranous axis consists of three median constituents that have been designated the apical dental segment from the caudal proatlas, the basal dental segment from the first cervical sclerotome, and the body of the axis from the second cervical sclerotome (31,37, 38, 39 and 40). These three constituents chondrify simultaneously around 6 weeks of gestation but remain segregated by the more cellular upper and lower dental synchondroses. Ossification of the cartilaginous axis occurs in three chronologic waves (Fig. 28.8). The first wave appears as a single ossification center within the axial body around 4 months of gestation. The second wave begins at 6 months of gestation as two separate ossification centers on each side of the basal dental segment (41, 42, 43 and 44). At birth, these two centers integrate and fuse, and the main component of the dens should at least have begun to show bony fusion with the axis body, even though a clear rarefaction may still be discernible at the lower synchondrosis till the fifth or sixth postnatal year (Figs. 28.8 and 28.9). Occasionally, the basal dens remains bifid (dens bicornis) when the third wave of ossification arrives within the apical dens around 3 to 5 years of age (37,42,45) (Figs. 28.8, 28.9 and 28.10). Ossification of the dental tip and bony fusion of the upper synchondrosis are not completed until adolescence (46, 47, 48 and 49).
Lastly, the apical ligament is almost certainly derived from the axial proatlas, and the alar and TALs are from the axial component of the first cervical sclerotome in association with the basal dental segments (25).
Lateral Sclerotomes
The lateral dense zone of the first cervical sclerotome develops into the posterior arch of the atlas, while the lateral dense zone of the second cervical sclerotome forms the arch of the axis. Their respective loose zones promote outgrowths of the second and third cervical nerves and segmental arteries. The hypochordal bow of the first cervical sclerotome ventral to the notochord subsequently forms the anterior arch of the atlas (Fig. 28.7, middle and right) (25,36,40,50). No definite hypochordal bows are seen caudal to this level, and equivalent cells in the lower segments appear to play no role in the formation of the vertebral column.
GENETIC CONTROL OF CVJ DEVELOPMENT
HOX GENES: THE CONTROL OF ROSTROCAUDAL SPECIFICATION
Following primary segmentation, the determination of the positional identity of the prevertebral segments along the embryonic axis, which in turn ordains the regional developmental specifications of the vertebral phenotypes, is controlled by Hox genes. The mammalian Hox genes encode transcription factors used in regulating the establishment of the body plan. They contain the phylogenetically highly conserved homeobox domain (1,51,52). In mouse and humans, there are 39 Hox genes distributed in four linkage clusters, Hox A, B, C, and D, on four different chromosomes (chromosomes 6, 11, 15, and 2). The members of each cluster, designated by Arabic numerals, are also grouped vertically along the clusters because analogous members of each group are linked by common origin from a single ancestral gene, so that Hox a4, b4, c4, and d4 are connected to the same phylogenetic origin and are called paralogues. The lower numbered paralogues are located on the anterior 3’ axis of the chromosome and the higher numbered ones are on its posterior 5’ locations (Fig. 28.11).
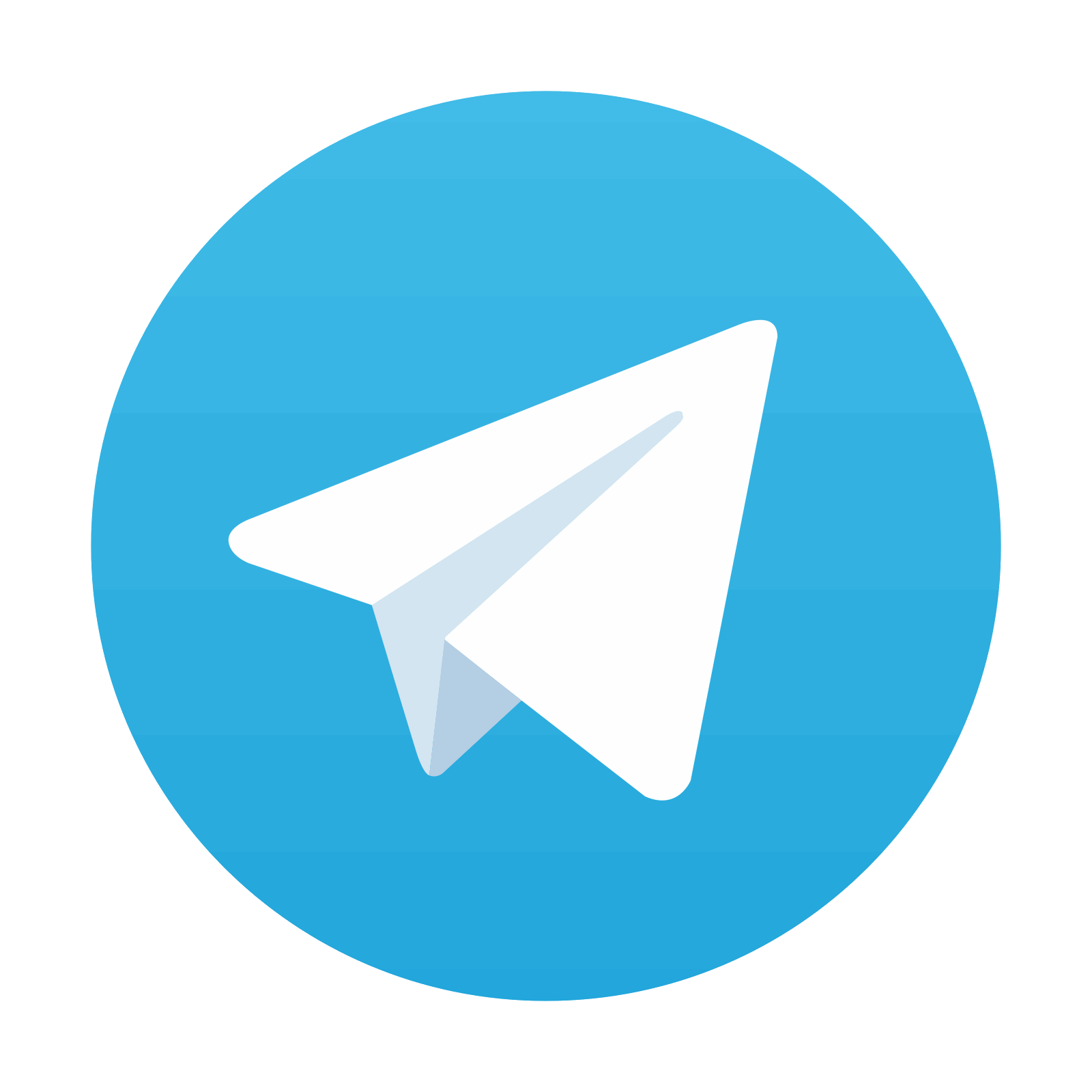
Stay updated, free articles. Join our Telegram channel

Full access? Get Clinical Tree
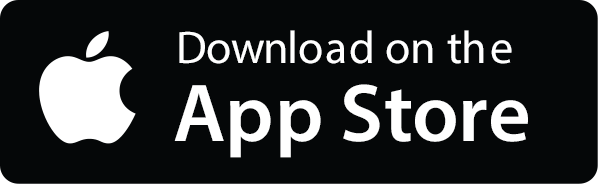
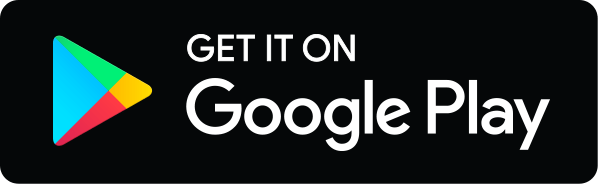