Fig. 21.1
Schematic representation of the unique environments within the gut wall. The innermost, toward the center of the gut tube, is the mucosa (Muc) with a thin muscle layer, the muscularis mucosa (MM) just below. Enteric ganglia are located within the submucosal (SMP) and myenteric (MP) plexuses which are separated by a thick layer of circular muscle (CM). Longitudinal muscle (LM) coats lie between the MP and the outer serosal (SER) surface of the gut
The cell bodies of enteric neurons lie within the submucosal and myenteric plexuses and primarily function to coordinate gut secretion/absorption and motor activity, respectively. The neurochemical coding and functions of enteric neurons are diverse and integration within the enteric nervous system by enteric sensory, inter- and motorneurons provides all the neurocricuitry necessary to carry out essential gut functions (Furness 2000) . Unlike motor circuits in the spinal cord, enteric motorneurons and secretomotorneurons are sandwiched between their effector end organs and provide local, moment-to-moment coordination of gut musculature, glands and blood flow.
Nerve fibers of enteric motorneurons course through the smooth muscle coats and diffusely innervate regions of smooth muscle. In the gut, the benefits of coordination outweigh the need for precise control of individual muscle fibers and neuromuscular junctions are traded in favor of mechanisms to promote coordination. Neurotransmitters released from enteric varicosities act upon a network of gap junction coupled interstitial cells of Cajal (ICCs) that lie between nerve fibers and smooth muscle cells (Sanders et al. 2012) . The ICCs thus distribute the neuron signal to a region of smooth muscle and function to coordinate gut activity.
We are now becoming increasingly aware that an additional cell type, called enteric glia , is ideally positioned to influence cellular interactions in each signaling compartments throughout the gut wall. Enteric glial cells intercalate between neurons within enteric ganglia and between neurons and cells within the mucosa and smooth muscle coats. Accumulating evidence suggests that enteric glia play essential roles in processes that control gut function. Importantly, alterations to glial functions have the potential to drive disease processes and gut pathology. Thus, we now recognize not only the need to incorporate enteric glial cells as additional players in physiological processes, but also that enteric glia are important drug targets to improve, or prevent gut pathophysiology.
21.3 Enteric Glia: The Astrocytes of the Gut
What exactly is an enteric gliocyte ? The answer to this question may not be as simple as we once thought because discrete differences between populations of glial cells in the gut wall are becoming increasingly apparent. Dogiel published the first description of glial cells associated with enteric neurons in 1899 but enteric glia were practically omitted from further study for the next 70 years or only briefly noted and incorrectly described as Schwann cells. Dogiel described what he saw as “nucleated stellate cells” associated with enteric neurons and assumed that these nucleated cells were some sort of connective tissue (Dogiel 1899) . Unfortunately, this conclusion was broadly accepted and enteric glia were not fully appreciated as a unique class of peripheral glia with distinct characteristics until the detailed anatomical analyses of Giorgio Gabella in the early 1970s (Gabella 1972) .
The classical definition of an enteric gliocyte refers to glial cells associated with enteric neurons and nerve fiber bundles within the enteric plexuses. These cells are easily identified by their stellate-shaped morphology with many highly irregularly branching processes (Gershon and Rothman 1991) , similar to astrocytes in the CNS (Fig. 21.2) As in the brain, glial cells embrace enteric neuron cell bodies within enteric ganglia and the dense synaptic neuropil within enteric ganglia and interganglionic nerve bundles is solely comprised of enteric neurons and glia. The majority of the glial cell body is filled by a 2–3 μm nucleus (Gabella 1981) and based on nuclear diameter, enteric glia are estimated to be approximately 10 times smaller in size than enteric neurons. However, this estimate does not incorporate the multitude of processes extending from the glial cell body. Enteric glia are “molded” to the surface of enteric neurons but unlike the satellite cells of sympathetic ganglia, enteric glia do not form capsules around enteric neurons and large areas of enteric neurons have no glial coverage (Gabella 1981) . Likewise, glia within interganglionic fiber tracts rarely ensheath an individual neurite and most often only act to separate nerve processes into groups. Thus, most nerve processes are in direct membrane-to-membrane contact.
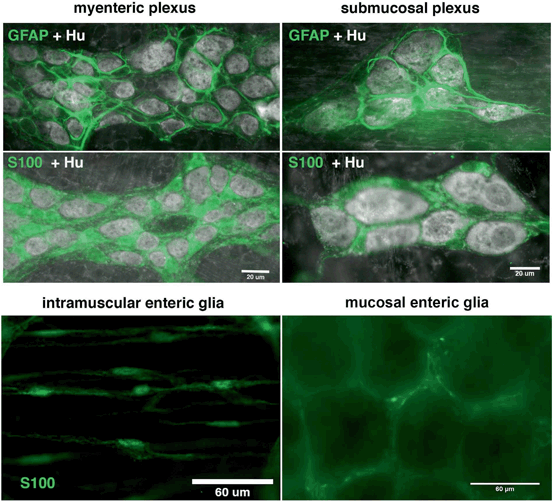
Fig. 21.2
Morphology of the main populations of enteric glia in the mouse colon. GFAP or S100 immunoreactive enteric glia (green, GFAP or S100 labeling where indicated) surround enteric neurons (Hu immunoreactive, grayscale) in the myenteric and submucosal plexuses of the mouse colon. Examples of S100 immunoreactive enteric glia (green) are shown within the smooth muscle coats (intramuscular enteric glia) and the mucosa (mucosal enteric glia)
Although small in size, the number of enteric glia within ganglia is often touted as being far greater than neurons. However, the exact ratio of enteric glia to neurons varies widely between species and increases with animal size. In mice, enteric neurons and glia are present at approximately a 1:1 ratio within myenteric ganglia and neurons actually outnumber glia in the submucosal plexus by almost a 2:1 ratio (Gabella and Trigg 1984) . In guinea pigs and rabbits enteric glia outnumber myenteric neurons by approximately 2:1 but are nearly equal to neuron numbers in the submucosal plexus. Sheep have over 4 times as many myenteric glia as neurons and 1.5 times as many submucosal glia than neurons (Gabella 1984) . Humans have the highest ratio of enteric glia to neurons in any species examined to date and enteric glia outnumber human enteric neurons by a ratio of up to 7:1 in myenteric ganglia and 2:1 in submucosal ganglia (Hoff et al. 2008) . Thus, enteric glia are by far the most abundant cell type within the human enteric nervous system.
The molecular makeup of enteric glia appears largely similar to astrocytes and enteric glia are commonly identified by expression of the astrocytic markers glial fibrillary acidic protein (GFAP) and S-100beta (Ferri et al. 1982; Jessen and Mirsky 1983) (Fig. 21.2). All glia within enteric ganglia are thought to express GFAP and S-100beta but expression of these glial markers may vary between enteric glial subtype, species, gut region and glial state in disease (discussed later). The transcription factors Sox8/9/10 are reportedly expressed by all enteric glia and immunoreactivity for Sox8/9/10 has been successfully used to quantify glial numbers in myenteric and submucosal ganglia (Hoff et al. 2008) . Electrophysiological recordings from enteric glia indicate astrocytic-like membrane properties including low input resistance, large passive currents in response to voltage steps and inwardly-rectifying potassium and sodium currents (Hanani et al. 2000) , indicating that a similar composition of cell membrane channels are expressed in both astrocytes and enteric glia.
21.4 Functions of Enteric Glia
As stated earlier, the term “enteric glia” encompasses multiple unique cell types along the length of the gut and through its thickness. All populations of enteric glia derive from a common pool of neural-crest-derived progenitors (Laranjeira and Pachnis 2009) and many maintain expression of common markers such as S100 (Ferri et al. 1982) , GFAP (Jessen and Mirsky 1983) and the transcription factors SOX8, SOX9 or SOX10 (Hoff et al. 2008) into adult life . However, the phenotype of enteric glia is heavily influenced by external cues (Dulac and Le Douarin 1991; von Boyen et al. 2004) and the unique microenvironments throughout the gut wall promote the development of several morphologically and functionally distinct populations of enteric glia.
21.4.1 Glial Regulation of Barrier Function
In the mucosal compartment, a population of enteric glia lies just below the epithelial lining of the gut wall in contact with epithelial cells, nerve fibers and blood vessels (Neunlist et al. 2013) . Increasing evidence suggests that enteric glia at the level of the mucosa regulate gut barrier function by releasing factors that modulate the transcriptome of gut epithelial cells. Maintenance of gut barrier function is essential to limit invasion by the multitude of bacteria within the gut lumen as well as viruses or other foreign substances. Indeed, an increase in epithelial permeability is associated with many gastrointestinal diseases and is regarded as a contributing event to the onset of disease pathology in the gut. Glia influence the differentiation, adhesion, migration and proliferation of epithelial cell monolayers in culture by releasing factors that include S-nitrosoglutathione (GSNO) (Savidge et al. 2007) , 15-deoxy-Δ12,14-prostaglandin J2 (15d-PGJ2) (Bach-Ngohou et al. 2010) , transforming growth factor (TGF)-β1 (Neunlist et al. 2007) and proepidermal growth factor (proEGF) (Van Landeghem et al. 2011) . Given the propensity of glia to change under culture conditions, it is unclear if mucosal glia maintain similar roles in vivo but conditional ablation of enteric glia does increase epithelial permeability (Savidge et al. 2007) . Thus, it seems that a loss of glial support of the epithelial barrier has the potential to promote, or exacerbate disease processes in the gut.
21.4.2 Enteric Glia and Neurotransmission
Enteric glia within the enteric nerve plexuses are morphologically (Jessen and Mirsky 1983) , and likely functionally similar to astrocytes in the central nervous system . Enteric glia are associated with neurons in submucosal ganglia, myenteric ganglia and the nerve bundles connecting ganglia. Glia within enteric ganglia display a star-shaped morphology that resembles protoplasmic astrocytes with many irregularly branched processes surrounding enteric neurons (Hanani and Reichenbach 1994) . The elongated, fibrous glia within interconnecting nerve fiber bundles are more similar morphologically to the fibrous astrocytes of CNS white matter tracts and these glia populations likely share similar functions.
Glia within enteric ganglia are actively involved in homeostatic mechanisms that maintain enteric neurotransmission (Gulbransen and Sharkey 2012) . Intraganglionic glia supply enteric neurons with essential precursors for the synthesis of neurotransmitters including nitric oxide (NO) (Aoki et al. 1991; Nagahama et al. 2001) , glutamate and γ-aminobutyric acid (GABA) (Jessen and Mirsky 1983) . NO has well characterized functions in both the physiology and pathophysiology of the enteric nervous system but the physiological significance of glutamate and GABA remain unclear. Interestingly, dysregulation of either glutamate or NO participates in disease by promote the death of enteric neurons (Kirchgessner et al. 1997) . In health, enteric glia supply neurons with antioxidants that limit death caused by oxidative stress (Abdo et al. 2010; Abdo et al. 2012) and mounting evidence suggests that enteric glia normally function to sustain enteric neurotransmission by regulating the bioavailability of neuroactive substances in the extracellular environment. Enteric glial cell surface enzymes such as the ectonucleotidase, nucleoside triphosphate diphosphohydrolase 2 (Braun et al. 2004; Lavoie et al. 2011) and transporters including those for peptides (Rühl et al. 2005) and GABA (Fletcher et al. 2002) play a key role in the removal of neuroactive compounds surrounding enteric neurons. Likewise, glial channels maintain neurotransmission and prevent excitotoxic neuron death by regulating, and buffering extracellular potassium (Hanani et al. 2000; Costagliola et al. 2009) . Taken together, this evidence suggests that glia are essential points of homeostatic control in enteric ganglia and that disruption of glial functions could alter gastrointestinal physiology by altering enteric neurotransmission or permitting enteric neuron death.
In addition to the passive, homeostatic mechanisms discussed above, recent evidence suggests that enteric glia actively participate in enteric neurotransmission and are capable of detecting and in turn, modulating the activity of enteric neurons. Enteric glia a diverse assortment of receptors that permits glia to “listen” to neuronal conversations and initiate intracellular signaling mechanisms in response to neurotransmitters released from enteric neurons (Gomes et al. 2009; Gulbransen and Sharkey 2009; Gulbransen et al. 2010, 2012; Broadhead et al. 2012) . ATP and related purines are the most ubiquitous signaling molecules involved in enteric neuron-to-glia transmission in vitro (Kimball and Mulholland 1996; Gomes et al. 2009) and in situ (Gulbransen and Sharkey 2009; Gulbransen et al. 2010; Broadhead et al. 2012) but enteric glia have the potential to detect a number of neuroactive substances including norepinephrine (NE) (Nasser et al. 2006) , glutamate (Nasser et al. 2007), thrombin (Garrido et al. 2002) , lipid signaling molecules (Segura et al. 2004a; Segura et al. 2004b) , serotonin (Kimball and Mulholland 1996; Boesmans et al. 2013) , bradykinin (Kimball and Mulholland 1996), histamine (Kimball and Mulholland 1996) and endothelins (Zhang et al. 1997) . Precisely how glial signaling mechanisms affect neurotransmission is still debated but recent evidence demonstrates that glial responses are associated with neuron activity during patterns of activity that underlie physiological gut function (Broadhead et al. 2012) .
The tight association of glial responses with neuron activity raises the possibility that enteric glia function as a feedback loop to modulate enteric neurotransmission. Conceivably, enteric glia could modulate enteric neurotransmission by either utilizing the mechanisms described above to regulate the availability of neurotransmitters or by releasing neuroactive substances upon stimulation in a process called gliotransmission. Although few studies have addressed the potential of enteric glia to release neuroactive substances, the available evidence does support the notion of enteric gliotransmission. Indeed, calcium responses in enteric glia can initiate the release of ATP in vitro (Zhang et al. 2003) and ATP and its purine metabolites are well known neurotransmitters/neuromodulators in the enteric nervous system (Galligan and Bertrand 1994) . In vitro, propagation of calcium responses between enteric glia depends on their release of ATP through hemichannels (Zhang et al. 2003) . We recently found that this process is maintained in situ and that substances released through connexin-43 hemichannels mediate intercellular communication in enteric glia (McClain et al. 2013) . Importantly, we found that gastrointestinal transit was delayed when connexin-43 was genetically ablated from glia. These results provide strong evidence that enteric glia have the potential to release gliotransmitters and that gliotransmitters influence gut function through actions on enteric neurons.
How other populations of enteric glia outside enteric ganglia interact with enteric neurons is currently unknown but enteric glia are associated with nerve fibers within the smooth muscle coats of the gut wall. Unfortunately, very little is known about this class of cells but they are morphologically similar to nonmyelinating Schwann cells . Intramuscular enteric glia do express receptors for neuroactive substances (Vanderwinden et al. 2003) but the interactions between nerve fibers and enteric glia in the muscle coats are still unexplored.
In summary, a growing body of evidence points toward enteric glia as important regulators of physiological gut functions at the level of the mucosa and within the enteric nerve plexuses. Importantly, these are two sites often associated with pathological processes in the gastrointestinal tract. In the following sections, we will discuss the evidence implicating enteric glia in gastrointestinal disease and how enteric glial alterations could contribute to disease pathology.
21.5 Enteric Glia in Gut Pathology
An emerging concept in gastroenterology is that inflammation within the enteric nerve plexuses is a key-contributing factor to organ dysfunction in many gastrointestinal diseases . A growing body of evidence supports the notion that enteric glia are altered by, and contribute to, pathological conditions in the gastrointestinal tract. Our current understanding of how enteric glia participate in gut pathophysiology stems mainly from histological studies but accumulating evidence suggests that specific glial changes can promote gastrointestinal disease and dysfunction .
21.5.1 Gastrointestinal Diseases Associated with Alterations to Enteric Glia
Glial alterations are associated with a wide array of diseases that affect the gastrointestinal system. These diseases can be broadly grouped as those primarily affecting the gastrointestinal tract (Primary) or diseases where gastrointestinal complications arise with other disease processes (Secondary). We begin this section by introducing the evidence for glial involvement in specific gastrointestinal diseases and then discuss the possible mechanisms by which glia could participate in pathology.
21.5.1.1 Primary Intestinal Diseases
Inflammatory bowel disease
Approximately 1.4 million people in the United States are affected by the Inflammatory bowel diseases (IBDs) , Crohn’s and ulcerative colitis (Loftus 2004) . Although both diseases are characterized by chronic or recurring gastrointestinal inflammation, the pathology and clinical manifestations of these diseases differ significantly (Table 21.1). Inflammation in ulcerative colitis is restricted to the colon, while inflammation in Crohn’s disease may occur anywhere along the GI tract from the mouth to the anus. These differences in inflammatory patterns result in slightly different clinical symptoms of the two diseases. The major symptoms of the IBDs are those typical of diseases of GI distress and include diarrhea, abdominal cramps, ulcers and constipation. Other less common symptoms include fever, weight loss and loss of appetite. Ulcerative colitis patients usually suffer from rectal bleeding, a symptom rarely seen in Crohn’s disease patients.
Table 21.1
Differences between Crohn’s disease and ulcerative colitis
Crohn’s disease | Ulcerative colitis | |
---|---|---|
Location | Entire GI tract | Colon only |
Characterization of inflammation | Patchy inflammation- Healthy regions adjacent to inflamed regions, skip lesions | Continuous area of inflammation |
Depth of inflammation | Transmural—changes to all layers of GI tract | Changes restricted to innermost layer primarily |
Clinical symptoms | Ulcers, Fever, Weight loss | Bloody diarrhea |
Cytokine response | Mixed Th1-Th17 response: IL-17, IL-23, IL-1β, TNF-α, IL-12, IFN-γ | Th2-like response: IL-13, IL-5, IL-4 |
The cytokine profile driving inflammation in Crohn’s disease and ulcerative colitis differs considerably. Ulcerative colitis is characterized by a Th2-like immune response with increases in interleukins 3, 4, 5 and 13 (IL-3, IL-4, IL-5 and IL-13) while Crohn’s disease features an increase in the Th1 response associated cytokines IL-12 and Interferon-γ (IFN-γ), which results in increases in interleukin 1β (IL-1β) and tumor necrosis factor α (TNFα) (Sartor 1995; Bamias et al. 2011) .Alterations to enteric glial survival, morphology and molecular makeup are now recognized as consistent features of IBD . However, the nature of glial alterations seems to differ between Crohn’s and ulcerative colitis. Assessment of GFAP content by Western Blot, enzyme-linked immunosorbent assay (ELISA), or immunohistochemistry all demonstrate a significant reduction in GFAP protein levels in non-inflamed regions of colon from individuals with Crohn’s disease but no change in non-inflamed regions during ulcerative colitis (Cornet et al. 2001; von Boyen et al. 2011) . In contrast, GFAP levels were significantly elevated in areas of active inflammation in Crohn’s disease and ulcerative colitis, although to a lesser extent in Crohn’s (Cornet et al. 2001; von Boyen et al. 2011) . Quantitative assessment S-100 immunoreactivity also showed a significant reduction in Crohn’s disease samples as compared to controls (Cornet et al. 2001) . These differences may reflect the influence of the differing cytokine profiles in the diseases and possibly indicate differing glial roles in the two distinct diseases.
In animal models of IBD, glial alterations are recognized as coinciding with intestinal disease. Cornet et al. (2001) used an autoimmune targeting strategy to ablate enteric glia and found that glial ablation resulted in fulminant jejuno-ileo-colitis and premature death. Other animal models of IBD , including dextran sodium sulfate and trinitrobenzene sulfonic acid, demonstrate that colonic inflammation alters glial receptor expression (Nasser et al. 2007) , enzyme expression (Green et al. 2004) , and increases glial proliferation (Joseph et al. 2011) . Whether glial changes reflect inflammatory state or actively promote inflammation is still debated and evidence for both possibilities is discussed below.
Functional Gastrointestinal Disorders
In addition to the IBDs, evidence for glial alternations are becoming apparent in a number of other disorders causing gut dysfunction. Termed functional gastrointestinal disorders, these syndromes are characterized by alterations in gastrointestinal function with few associated pathological changes. Irritable bowel syndrome (IBS) is described a disease of unknown pathophysiology that is characterized by symptoms of GI dysfunction including diarrhea and constipation. Individuals with IBS experience altered gut function but structural changes to the gut wall are not readily apparent (Törnblom et al. 2002) . However, closer examination of tissue from IBS patients using immunohistochemical analysis studies demonstrates evidence of inflammation and enteric neuropathy within enteric ganglia (Törnblom et al. 2002) . Enteric glia are thought to play a key role in enteric neuroprotection (Cornet et al. 2001; Abdo et al. 2010; De Giorgio et al. 2012) and are altered by inflammation (see above). Although detailed assessment of enteric glia in IBS has yet to be performed, the presence of enteric ganglionitis with IBS strongly suggests that enteric glia are involved in the mechanisms of IBS pathology.
Intractable slow-transit constipation (STC) refers to a particularly severe form of constipation. Patients tend to be resistant to typical therapies (Schiller 2001) and often require surgery for relief from symptoms (Bassotti et al. 2003) . The most prominent hypothesis explaining altered propulsion and peristalsis in STC is that the underlying mechanism involves alterations to the ENS , including enteric neuron dysfunction or death. In support, immunohistochemical analysis demonstrates an increase in the number of apoptotic neurons in tissue from STC patients (Bassotti et al. 2006) . Further, the density of both enteric neurons and glia is significantly reduced (Bassotti et al. 2006) . These alterations suggest that enteric glial alterations are associated with STC and raise the possibility that glial changes participate in mechanisms leading to altered motility.
Chronic idiopathic intestinal pseudo-obstruction (CIIP) is a syndrome that presents the symptoms of GI tract obstruction, although no physical blockage is present. Symptoms of CIIP are similar to those that would be observed with GI obstruction and include constipation, abdominal pain, nausea and vomiting. While the etiology of the disease is unknown, secondary forms of the disease have been observed with the presence of infectious viruses such as the JC virus. Current evidence indicates that glial density is significantly reduced in patients with CIIP (Selgrad et al. 2009) . Interestingly, the remaining glial cells contained the JC Virus. These results raise the intriguing possibility that the JC virus acts preferentially on enteric glial to cause pathology in CIIP.
Diverticular disease (DD) or diverticulosis is defined as the formation of protruding sacs or pockets within the colon wall. These diverticula are formed when there is an area of weakness in the colon wall, through which the mucosal layer can bulge. Diverticular disease is typically asymptomatic however, damage or inflammation of the diverticula can result in serious complications such as rectal bleeding, GI tract infection, GI tract blockage and colon or diverticula rupture. As with a number of other functional GI disorders, the etiology and pathology of diverticular disease are unknown but morphometric analysis of ENS plexuses indicates a significant decrease in enteric neuron and glial cell density in DD patients (Wedel et al. 2010) .
Necrotizing Enterocolitis
Necrotizing enterocolitis (NEC) is the most common and serious gastrointestinal disorder encountered among hospitalized preterm infants. As the name suggests, NEC is characterized by infection and inflammation that leads to the destruction of the terminal ileum and proximal colon. The causal nature of NEC is unknown but an ischemic event in the intestine is hypothesized to play a key role in disease pathology (Watkins and Besner 2013) . Histological evidence suggests that myenteric and submucosal neurons and glia decline in NEC (Wedel et al. 1998) . Interestingly, the loss of neurons and glia was most predominant at sites where ischemic lesions appear first. In animal models, intestinal ischemia reperfusion causes alterations in enteric glial morphology and disrupted glial morphology is associated with a decline in enteric neuron numbers (Thacker et al. 2011) . These intriguing results raise the possibility that alterations to enteric glia initiated by ischemic events in the neonatal gut contribute to the pathology of NEC .
Infectious Diseases
Infection is a well-recognized cause of inflammation in the gastrointestinal tract. Evidence supporting a role for enteric glia in gastrointestinal infections is mainly based on studies of Chagas disease: an infection by the protozoan Trypanosoma cruzi that commonly occurs in rural Latin America. Like the IBDs , Chagas disease causes systematic inflammation that can affect multiple organ systems. In the acute phase of the disease, which typically occurs within weeks of infection, symptoms are usually mild and may include fever, inflammation at the site of infection and gastrointestinal disturbances including diarrhea, vomiting and loss of appetite. The chronic phase develops a few months after infection and, in about 30 % of individuals, is characterized by chagasic megacolon; an abnormal dilation of the GI tract. In Chagas disease, it is hypothesized that inflammatory destruction of the plexuses of the enteric nervous system contributes to the pathogenesis of chagastic megacolon.
Glial alterations are associated with the pathology of Chagas disease and chagastic megacolon. Immunohistochemical analysis of tissue from Chagas’ patients reveals a significant decrease S-100 immunoreactivity (da Silveira et al. 2009) . This decrease in glial S-100 is evident in both dilated and non-dilated colon segments and is associated with a Chagas-mediated enteric neuropathy. However, GFAP expression was significantly higher in non-dilated segments than that in dilated colon segments. Thus, while glial alterations are clearly involved in the pathology of Chagas, it is unclear whether these changes indicate an alteration in glial numbers or a change in expression of glial markers.
JC virus is a polyomavirus which can infect both the central and enteric nervous system. JC virus infection in the brain causes progressive multifocal leuokoencephalopathy which is characterized by multiple inflammatory lesions throughout the brain. As indicated above, JC virus infection in the ENS is recognized as a secondary cause of CIIP. In CIIP, JC virus infects enteric glial cells (Selgrad et al. 2009) . Consequently, glial cell density in JC virus-associated CIIP was found to be decreased compared to control samples.
21.5.1.2 Secondary Intestinal Diseases
Type II diabetes
The incidence of type II diabetes has risen to the extent where diabetes is now the first metabolic disease to be classified as an epidemic by the world health organization. Gastrointestinal dysfunction is common in persons with diabetes and gastrointestinal complications can affect one or multiple regions of the gastrointestinal tract. Nausea, vomiting, abdominal pain, gastroparesis, constipation and diarrhea are commonly seen in Type II diabetes patients (Enck and Frieling 1997) and changes to the ENS are hypothesized to contribute to these symptoms. Mice ingesting a high-fat diet (an established model for Type II diabetes) experienced enteric neuron loss in the duodenum, along with an increase in apoptotic neurons (Stenkamp-Strahm et al. 2013b) . Immunohistochemical classification identified that inhibitory motor neurons were preferentially lost in diabetes associated enteric neuropathy. This neuropathy was accompanied by a decrease in the glial cell network during high-fat mediated diabetes in mice (Stenkamp-Strahm et al. 2013a) .
Parkinson’s Disease
Parkinson’s disease (PD) is typically thought of as a disease that primarily affects neurons in the basal ganglia but a current hypothesis proposed by Braak and co-workers postulates that PD originates in the gastrointestinal tract (Braak et al. 2006) . The formation of Lewy bodies is considered an important pathological feature of PD (Fujiwara et al. 2002) . (Braak et al. 2003) noted that the first appearance of Lewy pathology, during the initial stages of PD, is within the ENS and dorsal motor nucleus of the vagus nerve. Further support comes from findings showing that alpha-synuclein immunoreactive Lewy bodies are present within the ENS of Parkinson’s patients (Pouclet et al. 2012; Shannon et al. 2012) . Similar to the effects of PD on skeletal muscle motor control, one of the most common symptoms of PD is gastrointestinal dysfunction including constipation, impaired gastric emptying and dysphagia (Edwards et al. 1991; Pfeiffer 2011) . Further, disease severity in PD correlates with increased expression of pro-inflammatory cytokines increases in the colon, indicating that inflammation within the gut predicts disease activity and progression (Devos et al. 2013) . Altered expression of enteric glial markers is also associated with Parkinson’s disease severity. Devos et al. (2013) found that the expression of GFAP and Sox-10 both increased in colonic biopsies from individuals with PD. However, S-100 levels remained unchanged and GFAP and Sox-10 expression were not correlated with enteric Lewy pathology. A central tenant of Braak’s hypothesis is that altered barrier function contributes to disease initiation. Enteric glia are known to potently regulate barrier function so the finding that glial alterations are associated with disease progression raises the intriguing possibility that enteric glia are involved in the initiation of PD .
21.5.2 Enteric Glial Response to Pro-inflammatory Cytokines and Inflammatory Mediators
A common thread associating the pathology of intestinal diseases is inflammation . Inflammation is a key process driving pathology in the gastrointestinal tract and mounting evidence suggests that enteric glia are both a site of action of, and producers of, inflammatory mediators within the ENS . This reactive gliosis is characterized by glial cell hypertrophy, increases in the synthesis of GFAP and other proteins and secretion of cytokines (Rühl et al. 2004) .
An altered cytokine profile and the presence of other inflammatory mediators is intimately linked to disease pathology in IBD . Specifically, Crohn’s disease and ulcerative colitis are characterized by increases in pro-inflammatory cytokines (Nikolaus et al. 1998) . These diseases can be differentiated between based on the pro-inflammatory cytokines they upregulate, supporting the idea of different pathologies for the diseases. Ulcerative colitis is generally characterized by a Th2-like response with increases in IL-4 and IL-13 while Crohn’s disease is associated with a Th1 response predominated by IL-1β andTNFα (Sartor 1995) . Similar to Crohn’s, IL-1β and TNFα are upregulated in NEC, a common condition in preterm infants that destroys of segments of bowel. The effect of the proinflammatory cytokines IL-β and TNF-α on enteric glia provides some evidence of a role for enteric glia in the pathology of IBDs.
In vitro treatment of enteric glia with the pro-inflammatory cytokines IL-1β and TNF-α or lipopolysaccharide (LPS) induces an increase in GFAP expression (von Boyen et al. 2004) . In vivo, LPS initiates an immune response within the GI tract mediated by the proinflammatory cytokines TNF-α and IL-1β, raising the possibility that the effect of LPS could be secondary to cytokine release. However, because these studies were performed in vitro, they suggest that LPS has a direct effect of glial GFAP content. The increase in GFAP expression was accompanied by both an initiation of expression of GFAP in GFAP-negative glial cells and further upregulation of expression in already GFAP-positive cells (von Boyen et al. 2004) . An increase in GFAP-positive glia was also observed in tissue from patients with ulcerative colitis and Crohn’s disease, supporting the in vitro data above (Cornet et al. 2001) . However, the role of increased GFAP expression in the inflammatory process of the IBDs is not fully understood. Indeed, mice lacking GFAP develop and reproduce normally (Pekny et al. 1995) . Increased GFAP expression in CNS astrocytes is proposed to act to isolate inflammatory regions (Rühl et al. 2001a) . Thus, upregulation of enteric glial GFAP expression may play a similar role in the ENS and act as a defense mechanism of the ENS to protect against inflammation.
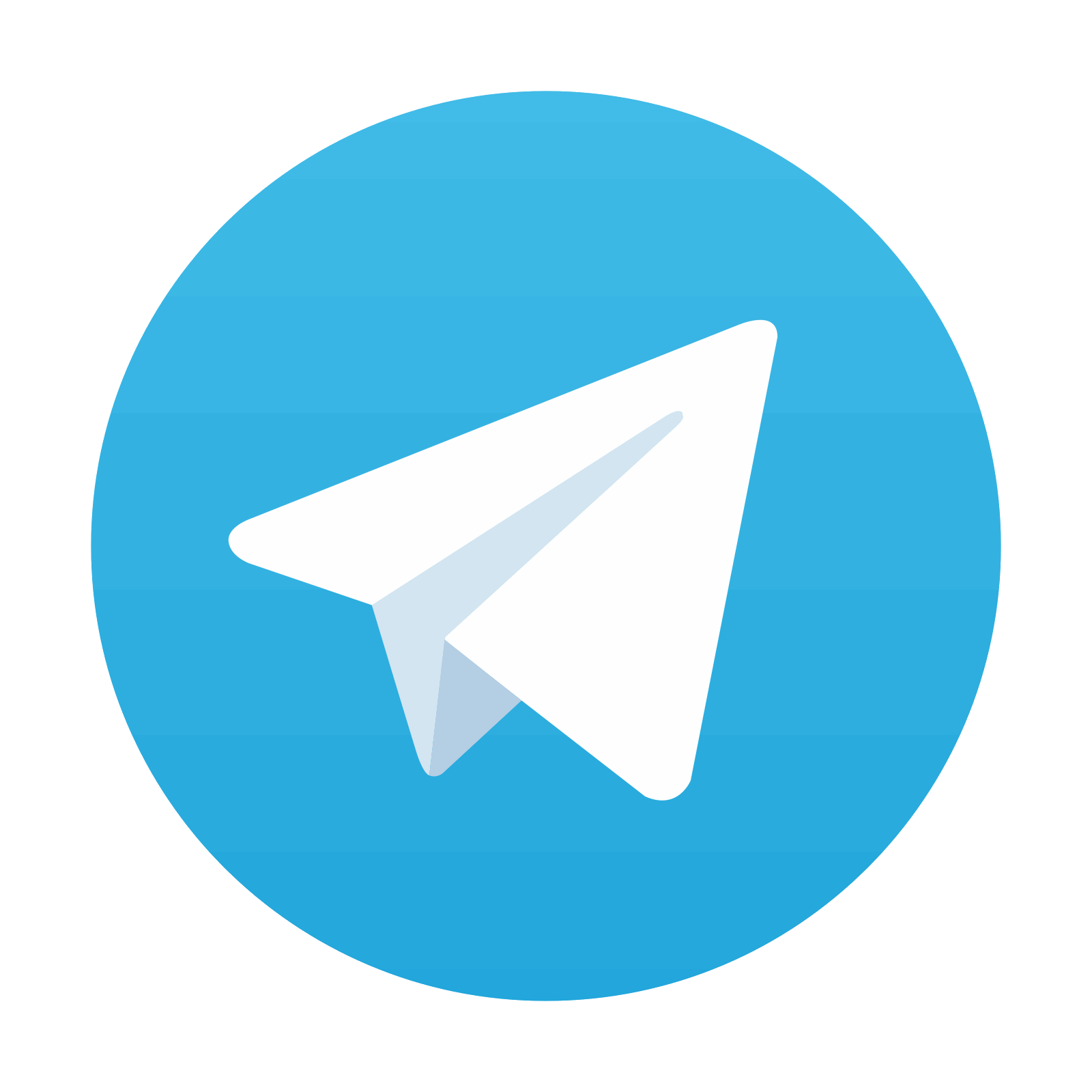
Stay updated, free articles. Join our Telegram channel

Full access? Get Clinical Tree
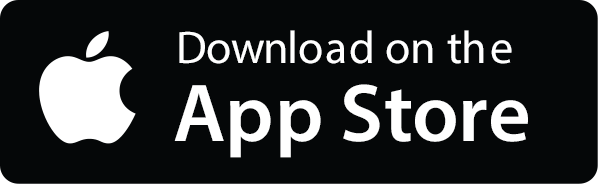
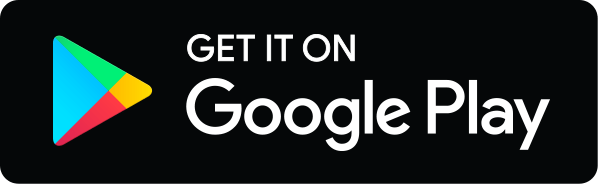