1 Embryology of the Spine
KEY POINTS
Gastrulation
Gastrulation begins in the third week of gestation and gives rise to three distinct germ layers, the ectoderm, the mesoderm, and the endoderm. The initial phase of gastrulation begins with formation of the primitive streak, which is sometimes named the primitive groove (Figure 1-1). This midline thickening of the germinal disc terminates in the primitive node. Under control of embryonic growth factors, cells of the epiblast layer migrate inward to form the mesoderm and the endoderm through the process of invagination. Cells migrating farthest from the epiderm and closest to the yolk sac become the endoderm. The remaining epiblast cells will eventually differentiate into the ectoderm (Figure 1-2). The migrating cells that are sandwiched between the endoderm and ectoderm layers will become the mesoderm. Control of these migrations is maintained through various cell-signaling pathways that also contribute to establishment of the body axes in all planes. The signaling pathways, or organizer genes, are secreted by the primitive streak and mesoderm. The cranial direction of the embryonic disc is established by a specialized area of cells, referred to as the anterior visceral endoderm, that expresses genes required for formation of the head and cerebrum. The dorsal-ventral axis is regulated by growth factors in the TGF-β family including bone morphogenic protein-4, fibroblast growth factor, and the sonic hedgehog gene. Control of sidedness is regulated by fibroblast growth factor-8, Nodal, and Lefty-2, all of which are secreted on the left side of the germinal disc. An additional protein, Lefty-1, is secreted to prevent migration of the left sided growth factors across the midline.1
Somite Period
The presence of the notochord induces proliferation of the mesoderm. At approximately 17 days of gestation the mesoderm thickens into two masses, each located directly adjacent to the notochord. This initial layer, termed the paraxial mesoderm, continues to spread laterally to eventually differentiate into three distinct areas, paraxial mesoderm, intermediate mesoderm, and lateral mesoderm. During the somite period, lasting from approximately 19 to 30 days post fertilization, the paraxial mesoderm will develop into segmental bulbs of tissue on either side of the notochord (Figure 1-3). The first pair of somites will appear adjacent to the notochord, and they will continue to develop in a cranial to caudal direction until a total of 42 to 44 pairs of somites appear by the end of the fifth week of gestation. The first 24 somite segments are responsible for the cervical, thoracic, and lumbar spine. Somites 25 through 29 contribute to formation of the sacrum, while pairs 30 through 35 are responsible for coccyx formation. The rest of the 42 to 44 somite pairs disappear through a process of regression, which occurs at approximately 6 weeks of gestation.
The somites continue to differentiate into two distinct tissues. Ventromedial cells develop into the sclerotome, while dorsolateral cells develop into the dermatomyotome. The latter cells will eventually give rise to the integument system and dorsal musculature of the body, while the sclerotome will migrate to surround the notochord and give rise to the vertebral column. Regulation of sclerotome formation is controlled by proteins coded by the sonic hedgehog gene, which is expressed by cells of the notochord. This process of sclerotome migration will begin by the fourth week of gestation. Each sclerotome will be divided by an intersegmental vessel and a loose area of intersegmental mesenchyme. In addition, a pair of myotomes and accompanying segmental nerves will be associated with each sclerotome.
As the process of differentiation continues, each sclerotome will divide into a cranial region of relatively loosely packed cells and a caudal portion of rapidly proliferating and densely packed cells. At this point in spinal development, classic embryology texts describe a phenomenon by which the pace of proliferation is so great that the caudal part of the one sclerotome begins to overgrow into the cranial portion of the adjacent sclerotome and thereby fusing to create a single mass of tissue destined to become the precartilaginous vertebral body. Parke (The Spine, 1999) suggests that this theory of “resegmentation” may not be accurate, and provides compelling evidence toward an alternate route of vertebral body formation. In his summary of the recent evidence, Parke outlines a pathway of spinal development which begins with a uniform layer of axial mesenchyme surrounding the notochord. The sclerotomal organization is still maintained with an intersegmental vessel, a nerve, and a peripheral layer of dermatomyotome associated with each segment. However, the uniform mesenchyme undergoes a period of differentiation by which densities develop within the loose tissues. These dense regions will develop into the intervertebral discs and eventually pinch off the notochord which will be trapped within the dense tissue to become the nucleus pulposus.2 The loose tissues between the discs form the cartilaginous centrum, which is the precursor of the vertebral bodies. The caudal portion of the centrum undergoes rapid proliferation, and cells migrate peripherally to surround the neural tube, forming the membranous neural arches which will serve to protect the neural elements. In total, each bony vertebral segment will consist of five ossification centers, one centrum, two neural arches, and two costal elements.
Ossification of the vertebral bodies occurs around the ninth week of gestation and begins at the thoracolumbar junction. Ossification then proceeds in both cranial and caudal directions, with the caudal segments demonstrating a quicker rate of ossification compared with the cranial segments. Ossification of the posterior arches begins at approximately the same time but begins in the cervical vertebrae and proceeds in a caudal direction. As the two neural arch centers approach midline, they begin to fuse, forming the lamina and spinous process. Fusion of the neural arches first occurs in the lumbar segments during the first year of life and proceeds cranially. Fusion is not completed until ages of 5 to 8 years. The costal ossification centers have a variable role in vertebral body formation. In the cervical spine, these centers have a minimal contribution and may contribute to part of the foramen transversarium. In the thoracic spine, these ossification centers are the precursors of the ribs. In the lumbosacral spine, the costal ossification centers are responsible for formation of the transverse processes and the anterolateral portion of the sacrum.2
Upper Cervical Spine
A detailed anatomic study of cervical spine anatomy was presented by O’Rahilly and Meyer in a serial time reconstruction of human embryos ranging from 8 to approximately 16 weeks of gestation.3 It is generally believed that the most cranial 4 or 5 pairs of somites are responsible for the occipital-atlas complex. Development of this junction is regulated by growth factors derived from the notochord as it crosses into the cranium. The notochord travels through the middle or slightly anterior portion of each centrum, and up through the future dens at the level of the axis. It then makes an anterior directed turn to enter the skull just above the level of the dens. At this time, each centrum is divided by a thickening of the notochord that will develop into the nucleus pulposus. The true boundary between the spine and cranium is not fully understood, with some authors suggesting that the atlas is a standalone accessory cranial bone with the true head-neck boundary being between the C1 and C2 articulation.
O’Rahilly and Meyer describe the centrum of the axis as being composed of three axial columns which they termed X, Y, and Z. The first and most cranial column, X, will develop an articulation with the anterior tubercle of C1, forming the atlanto-dens joint space.4 By approximately 9 weeks of gestation, the X column, or future dens, is already bounded posteriorly by the transverse ligament and anchored into the occipital condyles by the alar ligamentous complex. Columns Y and Z are separated by the remnants of an intervertebral disc that may persist well into birth. Although it is generally accepted that column Z will form the centrum of the axis, the fate of column Y remains uncertain. Some believe that it is incorporated into the axis centrum, while other embryologists believe, based on reptilian studies, that it is incorporated into the centrum of the atlas. Calcifications in the three columns are readily visible by the time the embryo reached a length of 120 mm; however, fusion will not take place until 6 to 8 years of age. In some instances, the tip of the dens may calcify independently without fusion to the remaining axis; this is termed os odontoideum.
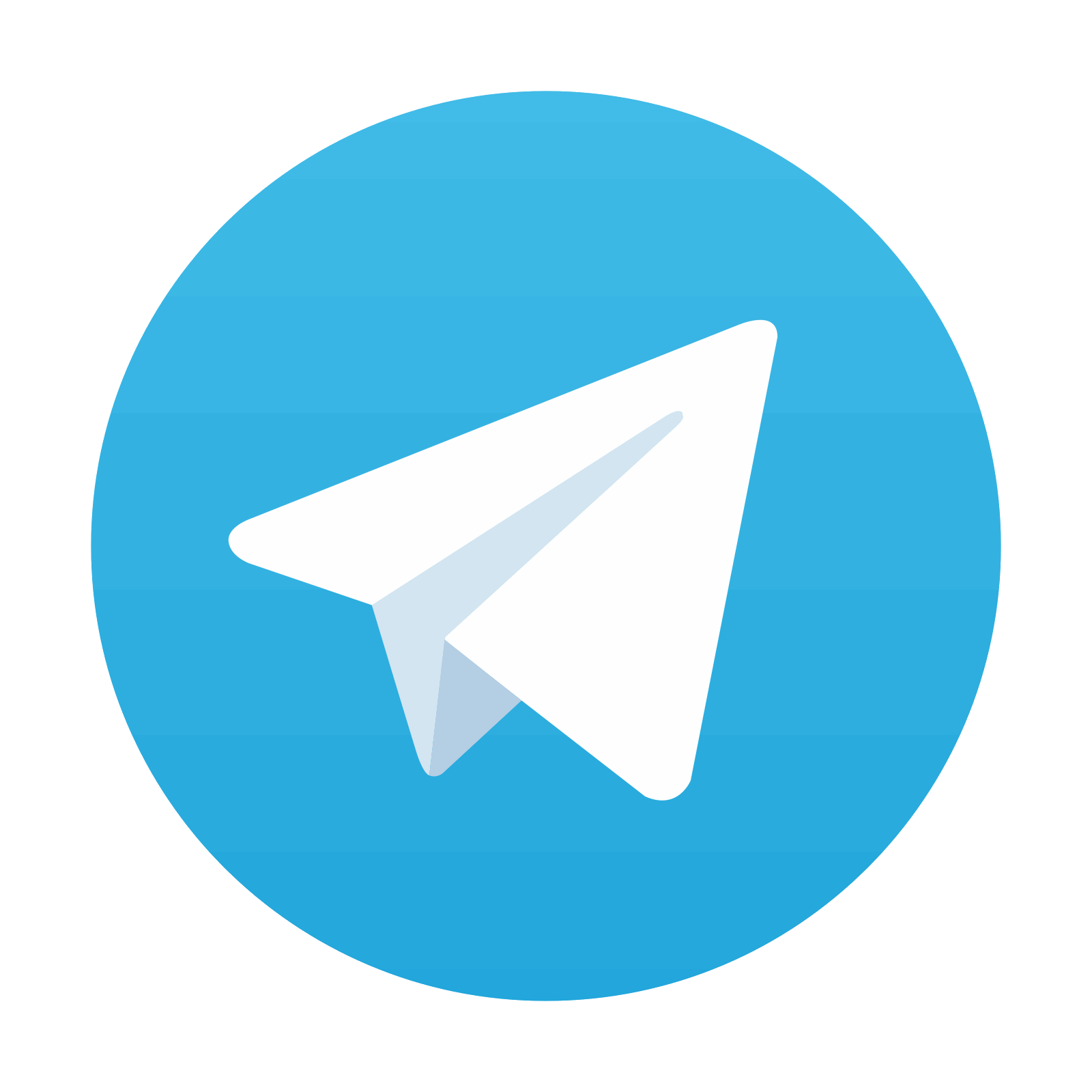
Stay updated, free articles. Join our Telegram channel

Full access? Get Clinical Tree
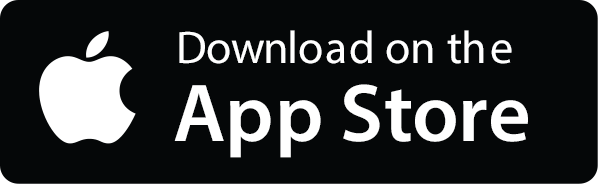
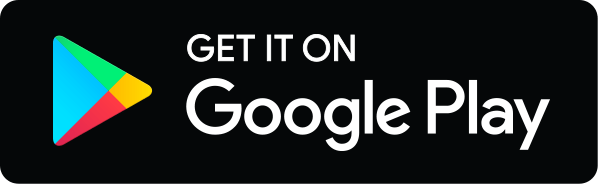