!DOCTYPE html PUBLIC “-//W3C//DTD XHTML 1.1//EN” “http://www.w3.org/TR/xhtml11/DTD/xhtml11.dtd”>
CHAPTER 30 EPILEPSY IN THE SETTING OF NEUROCUTANEOUS SYNDROMES
Neurocutaneous syndromes are genetically and clinically heterogeneous congenital diseases with common characteristics of distinct cutaneous stigmata in association with neurologic disease, and involvement of various organs and systems. Cutaneous findings, noted by the patient, the family, or a family physician, are usually the tip-off for suspecting a neurocutaneous disease. In a few neurocutaneous syndromes, epilepsy is the most common presenting symptom. Identification and chronic management of these patients with complex epilepsy issues often fall into the hands of neurologists and epileptologists, who sometimes lead a team of various specialists in a multidisciplinary clinic model to provide disease-based clinical care. Recognition of neurocutaneous syndromes associated with epilepsy is therefore of critical importance for neurologists and epileptologists. Accurate early diagnosis is vital to counsel the patient and the family regarding chronicity of the condition, choose appropriate antiepileptic drugs for epilepsy, ensure timely selection of candidates for epilepsy surgery, guide testing and consulting for coexisting morbidities, and, when appropriate, refer for genetic testing and counseling. In this chapter, we discuss diagnosis and treatment of such conditions. While our focus is on the diagnosis and treatment of epilepsy in these conditions, we touch upon important aspects of comorbid neuropsychiatric conditions, and other organ system involvement that impact decision making for medical and surgical treatment of epilepsy. Clinical, radiographic, and pathologic findings are presented in the pictorial atlas (Chapter 5).
TUBEROUS SCLEROSIS COMPLEX
Tuberous sclerosis complex (TSC) is a multisystem genetic disease with an autosomal dominant pattern of inheritance. The prevalence is reported to be 1 in 6000 to 10,000 (1). Seizures are one of the most common presenting symptoms in TSC, and most patients with TSC develop lifelong refractory epilepsy. The classic Vogt triad of mental retardation, seizures, and adenoma sebaceum that was used to diagnose TSC in the pre–magnetic resonance imaging (MRI) and gene testing era is found in only 29% of TSC patients (2,3). Diagnostic criteria for clinical diagnosis of TSC have been developed and are clinically useful (4). However, TSC remains a disease with extremely variable expression, and approximately 7% of the patients with TSC1 or TSC2 mutation may not meet diagnostic criteria for TSC, and 15% of patients who meet the criteria for TSC may not have a detectable mutation or deletion in TSC1 or TSC2 (5). Approximately 60% of the patients are the only family member affected, suggesting a new spontaneous mutation (6). Mutations in two genes have been identified in patients with TSC. TSC1 is located at chromosome 9q34 and encodes a protein called hamartin. TSC2 is located at chromosome 16p13.3 and encodes a protein called tuberin. Although located on different chromosomes, the two genes code two proteins that work in the same biochemical pathway, the mTOR (mammalian target of rapamycin) pathway that is critical to cell cycle regulation. Although most patients have mutations localizable to either TSC1 or TSC2 by currently available testing, 15% of patients may not have a detectable known mutation (7). Such patients should be screened for TSC 1 and 2 gene deletions. Sporadic mutations are more common in the TSC2 gene. TSC1 seems to have less severe phenotypic expression. Specifically TSC1 patients appear to have fewer seizures, fewer intracranial lesions, and less severe mental retardation (7). Both parents of a child who has TSC should be examined for evidence of the disease. When the index case has an identified pathogenic mutation, family members at risk could be screened by looking for that specific mutation. In a case with no identifiable mutations, the family screening could begin by testing of the biologic parents including a complete physical examination including a Wood lamp inspection of the skin, dilated eye examination, MRI of the head, and renal ultrasonography. If the biologic parents have no evidence of TSC, the recurrence risk for their next child is low due to a remote possibility of gonadal mosaicism; if either parent has the disease, the risk for another affected child is 50%.
Pathogenic mutations in TSC 1 or TSC 2 genes activate the mTOR cascade, resulting in abnormal growth and proliferation in various organs and systems. An understanding of this mechanism provided a unique opportunity to test already available mTOR inhibitor drugs such as rapamycin as a potential therapeutic agent targeting TSC-related tumoral diseases in the brain, kidneys, and lungs (8–10). Later, clinical trials led to the FDA approval of mTOR inhibitor everolimus as a treatment option in selected TSC patients with subependymal giant cell astrocytomas (SEGA) and renal angiomyolipomas (9,11–13).
Epilepsy and Neurologic Manifestations in TSC
Seizures are one of the most common presentations in TSC and occur in up to 80% patients with TSC. Most TSC patients with seizures tend to present early in life, and 70% of TSC patients develop epilepsy by the age of 1 year (3,14). A typical patient is an infant who presents with infantile spasms with no prenatal and perinatal adverse events (15–17). Lifelong neurologic morbidity of TSC is usually defined early in life by intractable epilepsy and global cognitive delay that typically go hand in hand. Early onset of epilepsy is one of the most important risk factors for continuing seizures and cognitive disability later in life (18). Most infants with epileptic spasms, despite going into remission with antiepileptic treatment (vigabatrin, ACTH, or other medications), develop complex partial, focal motor, tonic, or generalized tonic–clonic seizures later in life. It is generally accepted that most seizures in TSC are partial-onset seizures from one or more cortical tubers. Infrequently, a TSC patient may present with seizures later during teenage years or adulthood with exclusively partial seizures (3,18,19).
Electroencephalography (EEG) has no specificity to the diagnosis of TSC. A variety of abnormal findings could be seen during the interictal period including a picture of hypsarrhythmia during infancy and Lennox–Gastaut syndrome in older children and adults. Westmoreland found that 88% of TSC patients had abnormal recordings; 75% had epileptiform discharges, and focal slowing occurred in 13%. The epileptiform discharges were multifocal in 25% and focal in 23%; hypsarrhythmia occurred in 19% and generalized spike–wave discharges in 8% (19). Approximately 70% of focal spikes were in the temporal lobe. A variety of seizure onsets (ictal EEG) could be observed ranging from exclusively well-localized focal seizures with partial semiology to generalized or nonlocalized ictal EEG onset with generalized motor or bland seizures. It remains unclear if the threshold of epileptogenicity from the tuber(s) changes throughout the patient’s lifetime. It is also not known if and how multiple epileptogenic tubers interact with each other (or kindle each other), leading to an unpredictable course of epilepsy. Cusmai et al. (20) reported that during follow-up EEG, although some epileptic foci disappeared (usually occipital foci), others became evident, especially in the frontal regions (consistent with posterior–anterior migration of epileptic foci in childhood). The study also found that, although there was no correlation between the number of EEG foci and the number of cortical tubers, in 25 of 26 patients in this series, there was an EEG and MRI topographic correlation between at least one large tuber (larger than 10 mm in the axial plane and larger than 30 mm in the coronal plane) and an EEG focus. Secondary bilateral synchrony appeared in 35% of children with tuberous sclerosis after the age of 2 years, especially during drowsiness and sleep (20,21).
Fifty to sixty-five percent of TSC patients have mild to moderate mental retardation. IQ appears to be bimodally distributed in patients with tuberous sclerosis. On the good side of the spectrum are the few patients who have no or infrequent seizures beginning after early childhood with no or mild learning disabilities and an IQ that is lower than that of siblings without TSC. On the guarded side of the spectrum are TSC patients who present with infantile spasms or catastrophic epilepsy with onset before 12 months of age and who end up with moderate to severe mental retardation, limited or no speech, and inability to ambulate (22). Autism is commonly described in TSC patients and is reported in 25% to 50% of patients. Between 26% and 58% of children with tuberous sclerosis and infantile spasms have autism, compared with 13% of patients with infantile spasms who do not have tuberous sclerosis (22–24). The association between autism and tuberous sclerosis therefore appears to be more than coincidental. Both the number of tubers and their topography seem to play an important role in the cognitive outcome. The persistence of epileptic foci in anterior and posterior areas is thought to be important in the development of autistic traits, such as severe disability in verbal and nonverbal communication, stereotypies, and complete indifference to social interaction. Patients with multiple cortical lesions are likely to have developmental delay and intractable seizures. However, it is not possible to predict neurocognitive function or burden of epilepsy in an individual TSC patient by brain MRI alone. Patients with TSC also have other neuropsychiatric morbidity in the form of high frequency of hyperactive and aggressive behavior, and rarely self-mutilation (23). SEGA may grow and obstruct the CSF flow presenting with symptoms and signs of hydrocephalus requiring tumor excision with or without placement of a ventriculoperitoneal shunt.
Nonneurologic Lesions in TSC
TSC patients have a multitude of cutaneous lesions such as hypomelanic macules (ash leaf spots), adenoma sebaceum (facial angiofibromas), forehead plaques, shagreen patches, and ungual or subungual fibromas. In Gomez’s series of 300 tuberous sclerosis patients, skin lesions were seen in 96%, followed by seizures in 84%, retinal hamartomas in 47%, and mental impairment in 45% (3). Patients with TSC may have many visceral lesions including cardiac rhabdomyomas, retinal astrocytomas (hamartoma) in the eyes, renal cysts and angiomyolipomas, hepatic cysts, and pulmonary leioangiomyomatosis.
Brain Involvement and Neuroimaging in TSC
TSC is associated with a variety of brain lesions, and these are cortical tubers, white matter lesions, subependymal nodules (SEN), and SEGA (25,26). Histologically, each of the four types of intracranial lesions is composed of clusters of giant cells with varying degrees of neuronal and astrocytic differentiation, and the presence of cells that are transitional forms between these two types. On an unenhanced brain CT, cortical and subcortical white matter lesions in TSC appear hypodense and may be mistaken for a remote insult; however, the presence of multiple lesions and associated calcified SEN usually clarify the diagnosis. Many unsuspecting patients are identified to have TSC for the first time by a brain CT done as a part of workup for unrelated questions (e.g., in the emergency room after a head trauma).
While brain CT is helpful in noticing calcified lesions in TSC, brain MRI is the neuroimaging procedure of choice to elicit the extent of all lesions. Age is an important factor in the interpretation of brain MRI in TSC. Cortical tubers are hyperintense on T2 and FLAIR sequences, and hypointense on T1 sequences in patients with mature myelination. In newborns and infants with immature myelination, the tubers are hyperintense to unmyelinated white matter on T1 sequences and appear hypointense on T2-weighted images. This effect of immature myelination on the brain MRI findings in infants with TSC is felt to be secondary to the increased water content in unmyelinated regions of the brain. There is evidence to suggest that cortical tuber count and location are associated with increased risk of infantile spasms (27). There also appears to be a correlation between increased number of tubers, development of early seizures, and developmental delay.
There are three types of white matter lesions in TSC (25,26). These are, in the order of common occurrence, thin linear bands extending radially from the ventricular surface to cortical tubers, wedge-shaped bands with apices near ventricles, and amorphous lesions in the deep white matter. The white matters lesions tend to be predominant in frontal lobes.SEN are often near the caudate head or caudothalamic groove. They are variable in appearance and signal intensity. SEN do not usually obstruct CSF flow, but may uncommonly do so by mechanical pressure against the foramen of Monro. SEN may rarely enhance on gadolinium administration in a nodular or ring-like fashion, and enhancement is better appreciated at higher signal strengths. When small, SEN are better seen on CT than MR because of the presence of calcification. SEGA are slow-growing tumors. They are typically located near the foramen of Monro and are believed to originate from SEN. However, rarely SEGA may also appear in other locations in the brain. Typically, there is no edema in brain parenchyma adjacent to the SEGA. Most SEGA are benign, although there are rare cases of malignant degeneration. The symptoms of SEGA are mainly due to their location. TSC patients with SEGA often present with acute or chronic increased intracranial pressure suggesting chronic or intermittent obstruction at the level of the foramen of Monro. Incidence of SEGA in various studies on TSC is reported to be 1.7% to 26%. A series in a moderate-sized group of patients reported 8.2% (28). SEGA are generally iso- to hypointense to brain parenchyma on T1 and hyperintense on T2-weighted images. SEGA are heterogeneous in appearance. Flow voids may be identified within these lesions. They often have internal susceptibility artifact reflecting hemorrhage or calcification. SEGA usually show contrast enhancement. SEGA should be suspected and periodic screening scans are indicated if SEN show increased size and contrast enhancement.
Medical Treatment of Epilepsy
The first-line treatment for seizures is antiepileptic medications. Many case series and randomized clinical trials show that vigabatrin is particularly effective in the treatment for infantile spasms and partial seizures during infancy in TSC (29–31). Vigabatrin may cause irreversible peripheral field visual defects after long-term use, and most experts agree not to use vigabatrin beyond 6 to 12 months of treatment. Corticotropin (ACTH) provides an alternative treatment; the response of children with tuberous sclerosis and infantile spasms to corticotropin is similar to the response of children with cryptogenic infantile spasms; however, those with tuberous sclerosis have a higher relapse rate. Vigabatrin may be superior compared to ACTH considering the similar or marginally better rate and rapidity of seizure remission, ease of oral administration, lack of serious side effects in the short term, and significantly lower cost of treatment (29–31). Many experts use Vigabatrin as the first-line drug in infantile seizures in the setting of TSC. Other antiepileptic treatments could be used in TSC following the general guidelines of partial versus generalized seizures. However, one should keep in mind that most seizures in patients with TSC are partial in origin despite an overwhelming electroclinical picture of generalized epileptic encephalopathy and, therefore, not contraindicating antiepileptic drugs that are effective only for partial epilepsy. Coexisting renal morbidity in TSC warrants cautious use of topiramate and zonisamide, and ketogenic diet. Extra caution is required for monitoring preexisting neuropsychiatric and behavior issues that may paradoxically show worsening with antiepileptic drug(s). Recently, mTOR inhibitors have been proposed as an option for treatment of epilepsy in TSC. However, it remains controversial, and at the time of writing this chapter mTOR inhibitors are not approved by the FDA (USA) for treatment of epilepsy in patients with TSC, but treatment trials are ongoing, suggesting encouraging preliminary results (32).
Surgical Treatment and Outcome of Epilepsy in TSC
Like in any other refractory partial epilepsy, when medical treatment fails, evaluation for the possibility of epilepsy surgery should be considered early in every patient with TSC (33). Frequency of medical intractability of seizures is higher in TSC and reported to be approximately 50% in patients with tuberous sclerosis and partial epilepsy (18). Seizure freedom or effective control of debilitating seizures after epilepsy surgery would likely improve quality of life in patients and families with TSC. However, two key rules for planning surgical strategy, localization of the epileptogenic zone (EZ) and minimizing risk of a permanent new postoperative deficit(s), pose additional challenges unique to TSC. These challenges emanate from multiple factors, often age related, and in complex interaction with each other. First, the presence of multiple bilateral tubers, sometimes partially or nearly confluent, on the brain MRI portrays a possibility of multiple epileptogenic lesions. The tubers often intermingle with white matter abnormalities and defy accurate identification of margins even on a high-resolution brain MRI making anatomical delineation for surgery inaccurate. Second, the patient is frequently a child or a cognitively disabled young adult with stereotypic nonfocal seizure semiology (epileptic spasms, bland seizures with behavior arrest, tonic or atonic seizures with falls) and a scalp video-EEG that often shows overwhelming generalized or multiregional interictal abnormalities and nonlocalizable ictal onset. Considering recent reports of a single epileptogenic lesion on the brain MRI leading to generalized and multiregional interictal and ictal abnormalities on scalp EEG, a scenario of multiple epileptogenic lesions with such an EEG appears to be an insurmountable condition for epilepsy surgery (Chapter 88). Third, young age along with cognitive delay and behavior issues renders cooperation for noninvasive mapping of eloquent functions, whenever necessary, challenging or even impractical. And lastly, lack of long-term longitudinal postoperative outcome studies makes family counseling before surgery imprecise as there is always a potential concern for emergence of epileptogenicity from the nonresected tubers. It is therefore no wonder that in the quest for a successful surgical strategy in TSC, multiple presurgical investigative tools in various combinations and recipes have been reported in the published case series from various centers (33).
Multimodal presurgical tools such as 18F fluorodeoxyglucose–positron emission tomography (PET), alpha-[(11)C] methyl-l-tryptophan (AMT)-PET, ictal single photon emission computed tomography, and magnetoencephalogram (MEG) coregistered to the brain MRI have been used in varying combinations with claims of success; however, there is no perfect formula for identification of the epileptogenic tuber, and neither have any tools, alone or in combination, been shown to improve the rate of seizure freedom after surgery (33–40). A logical step-by-step by investigation tailored to each patient remains the cornerstone of presurgical evaluation in TSC. Recently, in patients with unidentifiable EZ (epileptogenic tuber(s)) on multimodal noninvasive investigations, bilateral subdural and depth electrode implantation encompassing wide regions of brain bilaterally followed by a focused search for the epileptogenic tuber(s) with staged resection(s) has been reported from one center (41). Palliative procedures, such as corpus callosotomy or partial or complete resection of the “most” epileptogenic tuber (cause of most frequent or most severe seizures), could also help in some patients with TSC where surgery is done to minimize injuries, abolish the most disabling seizures with falls and loss of consciousness, and prevent episodes of life-threatening status epilepticus.
Published series on epilepsy surgery in TSC have reported high rates of success in alleviating or significantly improving seizures in most patients who undergo surgery. In a systematic review of literature published between 1960 and 2006, Jansen et al. (42) reviewed a sample of 177 TSC patients who underwent epilepsy surgery and were subjects in 25 published articles in peer-reviewed journals. Not surprisingly, authors found these observational case series incomparable due to extreme variability in the collection and reporting of data (42). However, in a composite analysis, 75% TSC patients were either seizure free (57%; 0.1 to 47 [mean 3.7] years follow-up) or >90% improved in seizure frequency (18%; 0.5 to 20 [mean 4.2] years follow-up). Also of interest was the finding that a large number of TSC patients who underwent surgery had generalized and multiregional interictal scalp EEG abnormalities (48%) and nonlocalizable ictal onset (46%) findings; however, the presence of focal versus nonfocal scalp EEG abnormalities had no statistically significant relationship to seizure remission after surgery (42). Obviously, these patients were selected for epilepsy surgery based on other (not studied in the paper) criteria(s) such as semiology, functional deficits, dominant tuber(s) on the brain MRI, nuclear imaging studies, or MEG, again highlighting the point that generalized and multiregional EEG abnormalities on the scalp video-EEG does not preclude surgical candidacy in a child with a solitary or multiple lesions (such as in TSC). In a recent series of 33 TSC patients who underwent epilepsy surgery, complete removal of epileptogenic tissue detected by MRI and intracranial EEG, regional scalp interictal EEG patterns, and agreement of interictal and ictal EEG localization were the most powerful predictors of seizure-free outcome (43). Other significant predictors included occurrence of regional scalp ictal EEG patterns, fewer brain regions affected by tubers, presence of preoperative hemiparesis, and one-stage surgery. Remaining factors such as age at seizure onset, incidence of infantile spasms or other seizure types, duration of epilepsy, seizure frequency, mental retardation, as well as types and extent of resections did not influence outcome (43).
Overall, despite additional challenges of epilepsy surgery in TSC, the findings from the published TSC series are quite similar to the proven principles and practice of epilepsy surgery in children with extratemporal epilepsy substrates (Chapter 89). The investigation to find the culprit tuber and perituberal regions (and define the EZ) is, therefore, best individualized to each patient considering all aspects of his or her clinical condition and goals of epilepsy surgery. No formula fits all TSC patients. Every TSC patient with refractory epilepsy should undergo presurgical evaluation using a customized approach. In a few refractory patients who were not candidates for resective surgery or did not benefit from resection, vagus nerve stimulator implantation may offer some palliative benefit (44–46).
STURGE–WEBER SYNDROME
Sturge–Weber syndrome (SWS) or Sturge–Weber–Dimitri syndrome is also known as encephalotrigeminal angiomatosis or encephalofacial angiomatosis. SWS was first described in 1879 by Sturge, who thought that the neurologic features of the syndrome resulted from a nevoid condition of the brain similar to that affecting the face. Volland in 1912 and Weber in 1922 described the intracranial calcifications, and Dimitri was the first to report a case with calcifications seen on skull roentgenogram. SWS is a sporadic disease presumed to be caused by somatic mutation. Prevalence of SWS is estimated in one study to be 1 in 50,000. In a recent tissue-based study, SWS (brain tissue) and port-wine stains (PWSs) (cutaneous tissue) were shown to be caused by somatic mosaic mutations in the GNAQ gene leading to disruption of normal vascular development (47). The study group identified a nonsynonymous single-nucleotide variant (c.548G–>A, p.Arg183Gln) in GNAQ in samples of affected tissue from 88% of the participants (23 of 26) with the SWS and from 92% of the participants (12 of 13) with apparently nonsyndromic PWSs, but not in any of the samples of affected tissue from 4 participants with an unrelated cerebrovascular malformation or in any of the samples from the 6 controls (47).
Characteristic clinical features of SWS are unilateral facial PWS, ipsilateral pial angiomatosis, epilepsy, stroke-like episodes and glaucoma. Neurologic manifestations include seizures, varying degrees of mental retardation, migraine-like headaches, intermittent or progressive stroke-like episodes with focal deficits such as hemiparesis, hemiatrophy, aphasia, and hemianopsia (26,48,49).
Chronic cortical ischemia from angiomatous malformation leading to calcification and laminar cortical necrosis have been proposed as likely mechanisms of brain injury. During the sixth week of intrauterine life, the primitive embryonal vascular plexus develops around the cephalic portion of the neural tube and under the ectoderm in the region destined to be the facial skin. In SWS, it is hypothesized that the vascular plexus fails to regress, as it should in the embryo in the ninth week, resulting in angiomatosis of related tissues (50). The intracranial lesion is thought to be related to proliferation of leptomeningeal vessels in the subarachnoid space, which causes shunting of blood away from the brain tissue resulting in decreased blood flow, decreased venous return (venous stasis), and consequent focal hypoxia leading to cellular death. This is seen radiographically as gliosis, volume loss, and calcification.
Epilepsy and Neurologic Manifestations in SWS
Seizures are the most common neurologic presentation, and occur in 75% to 80% of children with SWS. Only 10% to 20% of children with unilateral port-wine nevus of the forehead have a leptomeningeal angioma. Typically, SWS involves occipital and posterior parietal lobes ipsilateral to the port wine nevus, but it can affect other cranial regions and both cerebral hemispheres. Bilateral brain lesions occur in 15% of children. Bilateral hemispheric involvement increases the risk of seizure. The age range of seizure onset varies between birth and 23 years with a median age of 6 months (51
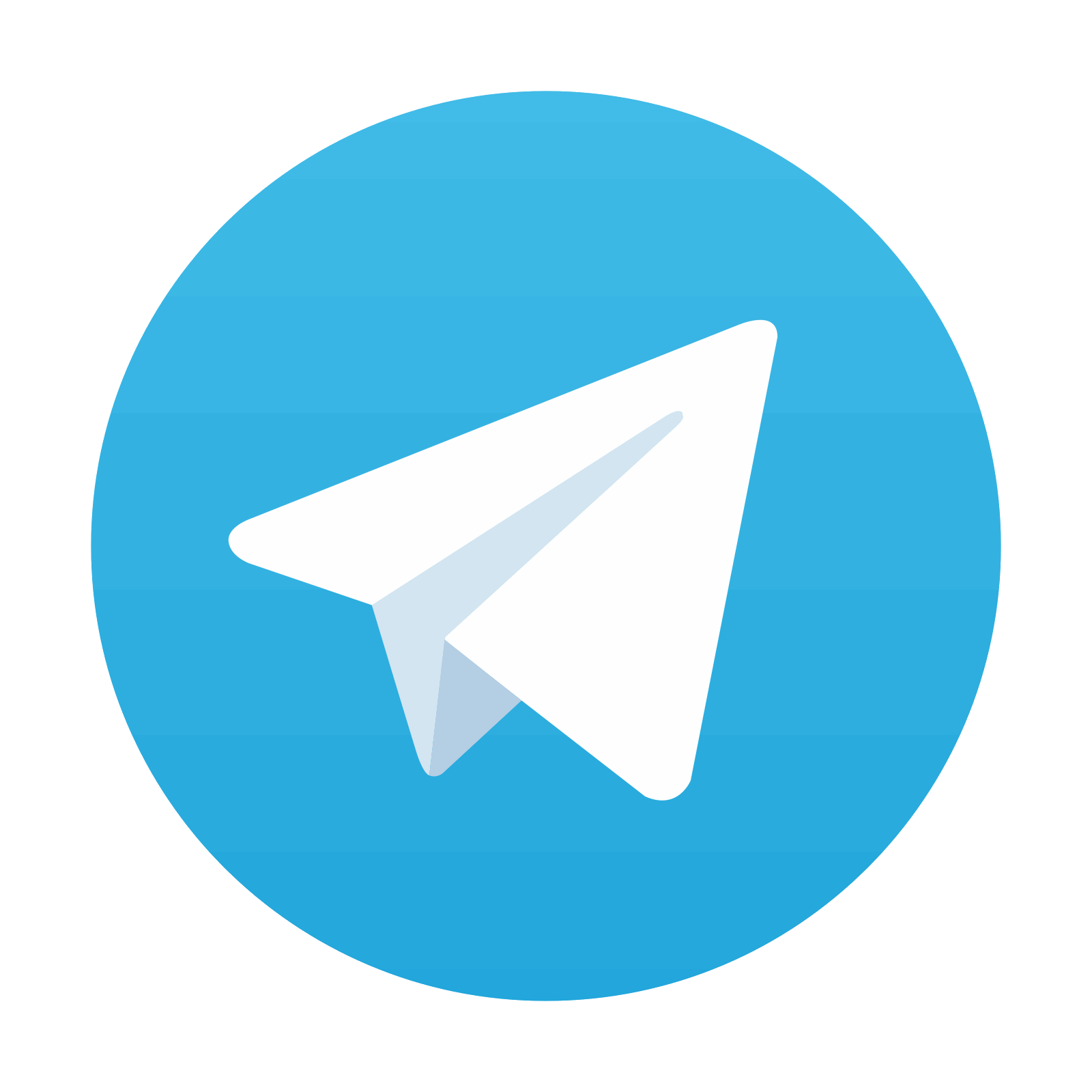
Stay updated, free articles. Join our Telegram channel

Full access? Get Clinical Tree
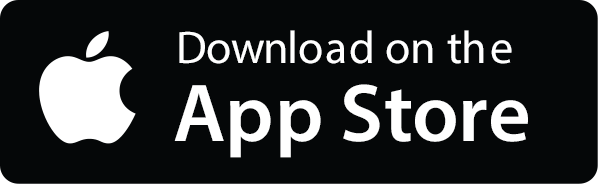
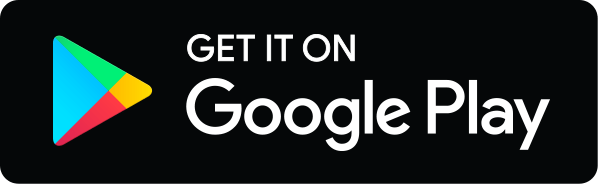