Array CGH
Children
Adults
<4 years
>4 years
Balanced profile
Few imbalances
Few imbalances
Extensive imbalances
Gains
1q, 7, 9q
7, 9, 12, 5, 18, X, 2
Losses
22, 3, 9p, 13q, 6q, 1p, 17, 6
22/22q, 10, 13q, 6, 14q
Witt et al. focused on posterior fossa ependymoma in 177 adults and children and identified two distinct molecular subtypes [12]. The subtype A occurred in younger patients (median of 2.5 years old) with relatively less genomic instability. The most frequent DNA copy number variants included gain of chromosome 1 and loss of chromosome 22. On the other hand, subtype B occurred in older patients (median of 20 years old) with extensive chromosomal aberrations. More recently, Mack et al. [13] and Parker et al. [14] found that supratentorial ependymomas carry an intrachromosomal translocation that creates a new tumor-driving gene, posterior fossa type A lack tumor-driving mutations, but has aberrant epigenetic modifications, and type B shows neither gene mutations nor epigenetic aberrations.
20.3 The Location of Ependymoma Influences Its Genetic Landscapes
The meta-analysis made by Kilday et al. revealed location-specific abnormalities as shown by CGHa [8]. Recent genome-wide expression analyses have revealed that despite histological similarities, ependymomas arising from different locations of the CNS are biologically distinct in terms of genetic aberrations and transcriptional profiles [2, 9, 11–20]. Table 20.2 recapitulates the main biological characteristics associated with tumor location reported in literature.
Table 20.2
Biological characteristics of pediatric ependymomas according to location
Location | Biological characteristics | Role | References |
---|---|---|---|
Supratentorial | Ch 9 deletion | Uncertain | |
ECM and cytoskeleton genes | Mesenchymal transition | [18] | |
EphB–ephrin and Notch signaling | Tumor stem cell expansion | ||
CyclinD1 | Increased proliferation rate | [2] | |
NEFL70, LHX2, FOXG1, TLX1 | Neuronal differentiation, better prognosis | [19] | |
Ch11 translocation (C11orf95–RELA fusion) | Involve in NF-kB signaling. Induces ependymoma-like tumors in mouse brain | [14] | |
Posterior fossa | Ch 1q gain | Worse prognosis | [8] |
Ch 9q33 – 34 gain at relapse | |||
PAX3, OGN, FMO1 | None identified | [15] | |
ZIC1,2,3,4 and LFNG | Unknown | [16] | |
ID1,2,4 | Glial stem cell marker | [2] | |
AQP1,3,4 | Angiogenesis and invasion | [2] | |
TNC overexpression | Worse prognosis | ||
CpG island methylator phenotype | Repression of differentiation genes | [13] | |
Spinal | Ch 7 and 9 gains | ||
Overexpression of HOX genes | Alteration of anteroposterior differentiation | ||
IGF1 | Increased cell growth |
20.3.1 Identification of Key Players in the Tumorigenesis and Progression of Pediatric Ependymomas
It is now clear that ependymomas from distinct locations will be initiated and will progress through different biological pathways. In 2005, Taylor et al. showed that supratentorial posterior fossa and spinal ependymomas have distinguishable gene expression signatures and proposed radial glia cells as the cell of origin for supratentorial and spinal cord ependymomas [2]. They showed also an upregulation of EphB–ephrin in the supratentorial group compared to the others. Neuronal differentiation has been recently shown to be characteristic of supratentorial ependymomas (68 % IHC positivity in supratentorial tumors and only 12.5 % for infratentorial ependymoma) with the positivity of NEFL70 (neurofilament light polypeptide) associated with a better prognosis [19]. On the opposite, RELN (reelin) and TN-C (tenascin C, an extracellular matrix protein of the stem cell niche), both belonging to the Notch1 gene signaling pathway, have been found significantly upregulated in infratentorial ependymoma [11, 12, 15–19].
To better understand the biological abnormalities underlying ependymoma progression, Puget et al. [11] analyzed 59 pediatric ependymoma by CGHarray including 33 tumors at the time of diagnosis and 26 at relapse. By comparing genomic abnormalities of these two groups of tumors, they observed a significant increase of chromosomal imbalances in relapsed ependymoma compared to those analyzed at the diagnosis, such as gain of the 9qter and 1q and loss of 6q. The presence of the 9qter gain was a sign of tumor aggressiveness as it was associated with more pejorative prognostic and occurred preferentially at relapse in children over 3 years and located in the posterior fossa. Two important genes located in this region, NOTCH1 and tenascin C (TN-C) were overexpressed in ependymoma compared to normal and fetal brain. Quantitative PCR analyses of the tumor samples highlighted overexpression of the Notch1 pathway target genes, such as DLL1 (ligand), and four effector genes (HES1, HEY1, MYC, and TNC) and the repression of FBXW7, a Notch1 repressor, involved in Notch1 ubiquitination (Fig. 20.1). Finally, mutations of NOTCH1 gene were identified both in TAD and in the HD domains. To date, this is one of the few candidate oncogenes identified in ependymoma [11, 12, 15–20].


Fig. 20.1
( a) Notch pathway landscape in pediatric ependymoma. This figure shows the RT PCR expression of some of the Notch pathway genes, both ligands and effectors. (b) Immunocytochemistry for HES1 (red dots) in pediatric ependymomas stem cells
To better understand the biological mechanisms involved in ependymoma relapse, Peyre et al. compared CGHarray and gene expression in 17 ependymoma tumors at the time of diagnosis and at subsequent relapses [18]. A tumor relapse signature based on the gene expression changes was established. They found a frequent overexpression of genes involved in the kinetochore (including ASMP, already described in the mechanisms of malignant glioma progression, and kinesin KIF11) and gene implied in neuronal development (CD133, Wnt and Notch signaling pathways). Conversely, metallothionein genes (including MT3, also known as neuronal growth inhibitory factor) were described as downregulated in more than 80 % of the recurrent ependymoma compared with the diagnosis. These data were confirmed by immunohistochemistry of ASMP and MT3 proteins on paired tumors at diagnosis and relapse on independent series. Some of these proteins could represent potential therapeutic option in the future.
20.3.2 Identification of Ependymoma Subgroups and Oncogenesis Pathways
Gilbertson’s team crossed the database of genomic abnormalities of human ependymoma to those of tumor models developed in mice [20]. They first identified subgroups of similar genomic alteration from 83 ependymomas (adults and children). Comparing their genomic abnormalities, they identified nine different subgroups, divided according to age and location. The transcriptome of human tumors was then crossed with those derived from murine neural stem cells (NSCs), isolated from different parts of the CNS, at different stages of development and having either a locus Ink4a/Arf, encoding for CDKN2A and CDKN2B, wild type or deleted. They showed that only the transcriptome of human supratentorial ependymoma which specifically amplifies the oncogene EPHB2 matched with NSCs isolated from Ink4a/Arf −/− mouse brain. Based on these results, they generated the first mouse model for supratentorial ependymoma. Mice transplanted with mouse NSCs Ink4a/Arf −/− and activating EPHB2 signaling developed brain ependymomas. Further comparative analysis of matched mouse models and a supratentorial ependymoma subgroup showed deregulation of genes implied in synaptogenesis, pinpointing disruption of this pathway as a critical event in the oncogenesis of this supratentorial subgroup. These data support strongly the hypothesis that ependymoma variants arise from specific combinations of susceptible NSCs and matched mutations. The driver mutations of the eight other subgroups they have described have yet to be identified.
Work from Witt et al. focused on posterior fossa ependymoma and identified two distinct molecular subtypes, each with unique gene expression signatures, different levels of genomic instability, and different prognosis [12]. These two subtypes were concordant with an independent patient cohort. The subtype A occurred in younger patients with lateral extension in the cerebellopontine angle with relatively less genomic instability. This subtype had enrichment of genes associated for signaling pathways for angiogenesis, PDGF, MAPK, EGFR, TGF-β, integrins, extracellular matrix (ECM) assembly, RAS/GTPase, and tyrosine kinase receptors. On the other hand, subtype B occurred in older patients and was more likely located in the spinal cord or the midline cerebellum with extensive chromosomal aberrations. This subtype had enrichment of genes associated with ciliogenesis, microtubule assembly, and mitochondria/oxidation metabolism. These findings were confirmed by immunohistochemistry of two markers of ECM signaling for subtype A, named TNC and LAMA2 (laminin alpha 2), and two markers of ciliogenesis signaling and microtubule assembly for subtype B, named KIF27 (kinesin family number-27) and NELL2 (neural epidermal growth factor-like 2). Some of these proteins could probably help to stratify ependymomas patients, although the cohort analyzed included both adults and children.
Two recent papers using whole genome and whole exome sequencing [13, 14] found that supratentorial ependymomas carry an intrachromosomal translocation that creates a new tumor-driving gene (C11orf95–RELA fusion on chromosome 11), whereas posterior fossa ependymomas type A and B did not reveal any recurrently mutated gene or translocation. Mack et al. did find increased DNA methylation of specific genes, as well as silencing of their expression, in type A, but not type B posterior fossa ependymomas. Indeed, they found H3K27me3 marks on many of the genes with DNA methylation in posterior fossa type A tumors.
20.4 Prognostic Biomarkers
In recent years, efforts have been made to search for optimal molecular markers for ependymomas, but the degree to which they could accurately predict outcome is limited for several of them. Our knowledge has been hampered by results of cohorts including both adult and pediatric ependymomas, and most of the putative prognostic biomarkers identified so far still lack validation in prospective cohorts. For instance, members of the receptor tyrosine kinase 1 (RTK1) family have been proposed as potential prognostic markers for pediatric ependymomas [21, 22]. It concerned ERBB2/ERBB4 and EGFR, but the results on mixed cohorts were not confirmed in subsequent pure pediatric series [21].
20.4.1 Chromosomal Imbalances
Several studies have revealed chromosome 1q gain as a pejorative prognostic marker in ependymoma [9, 10, 23, 24]. Some genes located within 1q locus have been proposed as potential candidate oncogene, such as DUSP12, members of the S100 family, and CHI3L1, but they have to be validated in large series of pediatric ependymomas. The recent results from Korshunov et al. on 122 ependymomas confirm and validate previous results [9]. They also pointed out a small group of poor prognosis associated with CDKN2A deletion and another group characterized by chromosomal imbalances associated with a better prognosis that have not been confirmed by another study yet. Considering that the cohort included both adult and pediatric ependymomas, all of the findings may not apply to children.
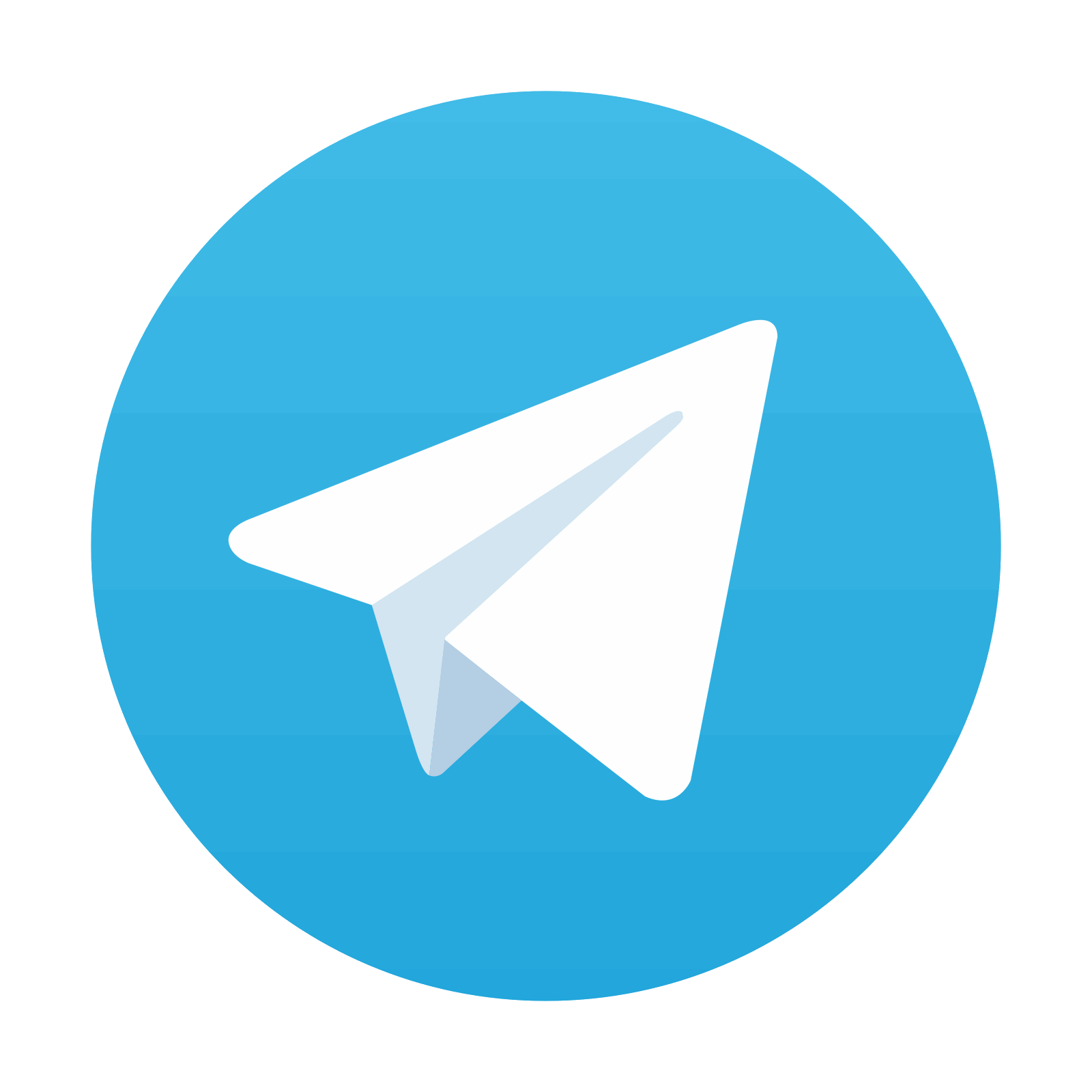
Stay updated, free articles. Join our Telegram channel

Full access? Get Clinical Tree
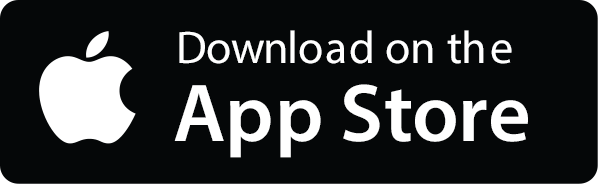
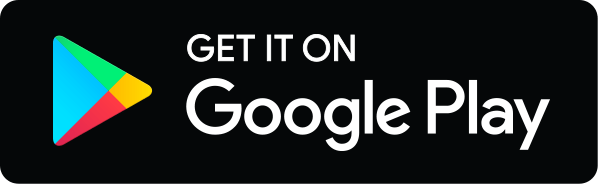