Authors
N of patients
M/F
Age
Method
Prevalence
Schon and Blau (1987) [27]
100
39/61
32 (mean)
Interview
18 %
Ottman and Lipton (1994) [25]
1,948
40 %/60 %
≥18
Structured telephone interviews + medical records review for 60 % of probands
24 %
Ito and Schachter (1996) [28]
162
82/80
19–65 (range)
Questionnaires mailed to the subjects + medical records review
NAa
Ito et al. (1999) [29]
109
36/73
38 ± 12b
Questionnaire + interview + medical records review
12.8 %
Velioglu and Ozmenoglu (1999) [18]
412
212/200
15–70 (range)
Interview with a standardized questionnaire
14 %
Leniger et al. (2001) [30]
341
154/187
40 ± 15 (mean)
Interview with a standardized questionnaire
18.2 %
Karaali-Savrun et al. (2002) [31]
135
80/55
≥10
Questionnaire administered to patients
14.8 %
Förderreuther et al. (2002) [32]
110
69/41
35.2 (mean)
Semi-standardized interview
10 %b
Ito et al. (2004) [33]
364
163/201
12–81 (range)
Structured interview with standardized questionnaire
8 %
Syvertsen et al. (2007) [34]
109
44/65
20–71 (range)
Questionnaire + semi-structured telephone interview
20 %
Kwan et al. (2008) [35]
227
98/129
36.0 ± 11.3 (mean)
Interview with standardized questionnaire + seizures and headache diary over the 3-month observation period + final interview
6.6%b
HELP Study Group (2010) [36]
597
348/249
≥13
Questionnaire at initial visit
12.4 %
Tonini et al. (2012) [11]
492c
154/338
≥18
Direct interview with questionnaire
18.3 %b
Duchaczek et al. (2013) [20]
201
106/95
≥18
Semi-structured interview
11 %
Winawer et al. (2013) [4]
730d
285/445
≥12
Telephone or in-person interview + medical record abstraction
25.2%e
Gameleira et al. (2013) [37]
304
141/163
4–88 range
Patients evaluated at the epilepsy clinic
32.9%f
Wang et al. (2014) [23]
1,109
607/502
≥18
Self-administered questionnaire + standardized semi-structured telephone interview
12.53 %
Peri-IH headaches are common in children with epilepsy, affecting 41 % [34, 38]. This headache, including migraine without aura (MO), appears to be more often associated with focal epilepsy, both temporal and extra temporal lobe. Furthermore, peri-IH and in particular pre-IH may be related to the epileptic discharge and may have lateralizing value [22, 39].
Pre-IHs have been reported to occur in 5–15 % of people with epilepsy. The underlying mechanisms of migraine attack with or without aura followed by a typical epileptic seizure are subject to debate. Whereas IHs are rarer (3–5 %), post-IHs are more common (10–50 % of cases) [1, 24]. IH is more frequent in children than in adults due to different clinical features both in epilepsy and in headache. Children with epilepsy are more likely to have autonomic attacks with long-lasting ictal autonomic manifestations, while adults more often have other sensory or motor ictal signs. It has been speculated that in many children, seizures mimic migraine, which can be easily recognized by an EEG recording [10, 40, 41]. The main involvement of posterior regions emerged in people with ictal epileptic headache (IEH) who were affected by focal symptomatic epilepsies [42]. IH cannot, however, be diagnosed without an EEG recording. Few case reports on EEG-documented IEHs in focal or generalized idiopathic epilepsy have been published [43, 44]. Of these, one describes IEH associated with nonconvulsive status epilepticus in an individual with eyelid myoclonia plus absences [45].
Young adults with a history of inter-IH are more likely to develop post-IH [46]. Post-IHs also frequently occur after seizures in predisposed children, interrupting their daily activities [10, 40, 41]. Young age at onset and long duration of epilepsy, drug-resistant seizures, generalized tonic-clonic seizures, and, possibly, occipital epileptic focus are additional risk factors. Post-IH is estimated to have a significant impact on the quality of life of people with epilepsy, but it is frequently undertreated. Case reports suggest that post-IHs respond to simple analgesic as well as to triptans [47].
3.4 Classification Concerns and Some Definition Proposals
In the 1960s, the term “migralepsy” was introduced to describe a condition in which an MA attack is followed by symptoms characteristic of epilepsy. To make a diagnosis of migralepsy, a temporal relationship between the migraine aura and a seizure event (within an hour) is necessary [48]. The definition of migraine-triggered epilepsy or migralepsy was included in the second edition of ICHD-II (under point 1.5.5) referring to epileptic seizures occurring during or within 1 h after a MA. Despite this, the term “migralepsy” has not been used uniformly in clinical practice, causing debate about the inadequacy of its definition in the current ICHD-II criteria [49–52]. People with IH are difficult to classify in ICHD-II and ICHD-3 beta and ILAE. The ICHD-II classification [19] defines “epileptic headache” as a headache with migraine features, while the individual also has a focal epileptic seizure. The term does not appear in the current ILAE and ICHD 3 beta classification [53]. In the ICDH-3 beta, code 1.4.4 refers to migraine aura-triggered seizure which is described as a seizure triggered by an attack of MA (Table 3.2).
Table 3.2
Migraine aura-triggered seizure definition from ICDH-3 beta
Diagnostic criteria include: |
A. A seizure fulfilling diagnostic criteria for one type of epileptic attack and criterion B below |
B. Occurring in a patient with 1.2 migraine with aura, and during, or within 1 h after, an attack of migraine with aura |
C. Not better accounted for by another diagnosis |
In the comments, it is reaffirmed that this phenomenon, sometimes referred to as migralepsy, is a rare event, originally described in patients with 1.2 migraine with aura and that evidence for association with 1.1 migraine without aura is still lacking |
The ILAE classification considers headache as a possible semiological ictal phenomenon among non–motor (point 2.0) features, describing it as a cephalic sensation (subclassified at sub-point 2.2.1.7) and not the only ictal expression of an epileptic seizure. Moreover, headache is not classified as a “pain” (among the “somatosensory” features at 2.2.1.1) or “autonomic” sensation (2.2.1.8), like signs of involvement of the autonomic nervous system, including cardiovascular, gastrointestinal, vasomotor, and thermoregulatory functions.
Over the last decade, IEH has been considered “a headache representing the only ictal phenomenon.” In fact, IEH is recognized as a headache (only an ictal epileptic manifestation) lasting from minutes to days with evidence of ictal epileptiform EEG discharges, which resolve after intravenous antiepileptic medications. This can be an epileptic manifestation per se, with onset and cessation if isolated, correlating with the scalp or deep EEG pattern [21, 54]. It has been suggested that “migralepsy sequence” may not exist at all and that the initial part of the “migralepsy sequence” may be simply an “IEH” followed by other ictal autonomic, sensory, motor, or psychic features [54]. The term “IEH” was coined to describe episodes of migraine/headache of epileptic origin when confirmed by EEG recording.
It has been suggested that this condition be described as “pure” or “isolated” epileptic headache (EH), when the headache/migraine is the only epileptic manifestation (requiring differential diagnosis from other headache forms) [21]. According to ICD-II, “hemicrania epileptica” (if confirmed) is a very rare variant of EH, characterized by the ipsilateral location of headache and ictal EEG paroxysms.
According to some authors, headache pain originates from trigeminal terminals in cerebral blood vessels; thus, headache should be classified as an “autonomic” sensation in the ILAE Glossary and Terminology. Headache should therefore be interpreted as the sole expression of an epileptic seizure and classified as an autonomic seizure. The debate continues and the matter needs further investigation [55].
3.5 Mechanisms Underlying Epilepsy and Migraine Comorbidity
Migraine attacks, in the same way as epileptic seizures, have been attributed to excessive neocortical cellular excitability. This state of brain hyperexcitability, which can be produced either by genetic factors or be acquired (due to head injury), increases the risk of both migraine and epilepsy, potentially leading to the comorbid association.
Cortical spreading depression (CSD) associated to migraine has been hypothesized as a link between headache (migraine) and epilepsy. CSD onset was described during the propagation of electrically provoked seizure discharges in the cerebral cortex and similarities in the ways in which CSD and seizures propagate were recorded [56]. CSD is characterized by a slowly self-propagating wave (2–6 mm/min) of sustained strong neuronal depolarization, responsible for transient (seconds), intense spike activity followed by neural suppression lasting for minutes [57].
Later, it was reported that seizures propagate rapidly, whereas the depolarization in CSD propagates slowly [58–60]. More specifically, evidence suggests that epileptiform activity associated with enhanced excitatory neurotransmission propagates at rates comparable to CSD, whereas epileptiform activity caused by reduced inhibition propagates much faster [61]. These two pathological phenomena were originally considered separate physiological events characterized by their different patterns of neuronal activities and characteristic ionic changes.
Despite these differences, both seizures and CSD are induced by similar mechanisms which include hypoxia, hypoglycemia, neural injury, high concentrations of K+, or Na+/K+ pump inhibition [62]. More recently human studies have observed this depolarizing phenomenon in a variety of pathological states, including epilepsy [63]. Based on these observations, models of neuronal membrane biophysics have recently been developed which can include a broad range of experimental observations, from spikes to seizures, supporting the hypothesis that seizures and CSD lay along a dynamic continuum of the neuronal membranes [64].
CSD has been considered the pathophysiological correlate of MA [65]. Imaging studies of people with MA have also suggested that the presence of CSD in silent areas is an underlying mechanism [66–68]. Experimental findings [69, 70] as well as functional neuroradiological investigations in humans [71] have suggested the potential role of CSD in determining trigeminovascular activation, describing it as the final common pathway responsible for the pain of migraine attacks. An increase in regional cerebral blood flow (rCBF) is associated with the depolarization phase, whereas a reduction in rCBF characterizes the phase of reduced neural activity [72]. In animal models, CSD can be triggered by focal stimulation (electrical, mechanical, high extracellular K+ or glutamate, persistent intracellular sodium influx, inhibition of the Na + −K+ ATPase pump) of the cerebral cortex, particularly occipital regions, while subcortical regions can also be involved [73, 74].
Experimental findings in 1990 had suggested that both seizure and CSD onset were due to the inability of the Na-K pump to regulate K+ extracellular concentrations [75]. These experimental findings were supported several years later by the identification of novel mutations involving Na+, K + −ATPase pump gene ATP1A2 associated with FHM and benign familial infantile convulsions and the demonstration of a monogenic defect in familial occipital lobe epilepsy associated with MA [76, 77]. Other complementary mechanisms seem to be involved in the ionic diffusions of both events (i.e., epileptic depolarization and CSD onset). In particular, synchronization in CSD has been related to “gap junctional” [78]. The gap junctions in synchronizing the human neocortical network are also involved in epileptiform activity [79, 80]. The calcium-sensitive current is able to promote both seizure-like discharges and CSD in experimental models [81]. In addition, glutamate is believed to be a critical mediator of hyperexcitability which has been demonstrated to be present in both focal seizures and migraine [82–85].
In focal epilepsy, seizure generation and spread is mediated by synaptically released glutamate acting on AMPA receptors, whereas triggering of CSD depends on NMDA receptors and its spread does not require synaptic transmission [86–88]. Therefore, the difference between the physiology of CSD and focal seizure activity could be due to CSD non-synaptic glutamate release from glia and the synaptic glutamate release from neurons and perhaps glia in the case of epileptic discharges [89–92].
Clinical research confirms that CSD (migraine) and cortical focal discharges (seizures) can facilitate each other. Migraines occasionally trigger seizures, which often initiate post-IH similar to migraines. The most compelling evidence for a relationship comes from FHM, where migraine and epilepsy can be caused by monogenic mutations involving CACNA1A, ATP1A2, and SCN1A genes [93]. CSD facilitates synaptic excitability and efficacy in human neocortical tissues, contributing to hyperexcitability of neocortical tissues in people with migraine. This is supported by the efficacy of some AEDs in preventing the occurrence of migraine attacks [94]. Electrophysiological findings have demonstrated that CSD can trigger epileptiform field potentials prevented by γ-aminobutyric acid (GABA)-mediated inhibition, suggesting that CSD increases neuronal excitability and facilitates synchronization of neuronal discharges in the presence of partial disinhibition of the neuronal tissues. This process might explain the occurrence of seizures in neurological disorders with partial impairment of inhibitory tone, such as epilepsy and brain ischemia [95].
3.6 Genetic Aspects of the Migraine-Epilepsy Comorbidity
MA and MO are a common familial condition. Different linkage and association studies have shown wide genetic heterogeneity, identifying susceptibility genes (ESR1, TNF, KCNK18, etc.) [96–98]. The presence of familial aggregation suggests a genetic background and probably an oligogenic inheritance which is also present in the common form of primary epilepsy (idiopathic generalized epilepsy). The number of identified susceptibility genes is rapidly growing and currently includes CACNA1H, CASR, CACNB4, GABRD, CLCN2, SLC2A1, GABRA1, and SLC12A5 [99–101]. At the moment it seems that susceptibility genes identified in migraineurs are different from those of people with idiopathic generalized epilepsy. However, powerful genetic studies (through whole genome association and exome studies) have not yet been performed on large cohort of people with both migraine and epilepsy. Instead, several genes associated with specific, monogenic forms of migraine have been reported to be associated with epilepsy, thus suggesting the existence of at least a common pathogenetic mechanism. Migraine and epilepsy are shared comorbidities in some people and related to specific mutations: (1) FHM to CACNA1A, ATP1A2, SCN1A, and episodic ataxia type 6 (EA6), due to mutation in the SLC1A3 gene (channelopathy); (2) mitochondrial disease to MT-TL1, MT-ND5, POLG, C10, or F2; and (3) vascular disease to NOTCH3, responsible for CADASIL (cerebral autosomal dominant arteriopathy with subcortical infarcts and leukoencephalopathy) [1, 5, 102, 103].
FHM
is a rare autosomal dominant syndrome characterized by hemiparesis during the aura phase of migraine and associated with mutation in one of three genes (CACNA1A, ATP1A2, SCN1A). A few studies have reported a prevalence of epilepsy about 7 % in FHM, but currently no conclusive data are available regarding a possible association between hemiplegic migraine and epilepsy. It is known that an increasing number of FHM with CACNA1A, ATPA1A2, and SCN1A mutations are associated with epilepsy.
CACNA1A
gene encodes for the pore-forming α1 subunit of human voltage-gated Cav2.1 (P/Q type) calcium channels [104]. Mutations in this gene were first identified in people with episodic ataxia type 2 (EA2) and in different families with FHM1 [104]. In 1997, the expansion of a CAG repeat, which predicted a coding for polyglutamine in the C-terminal coding region of the CACNA1A gene was identified in families with slowly progressive spinocerebellar ataxia (SCA6) [105]. Apart from the SCA6, which is associated with a specific dynamic mutation, genotype-phenotype correlation studies have identified an association between EA2 and loss-of-function mutations. Mutations described to date in people with HM have been missense, leading to a gain-of-function effect with enhanced Cav2.1 channel activity and subsequent increased intracellular calcium concentrations, which in turn causes CSD. On the other hand, a number of non-truncating mutations have been observed to cluster in the S5–S6 linkers and their borders even in EA2 [106, 107]. Genotype-phenotype correlations seem to be dependent on the functional effects of the mutations as well as the positions of the modified amino acids. Since no clear loss-of-function mutation (e.g., stop codon, frameshift, deletion) has been identified in people with FHM1 epilepsy, it is plausible that the gain-of-function effect is a specific mechanism for this endophenotype.
Regarding shared pathogenetic mechanisms, since CACNA1A variants may be responsible for repeated CSD, they could trigger both HM and epilepsy [94]. S218L produces a shift in activation leading to a gain of function, especially in the case of small depolarization [108]. This condition has been further supported by the higher incidence of recurrent CSD in S218L knock-in mice [109]. It has been reported that neutralization of gating charges in I and II S4 transmembrane domains of the Cav1.2 calcium channel (encoded by the CACNA1C gene) only slightly affected the equilibrium constant of voltage sensor transition [109]. When the Cav2.1 channel (encoded by the CACNA1A gene) lies in the first two S4 segments, it is highly unlikely that it plays a relevant role in the development of epilepsy since epileptic phenotypes have been found to be strongly associated when the mutations are located in the III and IV S4 segments. Therefore, people with F/SHM1 harboring such variants need to be closely followed up and managed.
ATP1A2
gene encodes the α2 subunit of the Na+, K+-ATPase [110], an integral membrane protein responsible for establishing and maintaining the electrochemical gradients of Na+ and K+ across the plasma membrane. Mutations in this gene were first identified in a large Italian family with FHM2 [111]. The functional analysis of nine different pathogenic mutations in this gene has been reported, and, overall, the data suggest that the disturbance of clearance of extracellular K+ by glial cells, thought to underlie FHM2, is due to a low turnover rate of the pump [112]. Other functional analyses have shown that some mutations cause total loss of function [111, 113, 114], while others reduce the catalytic turnover or change the kinetic characteristic of the pump [115, 116]. Glial and neuronal Na+, K+-ATPase play important roles in the clearance of extracellular K+ to prevent depolarization of neurons during high neuronal activity. Malfunctioning of the Na+, K+-ATPase pump may lead to neuronal hyperexcitability and facilitate both paroxysmal depolarizing shift (PDS) causing seizures and CSD causing migraine. These may occur in three synergistic events: elevation of extracellular K+ levels, accumulation of glutamate in the synaptic cleft, and an increase in intracellular Ca2+ concentration [117–119]. Missense mutations affecting the transmembrane domains can lead to both the perturbation of catalytic site functions and the modification of pump kinetic characteristics, and it is interesting to note that individuals carrying mutations within these domains are much more susceptible to developing epilepsy.
SCN1A gene
encodes the α1 subunit of the voltage-gated Na+ channel Nav 1.1. Mutations in this gene were first identified in people with familial epilepsy: generalized epilepsy with febrile seizures plus (GEFS+) [120] and Dravet syndrome, also known as severe myoclonic epilepsy of infancy (SMEI) [121]. A heterozygous mutation in the SCN1A gene has been identified in affected members of three European families with FHM3 [122]. Mutations can affect the protein structure in the intracellular, extracellular, and transmembrane domains. The genotype-phenotype correlation seems to depend mainly on the functional effect of the mutation rather than its position through the canal protein. Mutations leading to loss of function are mainly associated with SMEI, whereas gain-of-function mutations are mainly associated with GEFS+ or FHM3 [123].
In the case of FHM3-epilepsy comorbidity, experimental evidence suggests that a single mutation can have double functional effects. A study of a family with the T1174S (c.3521 C > G) SCN1A mutation associated with seizures and/or HM, applying a genetic and functional approach, reported that this variant induced divergent functional effects, loss of function or gain of function, a finding consistent with both epilepsy and HM phenotypes [124].
These genetic studies seeking to identify the genetic background of multifactorial or oligogenic diseases (such as the common forms of migraine and epilepsy) often fail to produce valid results due to high heterogeneity and environmental influences. More powerful studies on a larger cohort of people with migraine alone, epilepsy alone, and migraine-epilepsy comorbidity are needed to elucidate fully the possible shared genetic substrates. Study results from channelopathies suggest that mutation in a single gene that impairs neuronal or glial ionic homeostasis (i.e., sodium, potassium, and calcium) or affects GABAergic or glutamatergic systems or mitochondrial functions can lead to a spectrum of nervous system diseases with frequent migraine/epilepsy comorbidity.
3.7 Therapeutic Implications
Some AEDs are effective in the prevention of migraine [125–127]. A rationale for their use is the hypothesis that migraine and epilepsy share several pathogenic mechanisms [126, 127].
Imbalanced activity between excitatory glutamatergic transmission and GABAergic inhibition could play a role in both migraine and epilepsy. Abnormal activation of voltage-gated channels has been implicated in increased neuronal cortical excitability common to both disorders.
In people with epilepsy and any type of headache, especially migraine, AEDs for both pathological conditions are ideal treatments [128], whereas tricyclic antidepressants and neuroleptic drugs should be avoided as they may lower the epileptogenic threshold.
It is thought that AEDs exert their anticonvulsant action by targeting most of these pathogenic steps [125, 127, 129–131], but the reasons why only a few AEDs are effective in migraine prophylaxis are still unknown. It can be speculated that AEDs acting on multiple mechanisms of actions, such as valproic acid (VPA), topiramate (TPM), and gabapentin (GPT), are the best candidates for migraine prophylaxis. Accordingly, clear evidence in favor of an effective preventive action in migraine has been accumulated for both VPA and TPM. For GPT and lamotrigine (LTG), clinical data concerning their efficacy in migraine prophylaxis are still unclear.
TPM and VPA have been shown to be highly effective in preventing migraine, and both have been approved by the FDA for migraine prophylaxis [132]. Effective doses for migraine are generally lower than those for epilepsy, 880–1000 mg/day for VPA or 100 mg/day TPM.
The two drugs have also been shown to be effective for chronic migraine with and without medication overuse [133, 134]. LTG, a potent sodium channel blocker and glutamate receptor antagonist, has also been reported to be effective in people with MA (some of them with motor aura) but not in those affected by MO [135, 136]. Less robust evidence on efficacy is available for GPT and pregabalin (PGB) in episodic and chronic migraine forms. More promising results, which need to be confirmed, have been reported for levetiracetam (LEV) and zonisamide (ZNS). Other drugs such as phenytoin (PHT), oxcarbazepine (OCX), vigabatrin (VGB), and clonazepam (CNP) seem to be ineffective [137]. The findings of less headache in patients treated with carbamazepine (CBZ), recognized to be ineffective for migraine prophylaxis, in a study involving people with epilepsy and ictal and post-IH, may have been due to better controlled epilepsy in individuals who received this traditional first-choice treatment for partial epilepsy [34].
AEDs acting only via a single mechanism of action seem to be less effective in targeting migraine. Blockage of voltage-gated sodium channels alone, exerted by some AEDs (i.e., CBZ, PHT, OCX), seems to be insufficient to antagonize migraine crises, although this mechanism could be relevant in preventing epileptic crises. This does not imply that drugs such as VPA and TPM targeting Na+ currents (but not exclusively) could exert their effectiveness in preventing migraine, including MA, by counteracting Na+-dependent discharges and persistent Na+ conductance. Other targets for both drugs could be relevant for their prophylactic effect in migraine. This could also be relevant for HM, with and without an association with epilepsy [138].
AEDs targeting many pathogenic steps involved in migraine and epilepsy have been reported to be more promising in preventing MA and MO [127]. AEDs modulating high-voltage-activated (HVA) Ca(2+) channels used in migraine prevention with different evidence of efficacy affect both cortical and PAG neurons with different potencies. LTG and LEV, but not VPA, appear to be equally effective and potent in inhibiting HVA Ca(2+) currents in both neuronal populations, whereas TPM is more effective in blocking these currents in PAG neurons than in cortical pyramidal cells.
Drugs more effective on cortical neuronal activity should therefore theoretically be more effective for migraine aura than those for epilepsy. This is true for LTG which specifically affects only migraine aura, whereas date are lacking on LEV[139]. Additional targets of AEDs relevant for MA and MO other than epilepsy are ligand-gated channels, including both ionotropic and metabotropic glutamate receptors.
Inhibition of excitatory synaptic currents has been demonstrated for TPM, VPA, and GPT in experimental models suggesting that they might have an effect on CSD in both MA and HM, particularly in the presence of epilepsy. Another target is GABAergic transmission, as shown for VPA. Its potentiation could play a role in modulating CSD, therefore intervening in preventing not only MO but also MA [127].
It has also been demonstrated that the suppression of CSD in rats by chronic daily administration of TPM, VPA, as well as propranolol, amitriptyline, and methysergide is dose dependent [140, 141]. Magnetoencephalographic findings confirmed the reduction of cortical excitability in people with and without aura following 30-day treatment with VPA [142].
Chronic treatment with LTG appears to exert a marked suppressive effect on experimental CSD, which correlates with its rather selective action on the MA [143]. Therefore, this class of drugs could be considered the first-choice therapy when migraine and epilepsy are comorbid.
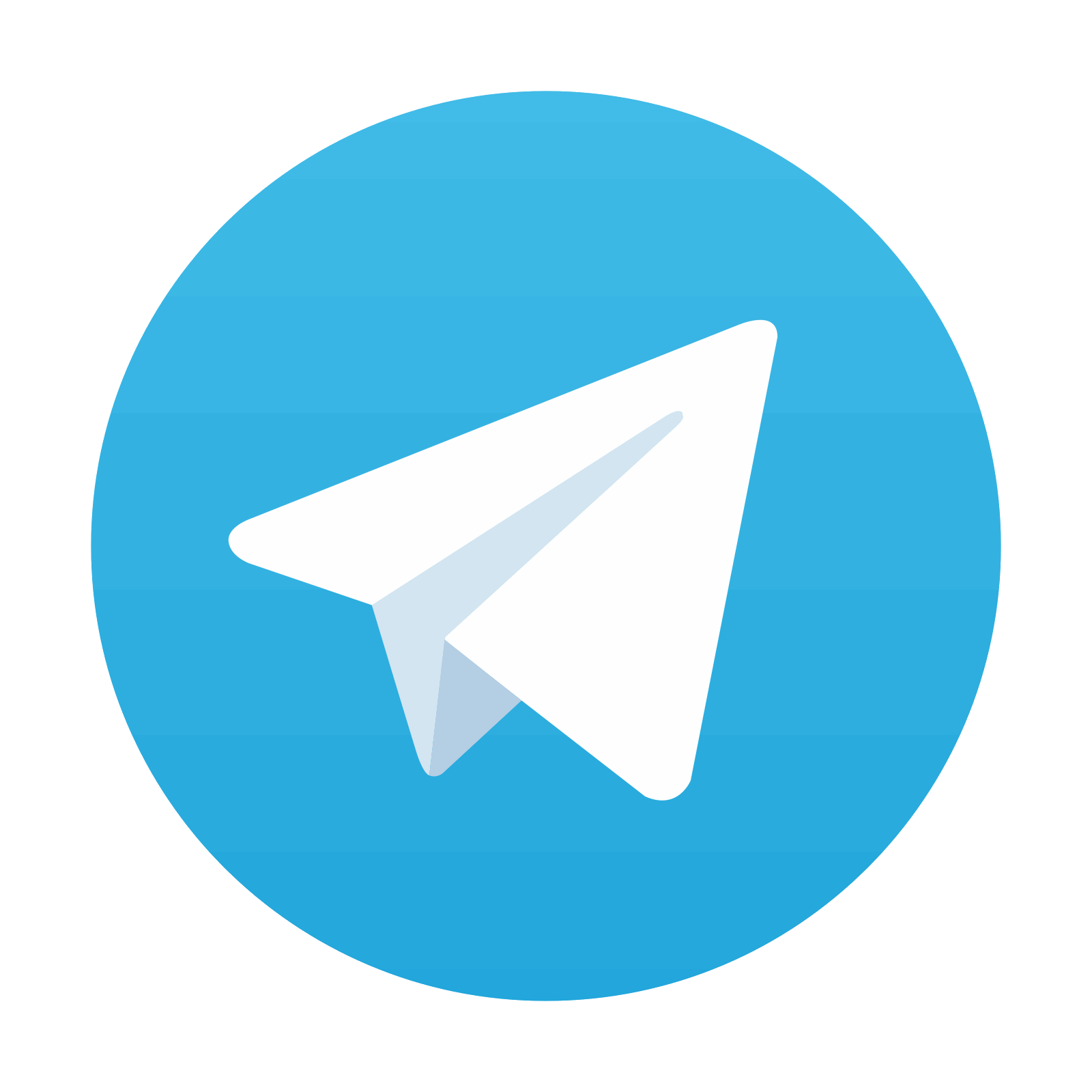
Stay updated, free articles. Join our Telegram channel

Full access? Get Clinical Tree
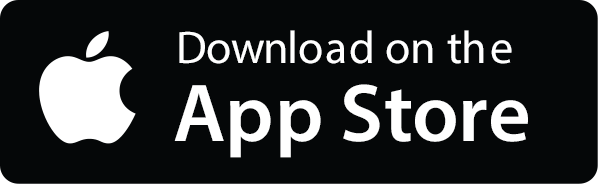
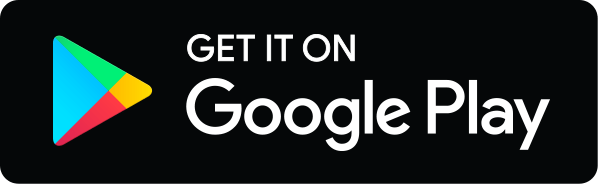