12
Evoked Potentials in Pediatric Brainstem Lesions
The human brainstem consists of the midbrain, pons, and medulla, contains 9 of the 12 cranial nerves, and is crossed by the ascending, descending, and cerebellar pathways and their nuclei as well as the reticular formation. Lesions in the brainstem may affect auditory and somatosensory pathways. Evoked potentials (EPs) or evoked responses (ERs) are electrical potentials recorded from the nervous system after the presentation of sensory stimuli. EPs are small electrical events arising from neural tissue and occurring in response to abrupt sensory stimulation. Auditory and somatosensory (and visual) stimuli are the methods of stimulation used commonly for clinical evoked potential studies. They consist of a series of waves that reflect the sequential activation of neural structures along the sensory pathways.
In current clinical application, there are two main types of sensory EPs used to assess the functional status of the brainstem. These are short-latency or brainstem auditory evoked responses (BAERs) and the short-latency somatosensory evoked potentials (SSEPs). BAERs and SSEPs provide sensitive measures of the central conduction functions of the auditory and somatosensory input systems at different levels of the central nervous system (CNS). BAER is the major objective test used in the detection of intrinsic or extrinsic lesions of the brainstem and play a major role in the testing of hearing. SSEPs have the capacity to evaluate both the peripheral nervous system and CNS from the distal peripheral nerves to the sensory cortex. The other important sensory potentials are visual evoked potentials (VEPs) that are often used in ophthalmologic assessment. In clinical application, evaluation with EPs are often less costly than other evaluation techniques such as MRI.
BAERs are also referred to as brainstem auditory evoked potentials (BAEPs) or auditory brainstem responses (ABRs). The responses have been widely used in pediatric, particularly neonatal, neurology to study and assess the hearing or auditory function and neuropathology that may involve the brainstem auditory pathway (1–3). Following brief acoustic stimuli (usually broadband clicks), the brainstem responses are detected by averaging the electroencephalogram (EEG) immediately after each of several thousand stimuli. The submicrovolt deflections in a recorded BAER occur in the first 10 minutes after the stimuli. The first wave component (wave I) in a BAER waveform recorded in normal adults occurs within 2 minutes after acoustic stimuli, with a cochlear microphonic and subsequent compound action potential from the auditory nerve. The following BAER components (waves II to V) occur within the first 7 to 9 minutes in adults and are of brainstem origin. Although the exact generators of the BAERs are not precisely localized, in general, the waveforms in clinical use are attributed to distinct brainstem regions for applicable purposes of interpretation. Wave III is thought to be of lower pontine generation, and wave V is thought in general to have lower midbrain generation (Figure 12.1). Wave I is a near-field response (meaning that the source of generation is close to the site of recording) and is recorded at the ear or mastoid electrode and generated by the peripheral nervous system. All the following waves are far-field responses (generated far from site of recording), generated in the brainstem but recorded at the scalp. Because of the immaturity of the brainstem auditory pathway, the latencies of BAER wave components in infants are longer than in adults, while the amplitudes are generally smaller than in adults. After birth, over time, BAER wave latencies and interpeak intervals are shortened or decreased while wave amplitudes are increased. This maturational process continues until early childhood.
FIGURE 12.1 Neural origin of BAER components.
Abbreviations: VIII N, auditory nerve; AC, auditory cortex; BAER, brainstem auditory evoked response; DCN, dorsal cochlear nucleus; E, extracranial; I, intracranial; IC, inferior colliculus; LL, lateral lemniscus; MGN, medial geniculate nucleus; NTB, trapezoid body; SOC, superior olivary complex; VCN, ventral cochlear nucleus.
One important advantage of BAERs is that they are unaffected by sleep state or sedation. This has led to a wide interest in using BAERs in pediatric audiology and neurology to assess the functional status or integrity of both peripheral and central, specifically brainstem, auditory pathways (1–3). In addition to assessing auditory function, the BAERs have been extended to quantitatively evaluate neural integrity and maturation of the immature brainstem and detect brainstem lesions in many pathological conditions that affect the brainstem auditory pathway. More recently, the maximum length sequence (MLS) has been introduced in pediatric neurology to record and analyze BAERs. This relatively new technique, which can exert a more stressful physiological/temporal challenge to brainstem auditory neurons, has been documented to improve the detection of brainstem lesions in pediatric, particularly neonatal, neuropathology (1,2,4).
SSEPs are also used to evaluate brainstem function. They can be elicited by mechanical stimulation, but in clinical application, SSEPs are elicited by electrical stimulation of peripheral nerves, which produces larger and more robust responses than mechanical stimulation. The stimulation sites typically used for clinical diagnostic SSEP studies are the median nerve at the wrist, the common peroneal nerve at the knee, and/or the posterior tibial nerve at the ankle. Recording of the SSEPs to stimulation of the ulnar nerve at the wrist is useful for intraoperative monitoring when the midcervical spinal cord or parts of the brachial plexus are at risk. Recording electrodes are placed over the scalp, spine, and peripheral nerves proximal to the stimulation site. The series of waves generated in a SSEP waveform reflect the sequential activation of neural structures along the somatosensory pathways. SSEPs are used for clinical diagnosis in patients with various neurologic diseases that affect the somatosensory pathways and for intraoperative monitoring during surgeries that place parts of the somatosensory pathways at risk. An abnormal SSEP can result from dysfunction at the level of the peripheral nerve, plexus, spinal root, spinal cord, brainstem, thalamocortical projections, or primary somatosensory cortex. The SSEP components generated in the brainstem and in the cerebral cortex are mediated entirely by the dorsal columns (posterior columns) of the spinal cord, the fasciculus cuneatus for upper limb SSEPs and the fasciculus gracilis for lower limb SSEPs. Lesioning of the dorsal columns of the spinal cord rostral to the root levels where the afferent somatosensory activity enters the spinal cord abolishes the SSEPs generated in the brain. However, the SSEPs can persist, following lesions of the anterolateral spinal cord. Diseases of the dorsal columns in which joint position sense and proprioception are impaired are invariably associated with an abnormal SSEP.
The latencies of SSEP components are, in general, shorter in infants and children than in adults and change progressively with growth and maturation. The latency changes predominantly reflect linear growth with elongation of the peripheral nerves and central somatosensory pathways. These effects are counterbalanced partially by myelination and increase in fiber diameters, which produce faster conduction velocities, and partially by maturation of synaptic transmission. The latter effects operate until age 6 to 8 years, at which time central conduction times (CCTs) have reached adult levels, and further latency changes are due to changes in stature.
The advance of sophisticated neuroradiologic imaging has had a great impact on the clinical use of EPs, with fewer diagnostic EP studies performed than in the pre-MRI and early MRI era. Nevertheless, EPs remain valuable as electrophysiological diagnostic measures in many clinical situations. Among the EPs, BAERs are most affected by brainstem lesions. Over the last three decades, BAERs have been the focus of noninvasive electrophysiological examinations of the functional integrity of the brainstem in pediatric neurology. Compared with SSEPs, VEPs, and the long- and middle-latency auditory evoked potentials (LAEPs and MAEPs), BAERs are simpler to record and are unaffected by sleep state or sedation, which makes the responses particularly suitable for use in very young infants and sick children. Numerous clinical studies have documented that BAERs are an important tool and adjunct to detect neuropathology that involves the brainstem auditory pathway in a wide range of pediatric and neonatal diseases. This chapter is mainly focused on the clinical application of BAERs in the diagnosis and management of brainstem lesions in some common pediatric, particularly neonatal, problems.
TECHNICAL FACTORS IN THE ASSESSMENT OF BAERS
Click Intensity and Repetition Rate
For the purpose of neurologic assessment, it is crucial to clearly and reliably identify wave I in the recorded BAER waveforms, which will allow accurate calculation of I-III interpeak interval and I-V interpeak interval—the so-called brainstem conduction time (BCT) and the most important and commonly used BAER variable to reflect brainstem function. Wave I can be enhanced by changing the stimulus parameters (eg, increasing stimulus intensity, decreasing stimulus repetition rate), using an ear canal or tympanic electrode or transtympanic electrocochleography (ECochG). In patients with peripheral auditory deficits, the simple way to enhance wave I is to increase stimulus intensity. For infants with a BAER threshold within the normal range (≤20 dB nHL), the intensity of click stimuli used to elicit conventional BAERs is usually between 70 and 80 dB nHL. For infants with a BAER threshold greater than 20 dB nHL, the intensity of clicks should be increased as appropriate in order to collect a well-formed BAER waveform.
For MLS BAER testing, the click repetition rates used are much higher than those used in conventional BAER, ie, the BAERs obtained using conventional averaging methods. This results in an increased perception of loudness of the stimulus trains due to temporal integration. Therefore, the click intensity should be relatively lower than that used in conventional BAER. A click intensity level at 45 to 55 dB above the BAER threshold of each subject is usually the best method to obtain a satisfactory MLS BAER waveform, with well-formed waves I, III, and V. Thus, 60 or 65 dB nHL is usually best for infants with a normal BAER threshold (≤20 dB nHL) (1,4). For infants with a BAER threshold greater than 20 dB nHL, the intensity of clicks should be increased to at least greater than 40 dB above the threshold of each individual infant. This will ensure BAER waves I, III, and V to be clearly identified in the recorded waveforms. For infants with a threshold greater than 45 dB nHL, however, it is often difficult to obtain well-formed and reliable waves I, III, and V at any high click intensities. For group comparison, it is important to ensure that there is no significant difference between groups in the click intensity level above the threshold of individual subjects in order to compensate for individual differences in hearing sensation and for the influence of sensation level on wave latencies, amplitudes, and interpeak intervals.
In some clinical situations, infants with neuropathology that affects the brainstem auditory pathway may not show any apparent BAER abnormalities at conventionally used repetition rates of clicks, eg, 11/sec or 21/sec. Increasing the stimulus rate could be a useful “stimulus challenge test” to improve the detection of some neuropathology that may not be shown by the BAERs recorded with relatively slow stimulus rates (1,4). However, a significant increase in click rate, eg, 91/sec, in conventional BAERs can degrade the recorded BAER waveform morphology, and, in turn, can cause difficulty in accurate and reliable identification and measurement of BAER wave components.
MLS Technique
The MLS is a relatively new technique to record and analyze BAERs (1,2,4–10). It can exert a more stressful physiological/temporal challenge to brainstem auditory neurons, thus potentially improving the detection of neuropathology that may not be detected by conventional BAERs (1,4). The MLS technique allows the presentation of stimuli at much higher rates (up to 1,000/sec or even higher) than is possible with conventional averaging methods. Since the higher rates provide a much stronger temporal/physiological challenge to auditory neurons and permit a more exhaustive sampling of physiological recovery or “fatigue” than is possible with conventional stimulation, this technique can potentially enhance the sensitivity of BAERs in the diagnosis of neuropathological conditions. In the past decade, MLS has been documented to be a valuable technique to enhance the diagnostic value of BAERs, particularly for some early or subtle neuropathology, which may not be detected by conventional examination and investigations (1,4,6–16).
The repetition rates of clicks for eliciting MLS BAERs are usually 91/sec, 227/sec, 455/sec, and 910/sec (1,4). Jiang proposed that in terms of detecting neuropathology that involves the brainstem auditory pathway, MLS BAER abnormalities are better detected with high-rate stimulation, typically 455/sec and 910/sec. The highest rate of 910/sec is very “stressful.” However, this rate can cause some alteration in the elicited MLS BAER waveform, which may result in difficulty in accurate and reliable identification and measurement of waves I and V. The rate 455/sec usually elicits well-formed MLS BAER waveforms, and appears to be “stressful” enough for the brainstem auditory neuron as to significantly enhance the detection of neuropathology. Therefore, 455/sec is the preferred rate of the four click repetition rates for clinical application (1). Due to temporal integration, the perception of loudness of the stimulus trains increases as the repetition rate is increased. Thus, a click intensity of 80 dB nHL or higher is intolerable for subjects with normal hearing. Even 70 dB nHL tends to be too loud for some subjects, particularly when the test is of a long duration (2,6). In addition, at such high click intensities, the recorded MLS BAER waveforms are often distorted or deteriorated.
Measurement of BAER Variables
The schematic measurement of various BAER components in children and adults is shown in Figure 12.2. Usually, the major point on a wave component that produces the greatest amplitude is selected as the peak of the wave. In some recordings, the top portion of a BAER wave is rounded or consists of a couple of small peaks, and there is no sharp peak of maximum amplitude that can be picked as the wave peak. A general solution is to extend lines from the two slopes of the wave component to a point where the two lines intersect. This point is then taken as the peak of the wave. There are several other methods to pick the peak, but all have certain limitations. The selection of methods is largely dependent on the particular waveform.
FIGURE 12.2 Measurement of (MLS) BAER wave components in children.
Abbreviations: amp, amplitude; BAER, brainstem auditory evoked response; IPI, interpeak interval; lat, latency; MLS, maximum length sequence.
The latency of a BAER wave is the time interval between the onset of the stimulus presentation and the appearance of a wave peak in the waveform. There are three major BAER wave latencies (wave I, III, and V latencies). Interpeak interval is the relative measure calculated as the time between the peaks of two different BAER waves. There are three interpeak intervals, including I-V, I-III, and III-V, and an interval ratio of III-V over I-III intervals (ie, III-V/I-III interval ratio) to reflect the relative changes in the two small intervals. The amplitude of a BAER wave is the measurement of the voltage difference between the peak and the preceding or following trough of a wave. The amplitude of wave I is measured from the positive peak of wave I to the negative trough immediately after the peak. Since the trough after wave III is considerably variable, it is not reliable to use the trough to measure the amplitude of wave III. Instead, the amplitude is measured between the peak of wave III and the immediate preceding trough of wave III. The amplitude of wave V is measured from the positive peak of wave V to the major negative trough immediately after the peak. With the amplitudes of BAER waves I, III, and V, relative amplitude ratios (ie, V/I and V/III amplitude ratios) are then calculated to reflect the relative changes in these amplitudes. In newborns and young infants, there is often considerable variation in the amplitude of wave I, which in turn significantly affects the reliability of the diagnostic value of the V/I amplitude ratio (13). To minimize this variation, the amplitude of wave I in young infants is measured from its peak to the lowest trough between waves I and III. The amplitude of wave III in young infants is measured from the peak of wave III to the lowest trough between waves I and III (1,13).
BAER Abnormalities in Brainstem Lesions
In brainstem lesions, the typical BAER abnormality is an abnormal increase in I-V interpeak interval, along with an increase in wave V latency. Intervals I-III and/or III-V may also be increased, depending on the location of the lesion in the brainstem and the nature of the pathology. For instance, wave V latency and I-V interval were found to be significantly increased in both infants with chronic lung disease (CLD) and infants after perinatal asphyxia (14). CLD infants showed a significant increase in the III-V interval but a generally normal I-III interval at all click rates, whereas infants after asphyxia showed a significant increase in both III-V and I-III intervals. Interval I-III was shorter and the III-V/I-III interval ratio was greater in CLD infants than in asphyxiated infants. Thus, CLD infants had a major increase in the more central BAER component (III-V interval), with no appreciable increase in the more peripheral component (I-III interval). In contrast, asphyxiated infants had a significant increase in both central and peripheral components (I-III and III-V intervals). These differences indicate that neonatal CLD affects the central regions of the brainstem more, whereas perinatal asphyxia affects both peripheral and central regions. This difference is likely related to the difference in the nature of pathology between CLD and asphyxia (14,15).
Compared with BAER wave latencies and interpeak intervals, the amplitudes of BAER wave components have a relatively large intersubject variability. Nevertheless, under well-controlled and consistent testing conditions, the amplitudes of BAER waves, particularly wave V, are useful variables to reflect the functional status of brainstem auditory neurons (17–22). The amplitudes can be reduced in some brainstem lesions, but not in others. For instance, the amplitude of BAER wave V was significantly reduced in perinatal asphyxia, but not in neonatal CLD, suggesting that there is major neuronal impairment in the auditory brainstem in asphyxia but not in CLD (13,19). This difference may be, at least partly, related to the difference in the nature of hypoxia associated with the two problems; the hypoxia in CLD is chronic and sublethal, which does not exert a major effect on brainstem neurons, but the hypoxia, which is associated with ischemia, in perinatal asphyxia is often acute and lethal and is detrimental to brainstem neurons.
HYPOXIC-ISCHEMIC ENCEPHALOPATHY
In infants with hypoxic-ischemic encephalopathy after perinatal asphyxia, lesions of the brainstem can be noted on MRI, with several distributive patterns of hypoxic-ischemic injury. There are often subtle but definite uniform symmetric brainstem lesions (23). Asphyxiated infants can manifest selective brainstem injury and exhibit palsy of the lower brainstem cranial nerves. Early histopathologic studies have shown that following perinatal asphyxia, or hypoxia-ischemia, there are discrete brainstem lesions involving auditory centers, including loss of neurons with gliosis or ischemic cell changes in the inferior colliculus and superior olivary complex (24). In fetal primates, asphyxic injury of the inferior colliculus was found to be one of the earliest effects of acute total asphyxia.
Since the late 1970s, BAERs have been used to examine functional impairment in the neonatal brainstem in infants after perinatal asphyxia. More recently, the responses have also been used to assess some therapeutic (eg, brain cooling) effect on the brainstem after hypoxia-ischemia (25). The BAER abnormalities in infants after hypoxia-ischemia include increased wave latencies and interpeak intervals, reduced wave amplitudes, and decreased V/I amplitude ratio, suggesting hypoxic-ischemic brainstem lesions (18,26). In conventional BAERs, a moderate increase in the click rate from 21/sec to 51/sec may not significantly improve the detection of BAER abnormalities, but a significant increase to 91/sec could (Figure 12.3) (26). In MLS BAER, the abnormalities are more pronounced at very high repetition rates (455/sec and 910/sec) than at lower rates (Figure 12.4) (6,7,13,27,28).
In term infants after hypoxia-ischemia, there are characteristically dynamic changes in the BAERs during the first month after birth, typically seen in the I-V interval (Figure 12.5). Jiang et al found that on the first day after birth, wave III and V latencies and I-V and III-V interpeak intervals are increased significantly, particularly at higher click rates (7,26). The abnormalities reach a peak on the third day after birth. Thereafter, the increased wave latencies and intervals recover progressively. These BAER variables approach normal values on day 15. By the end of the first month, the BAER abnormalities almost completely recover, although III–V and I–V intervals remain slightly increased (7,26). The dynamic changes, which are particularly obvious in MLS BAER, demonstrate a general trend of functional impairment of the neonatal brainstem after perinatal asphyxia, reflecting a specific time course of pathophysiological changes in the brainstem during the acute phase of hypoxic-ischemic brain damage (7). The impairment progresses shortly after birth, reaches a peak on the third day, and tends to recover progressively thereafter. The first week, particularly the first 3 days, is a critical period of hypoxic-ischemic brainstem damage. Two weeks after birth, the damage recovers significantly and largely returns to normal. By 1 month, the damage almost completely recovers in most cases.
FIGURE 12.3 BAER recordings at 21/sec, 51/sec, and 91/sec clicks in a normal term infant (A) and an asphyxiated term infant (B). Wave latencies and intervals are increased, and wave V amplitude reduced, mainly at 91/sec, in infant B.
Abbreviation: BAER, brainstem auditory evoked response.
FIGURE 12.4 MLS BAER recordings made from a normal term infant (A) and two term infants after severe asphyxia (B and C) on day 3 after birth. Compared with normal infant A, the amplitudes of waves I, III, and V in infant B are all reduced at all click rates, particularly of wave V at high rates. In infant C, BAER wave latencies, intervals, and wave amplitudes are basically normal at 91/sec clicks. As click rate is increased, the latencies and intervals are increased, and the amplitudes of wave I and, particularly, of wave V are significantly reduced.
Abbreviations: BAER, brainstem auditory evoked response; MLS, maximum length sequence.
This characteristic time course provides important information for studying and implementing any timing intervention or therapeutic measures to protect the neonatal brain and reduce further hypoxic-ischemic damage (7,26). The progression and deterioration of the abnormalities in BAERs during the first 3 days after birth indicate that early intervention during the critical first few days might prevent further hypoxic-ischemic brainstem damage or reduce the deterioration of damage. The time course could be used as a reference to monitor cerebral function, assess the responses of the brain to neuroprotective and/or therapeutic interventions, and help to judge the value of therapeutic interventions. The time course also provides a valuable time frame for recording BAERs in infants after perinatal hypoxia-ischemia. The first recording can be made on the first day after birth for the early detection of hypoxic-ischemic brain damage and for assessment of the severity of the damage. The second recording can be made at approximately day 3 to examine whether the damage has deteriorated or not. The third recording can be made on days 7 to 10 to assess any significant recovery. The fourth recording can be made at 1 month to examine whether the damage has largely resolved and the MLS BAER has returned to normal.
Figure 12.5 shows dynamic change in the I-V interval of MLS BAER during the first month after birth in a group of term infants after perinatal asphyxia (7). On day 1, the interval increased significantly at all repetition rates of clicks used (91–910/sec), especially the higher rates. On day 3, all these latencies and intervals increased further and differed more significantly from the normal controls. Thereafter, the latencies and intervals decreased progressively. On day 7, wave V latency and all intervals still differed significantly from the controls. These dynamic changes were more significant at higher rates of clicks than at lower rates. On days 10 and 15, all intervals decreased significantly. On day 30, all wave latencies decreased to the values in the normal controls on the same day. The intervals also approached normal values, although III-V and I-V intervals still increased slightly. These dynamic changes demonstrate a general time course of brainstem pathophysiology after perinatal asphyxia; the hypoxic-ischemic brain damage persists during the first week, with a peak on day 3, and recovers progressively thereafter. By 1 month, the damage has largely resolved. The first week, particularly the first 3 days, is a critical period of hypoxic-ischemic brain damage, and early intervention may prevent or reduce deterioration of the damage.
FIGURE 12.5 Means and standard errors of the I-V interval at different repetition rates of clicks (≥40 dB above BAER threshold) during the first month after birth in term infants after hypoxia-ischemia. The symbols in sequence from left to right represent the data of infants after hypoxia-ischemia on day 1, normal control infants on days 1 to 3, infants after hypoxia-ischemia on days 3, 5, 7, and 10, the control infants on days 10 to 15, infants after hypoxia-ischemia on days 15 and 30, and the controls on day 30.
Abbreviation: BAER, brainstem auditory evoked response.
There are limited reports that used SSEPs to assess perinatally hypoxic-ischemic brainstem lesions. Gibson et al. (1992) studied the median nerve SSEPs in 30 asphyxiated term infants over the course of their encephalopathy and until discharge (29). Three types of responses were noted: normal waveform, abnormal waveform, or absence of cortical response. During the follow up, nine infants died of their asphyxial illness and one of spinal muscular atrophy. Of the 20 survivors, 3 had cerebral palsy (CP), 4 had minor abnormalities, and 13 were neurodevelopmentally normal. The early SSEP results were closely correlated with the outcome. All 13 infants with normal outcome had normal SSEPs by 4 days of age, whereas those with abnormal or absent responses beyond 4 days had abnormal outcomes.
Using multimodality evoked potentials (MMEPs), Scalais et al. (1998) assessed cerebral function in 40 hypoxic-ischemic term or near-term neonates during the first week of life in order to predict the neurological outcome (30). A three-point grading system registered mild, moderate, or severe abnormalities in SSEPs, BAERs, and VEPs. At 24 months of corrected age, the infants were assessed with a blind protocol to determine neurological development. Grade 0 fVEPs and SSEPs were associated with a normal neurological status in 100% of the infants. Abnormal SSEPs or a total grade (VEPs + SSEPs) >1 were not associated with normal outcomes. Normal BAERs did not predict a normal outcome, but severely abnormal BAERs did predict an abnormal outcome. There was a significant correlation between EP (VEPs + SSEPs) grade, Sarnat stage, and clinical outcome. Compared with Sarnat scoring, both fVEPs and SSEPs are more accurate as prognostic indicators. EPs (VEPs + SSEPs) also are more accurate in predicting the ultimate neurological outcome.
The predictive value of early EP testing for neurodevelopmental outcome after perinatal asphyxia seems to be rather limited. Recently, Julkunen et al. (2014) studied SSEPs, BAERs, EEG, and Doppler in 30 asphyxiated term infants during the first 8 days (31). Cerebral blood flow velocities (CBFVs) were measured from the cerebral arteries using pulsed Doppler at 24 hours of age. EEG, EPs, Doppler findings, symptoms of hypoxic-ischemic encephalopathy, and their combination were evaluated in predicting a 1-year outcome. An abnormal EEG background predicted a poor outcome in the asphyxia group with a sensitivity of 67% and 81% specificity and an abnormal SSEP with 75% and 79%. Combining increased systolic CBFV with abnormal EEG or SSEP improved the specificity, but not the sensitivity. The predictive values of abnormal BAER and VEP results were poor. A normal EEG and SSEP predicted a good outcome in the asphyxia group with sensitivities from 79% to 81%. The combination of normal EEG, normal SSEP, and systolic CBFV less than 3 standard deviations (SD) predicted a good outcome with a sensitivity of 74% and 100% specificity. The authors concluded that combining abnormal EEG or EP findings with increased systolic CBFV did not improve prediction of a poor 1-year outcome in asphyxiated infants. A normal SSEP and normal EEG combined with systolic CBFV less than 3 SD at about 24 hours can be valuable in the prediction of normal 1-year outcome for term infants after asphyxia.
BILIRUBIN ENCEPHALOPATHY
Infants with bilirubin encephalopathy are lethargic, hypotonic, or hypertonic. They manifest a high-pitched cry, opisthotonus, seizures, and may even die. Patients surviving kernicterus have severe permanent neurologic symptoms (choreoathetosis, spasticity, hearing loss, ataxia, mental retardation). Less severe injury is associated with mild neurological abnormalities, including hearing loss. BAER has long been used as an important tool to study and evaluate bilirubin neurotoxicity in the brain and the auditory system and assess therapeutic (phototherapy and exchange transfusions) effect on the impaired brainstem and auditory function in neonatal hyperbilirubinemia (32–38). The majority of investigators found an increase in wave latencies and the I-V interval, although a few others reported no significant BAER abnormalities. In our BAER study of 90 term neonates with hyperbilirubinemia, 18% had an abnormal increase in the I-V interval, suggesting brainstem impairment (39). We further noticed that the increase is more significant in MLS BAERs than in conventional BAERs (Figure 12.6) (40). Both I-III and III-V intervals were increased significantly at higher rates of 455/sec and 910/sec. These BAER abnormalities were generally more significant at higher levels of total serum bilirubin than at lower levels (Figure 12.7), suggesting that the severity of BAER abnormalities and, in turn, auditory brainstem impairment is related to, though not completely in parallel with, the severity of hyperbilirubinemia. In addition, BAER wave amplitudes in neonates with hyperbilirubinemia are often reduced, suggesting that brainstem auditory electrophysiology is depressed (20,41). These BAER abnormalities are valuable markers or indicators of bilirubin ototoxicity or neurotoxicity. The degrees of these abnormalities are correlated with the severity of neonatal hyperbilirubinemia, though they may not precisely indicate the severity. The neurotoxic effect of hyperbilirubinemia on the auditory system could be transient, provided prompt treatment is initiated. Following exchange transfusions, the BAER abnormalities in infants with severe hyperbilirubinemia usually promptly returns to normal or near normal (37,42,43). The prompt recovery of BAERs following the decrease in the level of blood bilirubin further indicates that BAER is a sensitive test to assess the neurotoxic effect of neonatal hyperbilirubinemia and provide a valuable guide for early recognition and close monitoring of bilirubin neurotoxicity.
FIGURE 12.6 Boxplot of I-V interpeak intervals (bold line across the box, median; box, 25th and 75th centile; extensions, the largest and smallest values) at various click rates in neonates with HBN and age-matched normal controls. The I-V interval is longer in neonates with HBN than in age-matched normal controls at all click rates, which was particularly obvious at 455/sec and 910/sec.
Abbreviation: HBN, hyperbilirubinemia.
There is controversy over the use of SSEPs in assessing bilirubin neurotoxicity, as the lemniscal pathways that generate the SSEPs are not involved in kernicterus. Silver et al. (1996) described postnatal development of SSEPs in Gunn rats and the effect of jaundice (44). They found no effect of jaundice on the SSEPs in young jaundiced (jj) rats (16–28 d). However, adult (3–4 months) jj rats had prolonged latencies and decreased amplitudes of the P2 component of the SSEPs compared with adult nonjaundiced (Jj) rats. These changes in the SSEPs of jaundiced rats may reflect a synaptic lesion in these animals, possibly due to cumulative and/or progressive damage induced by bilirubin during the first 3 months of life. After sulfadimethoxine administration, marked latency prolongations (2%–6%) were observed in the early components of SSEPs in young (3-wk-old) jj (but not Jj) rats, as early as 2 hours after injection. These changes, which became more severe (4%–10%) with time, seem to be mostly peripheral. It appears that the SSEP may be a sensitive marker for the massive entry of bilirubin into the nervous system and could serve as part of an evoked potential battery (in addition to VEPs and BAERs) in assessing bilirubin-induced neurotoxicity in jaundiced newborns and infants (44).
FIGURE 12.7 Scatterplot of the I-V interval at 455/sec, with regression line against TSB. The interval is increased with the increase in TSB level.
Abbreviation: TSB, total serum bilirubin.
In 16- to 17-day-old jaundiced (jj) Gunn rats, Shapiro (2002) analyzed serial BAERs and SSEPs up to 8 hours after acute bilirubin toxicity (45). SSEPs to median nerve stimulation were recorded from surface electrodes over the brachial plexus (Erb’s) and contralateral parietal cortex and subtracted to obtain CCT. No significant change was seen in CCT in the SSEPs, whereas the BAERs were significantly abnormal and even abolished in some rats. When the injection of sulfonamide induced significant peripheral and central BAER abnormalities in jaundiced rats, no SSEP abnormalities occurred. The authors concluded that the SSEPs can assess proprioception but not other somatosensory functions or sensory integration, and BAER findings can sensitively reflect selective acute bilirubin toxicity for the auditory brainstem.
BRONCHOPULMONARY DYSPLASIA
Neonatal CLD, particularly bronchopulmonary dysplasia (BPD)—a severe type of CLD, is a major lung disease and one of the greatest risk factors of neurologic impairment and developmental deficits in very preterm or very low-birth-weight infants (46,47). BAERs were not studied in neonatal CLD or BPD until recent years (14,15,19,48). In conventional BAERs, infants with neonatal CLD show an increase in wave V latency and in I–V and III-V interpeak intervals (Figure 12.8) (48). In MLS BAERs, although there are no major abnormalities in wave I and III latencies and I–III intervals, wave V latency, I–V and particularly III–V intervals, and III–V/I–III interval ratios are all increased significantly (Figures 12.9 and 12.10) (14,15). The fundamental abnormality is a significant increase in III-V interval, a BAER variable that reflects the functional status of the more central regions of the brainstem. These abnormal findings in BAER and particularly MLS BAER indicate that CLD or BPD exerts a major damage to myelination and synaptic function of the more central regions of the immature brainstem (14,15). On the other hand, peripheral neural function is relatively intact, suggesting normal neural conduction along the more peripheral or caudal regions of the brainstem. No major abnormality can be seen in the amplitudes of MLS BAER wave components (Figures 12.9 and 12.10). It appears that neonatal CLD or BPD does not cause any major damage to neuronal function. The abnormalities in CLD infants mostly resolve several weeks after term.
FIGURE 12.8 BAER recordings at term at different rates of clicks in a normal term infant (A, female) and a very preterm infant with neonatal CLD (B, female, 29-wk gestation). Compared to the recordings in the term infant, the very preterm infant with CLD shows an increase in wave V latency, I-V, and, particularly, III-V intervals at all the 21/sec, 51/sec, and 90/sec clicks, with no abnormality in the amplitudes of BAER waves I, III, and V at any click rates.
Abbreviations: BAER, brainstem auditory evoked response; CLD, chronic lung disease.
Jiang et al. found distinct differences in the BAER and MLS BAER abnormalities between neonatal CLD or BPD and perinatal asphyxia. In infants after asphyxia, there is a significant increase in both peripheral (I-III interval) and central (III-V interval) components of BAERs and MLS BAERs (Figure 12.10) (6,7,14,26). This indicates that perinatal asphyxia affects both the peripheral and central regions of the brainstem, whereas neonatal CLD or BPD affects the more central regions of the brainstem, with no significant effect on the peripheral regions (14,15,48). In addition, infants after perinatal asphyxia show significant reduction in the amplitude of wave V at higher rates of clicks. This suggests that there is also major neuronal impairment in the brainstem after asphyxia, in contrast to no major neuronal impairment in CLD or BPD (13,19).
BRAINSTEM GLIOMAS
Brainstem gliomas are tumors arising from glial cells and mostly seen in children or adolescents. The tumors account for approximately three-fourths of the brainstem tumors in children. They are highly invasive, infiltrating the brainstem, which often makes total surgical removal impossible. BAER test is a valuable neurodiagnostic method, particularly when auditory pathways are primarily involved and when diagnosis via neuroradiology or clinical findings is not conclusive. BAERs and SSEPs, as electrophysiological measures, provide information on the functional status of the CNS, specifically the brainstem, whereas CT and MRI, as conventional neuroradiology, shows the structure.
BAERs are useful in follow up of the effect of the preoperative chemotherapy or the progression of the inoperable tumors. BAERs also proved effective in the assessment of postoperative neurological complications. For example, bilateral symmetrical prolongation of interpeak intervals and wave V reduction occur in postoperative occlusive hydrocephalus, with clinical signs of increased intracranial pressure. Unilateral prolongation of interpeak intervals occurs during irradiation or chemotherapy after medulloblastoma removal as signs of cerebral edema.
From mid-1970s till late 1990s, there were a number of reports on BAERs and SSEPs in pediatric patients with brainstem tumors. When the lesion involved the pons, almost all cases showed abnormal BAERs. For example, Nodar et al. (1980) described BAERs in seven children (aged 2.5 to 13 years) with diagnosed brainstem neoplasms, including one with astroependymoma, one with medulloblastoma, one with intraparenchymal ependymoma, and two with glioma (49). No tumor type was specified for the other two patients. All children showed some of the following BAER abnormalities, including absolute wave latency, wave latency difference between ears, wave I-V interval, response stability, amplitude, morphology, and presence of waves. In each case, the BAER test results clearly indicated the site of the lesion as determined by CT and observation during surgery. In 14 children with clinical and radiological evidence of brainstem glioma, Weston et al. (1986) found that all with pontine involvement (13 of the 14) had abnormalities of wave V (delayed latency, reduced or absent amplitude) (50). BAER abnormalities were consistently found in the stimulus ear ipsilateral to the tumor, though they were not invariably related to tumor size. The findings were consistent with intrinsic brainstem lesions in all except one case with a glioma, which was shown to be located predominantly in the medulla oblongata.
FIGURE 12.9 MLS BAER recordings from a normal control (A), an infant with CLD (B), and an infant after perinatal asphyxia (C). Compared to the control (A), the infant with CLD (B) shows a significant increase in I-V and, particularly, III-V intervals, but no major amplitude reduction for all waves I, III, and V; the infant after asphyxia (C), however, shows a significant increase in all I-V, I-III, and III-V intervals and a major amplitude reduction for all waves, particularly for wave V at very high rates (455/sec and 910/sec).
Abbreviations: BAER, brainstem auditory evoked response; CLD, chronic lung disease; MLS, maximum length sequence.
FIGURE 12.10 Boxplot of BAER interpeak intervals (bold line across the box, median; box, 25th and 75th centile; extensions, the largest and smallest values) at various click rates in normal term infants (A), infants with CLD (B), and infants after asphyxia (C). Interval I-V is similar in CLD infants and asphyxiated infants, although the interval in both groups is significantly longer than in normal controls (A). Interval I-III in asphyxiated infants is significantly longer than in CLD infants whose I-III interval is similar to that in normal controls at all click rates 21/sec to 910/sec (B). In contrast, the III-V interval in CLD infants is significantly longer than in asphyxiated infants at all click rates 21/sec to 910/sec, although the interval in both groups is significantly longer than in normal controls (C).
Rotteveel et al. (1985) reported their comprehensive clinical study of the contribution of SSEPs and BAERs in the primary diagnosis and follow-up of 26 children with infratentorial and supratentorial tumors (51). The 14 infratentorial tumors included six medulloblastomas (all but one in the fourth ventricle), four gliomas, two arachnoid sarcomas, two astrocytomas, and a ganglioneuroblastoma. The 12 supratentorial tumors were three craniopharyngiomas, three astrocytomas (grade I, II, and III) in different regions, an optic nerve astrocytoma, a lipoma, an ependymoma, a chromophobe adenoma, a germinoma, and an unspecified tumor in the left thalamus. Occasional BAER abnormalities in the supratentorial group were associated with brainstem dysfunction secondary to intracranial pressure effects (eg, compression of inferior colliculus). The majority of children in the infratentorial group had BAER abnormalities, including delayed interpeak intervals and total absence of waves. Abnormalities were not uncommon for BAERs elicited by stimulation of the ear contralateral to the tumor (pressure of effect). Tumors near the eighth nerve typically produced asymmetric findings, whereas symmetric findings were related to tumors in the midline fourth ventricle region. Serial BAERs had clinical value in documenting the progression of pathophysiologic changes, including development of hydrocephalus. The authors emphasize the clinical importance of BAERs by stating that large tumors are easy to detect, but removal surgically is difficult or impossible. With BAERs, it may be possible to detect small tumors in some cases, which permits effective surgical therapy. The BAERs were sensitive for supratentorial pressure effects and for local and distant posterior fossa tumor effects. The SSEPs, especially the specific complex, showed a latency increase in patients with supratentorial and brainstem mass lesions involving, directly or distantly, the somatosensory tracts. In the follow-up of children, EPs offer a good method of detecting tumor recurrence, whereas neuroradiological procedures may be obscured by surgical or radiation artifacts.
Fischer et al. (1982) summarized BAER findings for a group of 66 patients with various CNS tumors, including eight meningiomas, two fourth ventricle ependymomas, five cerebellar tumors, two third ventricle colloid cysts, a craniopharyngioma, a cyst, a pinealoma, an astrocytoma, a germinoma, and over 40 unspecified tumors involving the brainstem (52). The effect of posterior fossa meningiomas and cerebellar tumors on the BAERs depended on the size of the tumor and its location relative to auditory structures. CT information was generally more useful than BAERs in characterizing location and size. The authors stated that useful, and sometimes unique, diagnostic information was obtained from BAERs for 36 patients, including the evidence of a tumor by BAERs before CT scanning.
In 1997, Fukuda et al. described SSEPs elicited by median nerve stimulation in 17 patients with brainstem tumor (53). Of a total of 35 SSEP records in these patients, 13 had a normal CCT; eight, prolonged CCT; nine, abolished N20 potential; four, abolished N20 and N18 potentials; and one, abolished N20, N18, and P14 potentials. These SSEP groups were correlated with the size and location of the brainstem tumor on MRI. N20 potentials were unchanged in latency in patients with small localized gadolinium (Gd)-enhanced lesions, but were abolished in patients with tumors extending to the dorsal pons and the upper medulla oblongata. The extent of nonenhanced low-intensity lesions did not correlate with the changes in the N20 potentials. The degree of the impairment of the N20 potentials reflected the severity of the clinical symptoms. The N20 potential can evaluate brainstem dysfunction caused by brainstem tumor. In four patients whose extensive tumors (one Gd-enhanced lesion, three low-intensity lesions) involved not only the pons but also the medulla oblongata, the N18 potentials, probably generated from the medulla oblongata, were abolished.
SUPRATENTORIAL MASS LESIONS
Supratentorial mass lesions may produce neurological dysfunction by two mechanisms: cerebral hemispheric damage from the primary lesion itself and secondary brainstem damage from displacement, tissue compression, swelling, and vascular stasis. Early in 1980, MacKay et al. (1980) conducted an animal experiment to examine BAER changes due to acutely expanding mass lesions (54). In anesthetized cats, after posterior fossa balloon catheters were inflated slowly, there were reliable BAER changes, which occurred before the Cushing response and were reversible by balloon deflation. Since BAER changes precede the agonal changes of the Cushing response, serial BAER recording in patients with known or suspected posterior fossa masses may be useful in the management of these lesions.
In 1993, Inao et al. quantitatively measured brainstem distortion and neural dysfunction in 25 cases of chronic subdural hematoma (55). The horizontal and rotational brainstem displacements were measured on axial and coronal MRI in all patients preoperatively, and BAERs were obtained in 11 cases. BAER wave latencies and I-V interpeak interval were increased, which was correlated with septum shift. The increased wave V latency indicated that brainstem rotation in the coronal plane reflects upper brainstem dysfunction most closely. The correlation between brainstem displacement shown on MRI and the increase in BAER wave latencies and I-V interval demonstrated a good relationship between anatomical and physiological changes in the brainstem that are associated with supratentorial lesions.
Krieger et al. (1993) examined the clinical relevance of BAERs, along with pupillary responses and intracranial pressure monitoring, in 55 comatose patients (9–70 years) with acute supratentorial mass lesions (56). BAERs were rated “bilaterally normal,” “unilaterally abnormal,” or “bilaterally abnormal.” BAER categories correlated significantly with pupillary abnormalities and increased intracranial pressure but did not predict outcome. Increased intracranial pressure was associated with abnormalities in BAERs. The authors concluded that BAERs can be used to support the clinical relevance of abnormal pupillary status and increased intracranial pressure but have no prognostic value. Examination of BAERs, along with pupillary response and intracranial pressure monitoring, provides useful information for managing patients with supratentorial mass lesions.
Later, Krieger et al. (1995) examined the relevance of serial BAERs and SSEPs and clinical parameters (pupillary response and intracranial pressure) in patients with acute supratentorial mass lesions (57). BAER and SSEP results were ranked into three categories: (a) normal on both sides; (b) abnormal or absent on one side; and (c) EPs on both sides abnormal or absent. BAER results were correlated with intracranial pressure values during and at the termination of intracranial pressure therapy and with pupillary findings only at the time of termination of intracranial pressure therapy. No correlation was found between SSEPs and clinical parameters. Pupillary responses indicated a good or poor recovery during and at the termination of intracranial pressure therapy. BAERs and intracranial pressure values distinguished between good and poor outcome only at the termination of intracranial pressure therapy. SSEPs did not predict outcome. Thus, shortly after the manifestation of supratentorial mass lesions, the results of EPs and clinical parameters indicate increased intracranial pressure and incipient transtentorial herniation but do not predict sequelae. After institution of effective therapy, BAER and pupillary abnormalities are valuable prognostic predictors. In contrast, SSEPs reflect neither therapeutic efficacy nor outcome.
CEREBRAL PALSY (CP)
CP, a motor disease in children, is characterized by nonprogressive but varied neurologic deficits, including cerebellar ataxia, athetosis and spasticity, and sometimes associated neurologic disorders (epilepsy and mental retardation). CP is often associated with various adverse prenatal or perinatal risk factors, eg, asphyxia, extreme prematurity, retarded growth, viral infections in utero, and neonatal meningitis. The most common specific risk factor is cerebral anoxia-hypoxia occurring in utero, at delivery, or immediately following birth. It is important to realize, however, that most children with these risk factors do not have subsequent evidence of CP, and, conversely, most of the children with CP have had none of the risk factors.
As CP is a motor disease, normal findings would be expected for sensory EPs. However, abnormal BAER findings have been reported by some investigators (17,58,59). SSEPs (and VEPs) have also been studied by some investigators in children with CP and some abnormalities were found (60,61). Nevertheless, due to the lack of full cooperation by the children with CP during these tests, it is difficult to obtain reliable SSEP data and the results may vary considerably. By comparison, BAER testing does not necessarily require full cooperation of the subject, which makes it particularly suitable for children with CP who cannot fully cooperate with any test. Using BAERs, some investigators have devoted their studies to detect auditory and brainstem abnormalities or deficits in children with CP (17,58,59,62–64). These investigators have explored some BAER abnormalities in these children. Among the abnormalities, a major finding is an amplitude reduction in BAER late wave components (17,58,59). This interesting finding indicates that brainstem auditory electrophysiology is depressed in children with CP. The amplitude reduction is mainly seen in children who survived perinatal or postnatal asphyxia, though it is also seen in those who had other heterogeneous etiologies.
In children with CP, the BAER waveform, particularly the later components, tends to be depressed, which occurs in about 40% of the children (59). The main response abnormality is a significant reduction in the amplitude of wave V. On the other hand, abnormal findings in the interpeak intervals are rare. These findings are in contrast to common findings in the responses in infants and children with progressive neurologic abnormalities following conditions such as perinatal asphyxia and BPD, whereby the major BAER abnormality is a significant increase in I-V and, particularly, III-V intervals (1). Other abnormalities in children with CP include decreased V/I amplitude ratio, missing waves, prolonged I-V interval, and increased interaural difference in the I-V interval. These findings indicate that some children with CP have a depressed auditory electrophysiology, which may be due to decreased or altered neural firing or synchrony in the auditory brainstem. On the other hand, neural transmission along the auditory brainstem in CP is largely intact and rarely compromised, although it could be damaged at the active stages of original neuropathology such as perinatal asphyxia that results in CP. In infants after perinatal asphyxia, the amplitude reduction in wave V was persistent during the first month after birth. Therefore, the reduction appears to be persistent during the postnatal period in children who have CP. The persistent reduction in wave V amplitude in early life might be a valuable early indicator for developing CP later.
Jiang and Tierney (1996) found that in the majority of children who survive asphyxia, whether it occurred perinatally or postnatally, BAERs were normal, without any significant abnormality. However, a small proportion of the children had BAER abnormality, suggesting a residual brainstem lesion (17). The major abnormality was a reduction in wave V amplitude, followed by a decrease in the V/I amplitude ratio and a prolongation of the I-V interval. Missing waves were also seen in a few children. On the other hand, abnormalities in BAER wave latencies and interpeak intervals were rare. Amplitude reduction was less significant for BAER waves I and III, ie, waves prior to wave V. The abnormal BAER results or patterns could be categorized into five patterns, which are shown in Figure 12.11. The most frequently seen patterns were pattern A (combined wave V amplitude reduction and V/I amplitude ratio decrease) and pattern B (combined wave V amplitude reduction and/or V/I amplitude ratio decrease and I-V interval prolongation). Other abnormal patterns (C, D, and E) were relatively rare. Thus, the commonest BAER abnormality was the amplitude reduction in wave V, often accompanied by a decrease in V/I amplitude ratio or a prolongation in the I-V interval. The prevalence of prolonged I-V interval was less than half of the amplitude reduction in wave V. Apparently, perinatal and postnatal asphyxia can exert a long-term effect on the brainstem, resulting in residual neuronal dysfunction of the brainstem, evidenced by the significant reduction in wave V amplitude, but it does not appear to exert any major long-term effect on neuronal transmission, evidenced by the normal I-V interval in most cases. These findings are quite different from those seen shortly after the episode of asphyxia where the major abnormality in BAER measures usually is a prolonged I-V interval, followed by reduced wave V amplitude and V/I amplitude ratio. The prolonged I-V interval seen in the acute phase of asphyxia recovers much faster than the reduced amplitude of wave V. It seems that asphyxia is unlikely to exert any major long-term effect on nerve conduction and synaptic transmission or myelination in the brainstem.
FIGURE 12.11 Abnormal patterns of the central BAER components in children who survive asphyxia. The dashed traces are the age-matched controls. (A) Combined wave V amplitude reduction and V/I amplitude ratio decrease. (B) Combined wave V amplitude reduction and/or V/I amplitude ratio decrease and I-V interval prolongation. (C) Wave V amplitude reduction only. (D) V/I amplitude ratio decrease only. (E) I-V interval prolongation only.
Following perinatal asphyxia, there are discrete brainstem lesions involving auditory centers, including loss of neurons with gliosis or ischemic cell changes in the inferior colliculus and superior olivary complex (24). The major underlying mechanism for the persistent reduction of wave V amplitude is most likely to be fewer generating neurons and/or fewer fibers conducting the volley in the generators of the BAER wave V following hypoxic-ischemic insults to the brainstem. Wave V originates in the high pons or low midbrain. Compared to the earlier BAER waves I to IV, wave V has its origin in the most rostral part of the auditory brainstem. The persistent reduction of wave V amplitude in children with CP after asphyxia suggests that compared to the more caudal regions, the lesion in the rostral regions of the brainstem is more profound and/or that the recovery of the damaged neurons in the rostral brainstem is more incomplete or retarded.
OTHER DISORDERS
There are a number of other pediatric disorders that may directly or indirectly involve the brainstem, resulting in abnormalities in EPs, particularly BAERs. These include infections and inflammatory diseases (eg, meningitis, brain abscesses, viral infections, Kawasaki disease, fungal infections), neurodevelopmental disorders (eg, hydrocephalus, myelomeningocele and Arnold-Chiari malformation, autism), metabolic diseases (eg, storage diseases, phenylketonuria, diabetes mellitus, maple syrup urine disease, mitochondrial encephalomyopathies), degenerative diseases (eg, sclerosing panencephalitis, hereditary motor sensory neuropathy [Dejerine-Sottas disease]). In the following text are two samples of storage diseases: Leigh’s syndrome and Gaucher’s disease.
Leigh’s Syndrome
Mitochondrial disorders are a varied collection of progressive diseases that have in common morphological, biochemical, and/or genetic abnormalities of the mitochondria. Features include the accumulation of mitochondria and metabolic disorders. Among the others, Leigh’s syndrome (a subacute necrotizing encephalopathy that can affect the brainstem tegmentum) has been widely studied with BAERs (65–69). The responses are often markedly abnormal, including prolonged wave latencies and interpeak intervals. Sakai et al. (2004) reported two sisters (age 4 and 11 years) with Leigh’s syndrome with a T8993G point mutation of mitochondrial DNA (67). Clinical medical findings included low-density areas in the basal ganglia and posterior limb of the internal capsule by CT, high levels of lactate and pyruvate in the spinal fluid, muscle weakness, ataxia, retinitis pigmentosa, epileptic seizures, and mental retardation. The children were referred to the authors because they responded poorly to sounds. The older sister yielded essentially normal BAER findings. Hearing sensitivity, however, progressively worsened in the younger sister, associated with delayed BAER latencies. BAER threshold fluctuated remarkably during a 3-year follow-up, suggesting that her hearing problems may well have been caused by both cochlear nerves and retrocochlear or brainstem lesions.
In 1993, Yoshinaga et al. described a case of a 7-month-old girl with Leigh’s syndrome diagnosed with neurophysiologic, radiologic, enzymatic, biochemical, and molecular studies (68). Over time, the patient developed additional clinical symptoms of brainstem dysfunction (irregular respiration and dysphagia), hypotonia, and then seizures and tonic spasms. Blood analysis showed elevated levels of lactate and pyruvate and a mitochondrial DNA point mutation at 8993 in the patient and the mother. BAER abnormalities were among the first clinical signs found in this patient. Later in 2003, to assess the utility of BAERs in diagnosing brainstem changes in patients with Leigh’s syndrome, Yoshinaga et al. performed a longitudinal study of five patients with Leigh’s syndrome using both BAER and neuroimaging techniques (CT and MRI; 69). The brainstem components of the initial BAERs were abnormal in all five patients. In four patients, these abnormal findings preceded any clinical signs of brainstem impairment. Improvements in clinical findings were reflected in improvements in BAER findings in three patients. In one of these three patients, improvements in clinical findings were also reflected in improvements in MRI findings. In the other two patients, MRI findings showed no improvements, despite the improvements in clinical findings. In two of the patients, BAERs clearly revealed functional improvements in the brainstem, which were not revealed by MRI. Therefore, BAERs are an essential diagnostic technique for patients with Leigh’s syndrome.
With SSEPs and BAERs, Araki et al. (1997) evaluated brainstem dysfunction in a girl with Leigh’s syndrome (65). Serial analysis of BAERs and SSEPs showed progressive disturbances of the brainstem wave components. The authors also performed neuroradiological and other neurophysiological tests, including brain MRI, single-photon emission computed tomography, electrically elicited blink reflexes, and all-night polysomnography and detected many abnormalities. They concluded that the multimodality tests in combination with neuroradiologic examinations are useful for assessing brainstem dysfunction in patients with Leigh’s syndrome.
Gaucher’s Disease
This is a rare lipid storage disease due to an enzyme deficiency and the accumulation of ganglioside (cerebroside) in the nervous system as well as the viscera (liver, spleen, lungs). There is diffuse neuronal damage and progressive and marked CNS dysfunction (eg, muscle paralysis), which usually commences at 6 months of life. The infants often die at 1 year. The patients show gross BAER abnormalities (70–74). Kaga et al. (1982) recorded serial BAERs from an infant with Gaucher’s disease (72). The disease started at 3 months. The infant first showed stridor, strabismus, failure to thrive, and inguinal hernia, and then muscular rigidity, ocular palsies, and respiratory failure. The initial BAER recordings at 6 months revealed a prolongation or increase in the latencies of waves I, II and III, and disappearance of waves after wave IV. Subsequent BAER recordings at 8 months showed that these wave latencies became further prolonged and wave components after wave III disappeared as neurologic status deteriorated. He died of respiratory failure due to central origin developed. In autopsy, there was no obvious structural damage in the auditory brainstem, suggesting that metabolic and electrophysiologic changes precede histopathologic changes. In a later study, Kaga et al. (1998) correlated abnormal BAER findings (only wave I and wave II were recorded) with neuropathology (73). An autopsy revealed there were Gaucher’s cells in the cerebrum and thalamus, dorsal brainstem gliosis, and pathologic cells in the superior olivary complex with marked gliosis in the cochlear nucleus.
In eight children with type III Gaucher’s disease, Campbell et al. (2003) found a diverse collection of BAER abnormalities, including absence of all waves except wave I (a common pattern) and delays in later waves (III and V; 70). The abnormalities progressively deteriorated, reflecting underlying subclinical brainstem deterioration. To detect early subclinical nervous dysfunction in Gaucher’s disease type 1, Perretti et al. (2005) examined multimodality EPs in 17 patients with the disease. Five patients (31.2%) showed clear BAER abnormalities, the most frequent abnormality being a bilateral increased I-III interpeak latency (75). SSEP abnormalities were seen in three patients (18.7%), including an increased N13-N20 interval in two patients and an irreproducible N13 wave in one patient. In addition, the authors found a delayed latency of the P100 wave in VEPs in four patients (25%), and the central motor evoked potential was abnormal in nine patients (69.2%). The multimodal evoked potential approach provides information about nervous subclinical damage in Gaucher’s disease type 1 and helps early detection of subclinical neurologic dysfunction.
CONCLUSION
Lesions in the human brainstem may affect the auditory and somatosensory pathways. BAERs and SSEPs are the two main types of ERs to evaluate the functional status of the auditory and somatosensory input systems at different levels of the CNS. In clinical application, evaluation with the two methods are often less costly than other evaluation techniques such as MRI. BAERs are the most commonly used electrophysiological test to detect intrinsic or extrinsic lesions of the brainstem and has been widely used in pediatric, particularly neonatal, clinics. The BAERs are unaffected by sleep state or sedation. This significant advantage has led to the wide use of BAERs in pediatric neurology to assess the functional integrity of the brainstem and detect brainstem lesions in many pathological conditions that affect the brainstem auditory pathway. SSEPs are useful for clinical diagnosis of brainstem lesions in patients with various neurologic diseases that affect the somatosensory pathways in the brainstem. The SSEP components generated in the brainstem are mediated entirely by the dorsal columns (posterior columns) of the spinal cord, the fasciculus cuneatus for upper limb SSEPs and the fasciculus gracilis for lower limb SSEPs. Diseases of the dorsal columns in which joint position sense and proprioception are impaired are invariably associated with an abnormal SSEP.
Among the EPs, BAERs are most affected by brainstem lesions. Over the last three decades, BAERs have been the focus of noninvasive electrophysiological examinations of the functional integrity of the brainstem in pediatric neurology. Compared with SSEPs (and other evoked potentials, eg, VEPs), BAERs are simpler to record and are unaffected by sleep state or sedation. These advantages make BAERs particularly suitable for use in very young infants and sick children. Numerous clinical studies have documented that BAERs are an important tool and adjunct to assess and detect neuropathology that involves the brainstem auditory pathway in a wide range of pediatric diseases. As a relatively new technique, the MLS BAER has recently been introduced in pediatric, particularly neonatal, neurology. There is increasing evidence suggesting that the MLS is a promising technique to enhance the diagnostic value of BAERs for brainstem lesions in pediatric neuropathology and to provide valuable information for the clinical management of brainstem lesions. In terms of simplicity of clinical application, conventional BAERs remain a very useful test for pediatric brainstem lesions, whereas MLS BAER is recommended to enhance the detection of some early or subtle neuropathology that may not be detected with conventional BAERs (1,4). More clinical studies of MLS BAER are warranted in a wide range of pediatric diseases that may result in brainstem lesions.
REFERENCES
1. Jiang ZD. Brainstem auditory evoked response in neonatal brain damage. Curr Trends Neurol. 2013;7:1–14.
2. Wilkinson AR, Jiang ZD. Brainstem auditory evoked response in neonatal neurology. Semin Fet Neonatol Med. 2006;11:444–451.
3. Hall III JW. ABR: Pediatric clinical application and populations. In: Hall III JW, ed. New Handbook of Auditory Evoked Responses. Boston, MA: Pearson Education; 2007:313–365.
4. Jiang ZD. Maximum length sequence technique improves detection of brainstem abnormalities in infants. In: Lawson PN, McCarthy EA, eds. Pediatric Neurology. New York, NY: Nova Science Publishers; 2012:1–38.
5. Jirsa RE. Maximum length sequences-auditory brainstem responses from children with auditory processing disorders. J Am Acad Audiol. 2001;12:155–164.
6. Jiang ZD, Brosi DM, Shao XM, Wilkinson AR. Maximum length sequence brainstem auditory evoked response in term infants after perinatal hypoxia-ischaemia. Pediatr Res. 2000;48:639–645.
7. Jiang ZD, Brosi DM, Wang J, et al. Time course of brainstem pathophysiology during first month in term infants after perinatal asphyxia, revealed by MLS BAER latencies and intervals. Pediatr Res. 2003;54:680–687.
8. Jiang ZD. Neural conduction impairment in the auditory brainstem and the prevalence in term babies in intensive care unit. Clin Neurophysiol. 2015;126:1446–1452.
9. Lasky RE, Perlman J, Hecox K. Maximum length sequence auditory evoked brainstem responses in human newborns and adults. J Am Acad Audiol. 1992;3:383–389.
10. Picton TW, Champagne SC, Kellett AJC. Human auditory potentials recorded using maximum length sequences. Electroencephalogr Clin Neurophysiol. 1992;84:90–100.
11. Jiang ZD, Brosi DM, Li ZH, et al. Brainstem auditory function at term in preterm babies with and without perinatal complications. Pediatr Res. 2005;58:1164–1169.
12. Jiang ZD, Xu X, Brosi DM, et al. Sub-optimal function of the auditory brainstem in term neonates with transient low Apgar scores. Clin Neurophysiol. 2007;118:1088–1096.
13. Jiang ZD, Brosi DM, Shao XM, Wilkinson AR. Sustained depression of brainstem auditory electrophysiology during the first month in term infants after perinatal asphyxia. Clin Neurophysiol. 2008;119:1496–1505.
14. Jiang ZD, Brosi DM, Wilkinson AR. Differences in impaired brainstem conduction between neonatal chronic lung disease and perinatal asphyxia. Clin Neurophysiol. 2010;121:725–733.
15. Wilkinson AR, Brosi DM, Jiang ZD. Functional impairment of the brainstem in infants with bronchopulmonary dysplasia. Pediatrics. 2007;120:362–371.
16. Jiang ZD, Wang C, Chen C. Neonatal necrotizing enterocolitis adversely affects neural conduction of the rostral brainstem in preterm babies. Clin Neurophysiol. 2014;125:2277–2285.
17. Jiang ZD, Tierney TS. Long-term effect of perinatal and postnatal asphyxia on developing human auditory brainstem responses: brainstem impairment. Int J Pediatr Otorhinolaryngol. 1996;34:111–127.
18. Jiang ZD, Brosi DM, Wilkinson AR. Comparison of brainstem auditory evoked responses recorded at different presentation rates of clicks in term neonates after asphyxia. Acta Paediatr. 2001;90:1416–1420.
19. Jiang ZD, Brosi DM, Chen C, Wilkinson AR. Brainstem auditory response amplitudes in neonatal chronic lung disease and differences from perinatal asphyxia. Clin Neurophysiol. 2009;120:967–973.
20. Jiang ZD, Brosi DM, Wilkinson AR. Changes in BAER wave amplitudes in relation to total serum bilirubin level in term neonates. Eur J Pediatr. 2009;168:1243–1250.
21. Jiang ZD, Wu YY, Liu XY, Wilkinson AR. Depressed brainstem auditory function in children with cerebral palsy. J Child Neurol. 2011;26:272–278.
23. Sugama S, Eto Y. Brainstem lesions in children with perinatal brain injury. Pediatr Neurol. 2003;28:212–215.
24. Leech RW, Alvord EC Jr. Anoxic-ischemic encephalopathy in the human neonatal period: the significance of brain stem involvement. Arch Neurol. 1977;34:109–113.
25. Mietzsch U, Parikh NA, Williams AL, et al. Effects of hypoxic-ischemic encephalopathy and whole-body hypothermia on neonatal auditory function: a pilot study. Am J Perinatol. 2008;25:435–441.
26. Jiang ZD, Yin R, Shao XM, Wilkinson AR. Brainstem auditory impairment during the neonatal period in infants after asphyxia: dynamic changes in brainstem auditory evoked responses to clicks of different rates. Clin Neurophysiol. 2004;115:1605–1615.
27. Jiang ZD, Brosi DM, Wilkinson AR. Impairment of perinatal hypoxia-ischaemia to the preterm brainstem. J Neurolog Sci. 2009;287:172–177.
28. Jiang ZD, Brosi DM, Wilkinson AR. Depressed brainstem auditory electrophysiology in preterm infants after perinatal hypoxia-ischemia. J Neurolog Sci. 2009;281:28–33.
29. Gibson NA, Graham M, Levene MI. Somatosensory evoked potentials and outcome in perinatal asphyxia. Arch Dis Child. 1992;67:393–398.
30. Scalais E, François-Adant A, Nuttin C, et al. Multimodality evoked potentials as a prognostic tool in term asphyxiated newborns. Electroencephalogr Clin Neurophysiol. 1998;108:199–207.
31. Julkunen MK, Himanen SL, Eriksson K, et al. EEG, evoked potentials and pulsed Doppler in asphyxiated term infants. Clin Neurophysiol. 2014;125:1757–1763.
32. Ahlfors CE, Parker AE. Unbound bilirubin concentration is associated with abnormal automated auditory brainstem response for jaundiced newborns. Pediatrics. 2008;121:976–978.
33. Ahlfors CE, Amin SB, Parker AE. Unbound bilirubin predicts abnormal automated auditory brainstem response in a diverse newborn population. J Perinatol. 2009;29:305–309.
34. Amin SB, Ahlfors C, Orlando MS, et al. Bilirubin and serial auditory brainstem responses in premature infants. Pediatrics. 2001;107:664–670.
35. Funato M, Tamai H, Shimada S, Nakamura H. Vigintiphobia, unbound bilirubin, and auditory brainstem responses. Pediatrics. 1994;93:50–53.
36. Funato M, Teraoka S, Tamai H, Shimida S. Follow-up study of auditory brainstem responses in hyperbilirubinemic newborns treated with exchange transfusion. Acta Paediatr Jpn. 1996;38:17–21.
37. Hung K L. Auditory brainstem responses in patients with neonatal hyperbilirubinaemia and bilirubin encephalopathy. Brain Dev. 1989;11;297–301.
38. Wennberg RP, Ahlfors CE, Bickers R, et al. Abnormal auditory brainstem response in a newborn infant with hyperbilirubinemia: Improvement with exchange transfusion. J Pediatr. 1982;100:624–626.
39. Jiang ZD, Liu TT, Chen C, Wilkinson AR. Changes in BAER wave latencies in term neonates with hyperbilirubinaemia. Pediatr Neurol. 2007;37:35–41.
40. Jiang ZD, Wilkinson AR. Brainstem auditory impairment in term neonates with hyperbilirubinemia. Brain Dev. 2014;36:212–218.
41. Jiang ZD, Liu TT, Chen C. Brainstem auditory electrophysiology is supressed in term neonates with hyperbilirubinemia. Eur J Paediatr Neurolog. 2014; 18:193–200.
42. Yilmaz Y, Degirmenci S, Akdas F, et al. Prognostic value of auditory brainstem response for neurologic outcome in patients with neonatal indirect hyperbilirubinemia. J Child Neurol. 2001;16:772–775.
43. Wong V, Chen WX, Wong KY. Short- and long-term outcome of severe neonatal nonhemolytic hyperbilirubinemia. J Child Neurol. 2006;21:309–315.
44. Silver S, Sohmer H, Kapitulnik J. Postnatal development of somatosensory evoked potential in jaundiced Gunn rats and effects of sulfadimethoxine administration. Pediatr Res. 1996;40:209–214.
45. Shapiro SM. Somatosensory and brainstem auditory evoked potentials in the Gunn rat model of acute bilirubinneurotoxicity. Pediatr Res. 2002;52:844–849.
46. Baraldi E, Filippone M. Chroniclung disease after premature birth. N Engl J Med. 2007;357:1946–1955.
47. Cristea AI, Carroll AE, Davis SD, et al. Outcomes of children with severe bronchopulmonary dysplasia who were ventilator dependent at home. et al. 2013;132:e727–e734.
48. Jiang ZD, Brosi DM, Wilkinson AR. Brainstem auditory function in very preterm infants with chronic lung disease: delayed neural conduction. Clin Neurophysiol. 2006;117:1551–1559.
49. Nodar RH, Hahn J, Levine HL. Brain stem auditory evoked potentials in determining site of lesion of brain stem gliomas in children. Laryngoscope. 1980;90:258–266.
51. Rotteveel JJ, Colon EJ, Hombergen G, Stoelinga GB, Lippens R. The application of evoked potentials in the diagnosis and follow-up of children with intracranial tumors. Childs Nerv Syst. 1985;1:172–178.
52. Fischer C, Mauguière F, Echallier JF, Courjon J. Contribution of brainstem auditory evoked potentials to diagnosis of tumors and vascular diseases. Adv Neurol. 1982;32:177–185.
53. Fukuda M, Kameyama S, Honda Y, et al. Short-latency somatosensory evoked potentials in patients with brain stem tumor: study of N20 and N18 potentials. Neurol Med Chir (Tokyo). 1997;37:525–531.
54. MacKay AR, Hosobuchi Y, Williston JS, Jewett D. Brain stem auditory evoked response and brain stem compression. Neurosurgery. 1980;6:632–638.
55. Inao S, Kuchiwaki H, Kanaiwa H, et al. Magnetic resonance imaging assessment of brainstem distortion associated with a supratentorial mass. J Neurol Neurosurg Psychiatry. 1993;56:280–285.
56. Krieger D, Adams HP, Schwarz S, et al. Prognostic and clinical relevance of pupillary responses, intracranial pressure monitoring, and brainstem auditory evoked potentials in comatose patients with acute supratentorial mass lesions. Crit Care Med. 1993;21:1944–1950.
57. Krieger D, Jauss M, Schwarz S, Hacke W. Serial somatosensory and brainstem auditory evoked potentials in monitoring of acute supratentorial mass lesions. Crit Care Med. 1995;23:1123–1131.
58. Jiang ZD, Liu XY, Shi BP, et al. Brainstem auditory outcome and correlation with neurodevelopment after perinatal asphyxia. Pediatr Neurol. 2008;39:189–195.
59. Jiang ZD, Wu YY, Liu XY, Wilkinson AR. Depressed brainstem auditory function in children with cerebral palsy. J Child Neurol. 2011;26:272–278.
60. Ghasia F, Brunstom J, Tychsen L. Visual acuity and visually evoked responses in children with cerebral palsy: Gross Motor Function Classification Scale. Br J Ophthalmol. 2009;93:1068–1072.
61. Suppiej A, Cappellari A, Franzoi M, et al. Bilateral loss of cortical somatosensory evoked potential at birth predicts cerebral palsy in term and near-term newborns. Early Hum Dev. 2010;86:93–98.
62. Kaga K, Ichimura K, Kitazumi E, et al. Auditory brainstem responses in infants and children with anoxic brain damage due to near-suffocation or near-drowning. Int J Pediatr Otorhinolaryngol. 1996;36:231–239.
63. Zafeiriou DI, Andreou A, Karasavidou K. Utility of brainstem auditory evoked potentials in children with spastic cerebral palsy. Acta Paediatr. 2000;89:194–197.
64. Zhang L, Jiang ZD. Development of the brainstem auditory pathway in low birthweight and perinatally asphyxiated children with neurological sequelae. Early Hum Dev. 1992;30:61–73.
65. Araki S, Hayashi M, Yasaka A, Maruki K. Electrophysiological brainstem dysfunction in a child with Leigh disease. Pediatr Neurol. 1997;16:329–333.
66. Kaga M, Naitoh H, Nihei K. Auditory brainstem response in Leigh’s syndrome. Acta Paediatr Jpn. 1987;29:254–260.
67. Sakai Y, Kaga K, Kodama K, et al. Hearing evaluation in two sisters with a T8993G point mutation of mitochondrial DNA. Int J Pediatr Otorhinolaryngol. 2004;68:1115–1119.
68. Yoshinaga H, Ogino T, Ohtahara S, et al. A T-to-G mutation at nucleotide pair 8993 in mitochondrial DNA in a patient with Leigh’s syndrome. J Child Neurol. 1993;8:129–133.
69. Yoshinaga H, Ogino T, Endo F, et al. Longitudinal study of auditory brainstem response in leigh syndrome. Neuropediatrics. 2003;34:81–86.
70. Campbell PE, Harris CM, Harris CM, et al. A model of neuronopathic Gaucher disease. J Inherit Metab Dis. 2003;26:629–639.
71. Campbell PE, Harris CM, Vellodi A. Deterioration of the auditory brainstem response in children with type 3 Gaucher disease. Neurology. 2004;63:385–387.
72. Kaga M, Azuma C, Imamura T, et al. Auditory brainstem response (ABR) in infantile Gaucher’s disease. Neuropediatrics. 1982;13:207–210.
73. Kaga K, Ono M, Yakumaru K, et al. Brainstem pathology of infantile Gaucher’s disease with only wave I and II of auditory brainstem response. J Laryngol Otol. 1998;112:1069–1073.
74. Lacey DJ, Terplan K. Correlating auditory evoked and brainstem histologic abnormalities in infantile Gaucher’s disease. Neurology. 1984;34:539–541.
75. Perretti A, Parenti G, Balbi P, et al. Study of multimodal evoked potentials in patients with type 1 Gaucher’s disease. J Child Neurol. 2005;20:124–128.
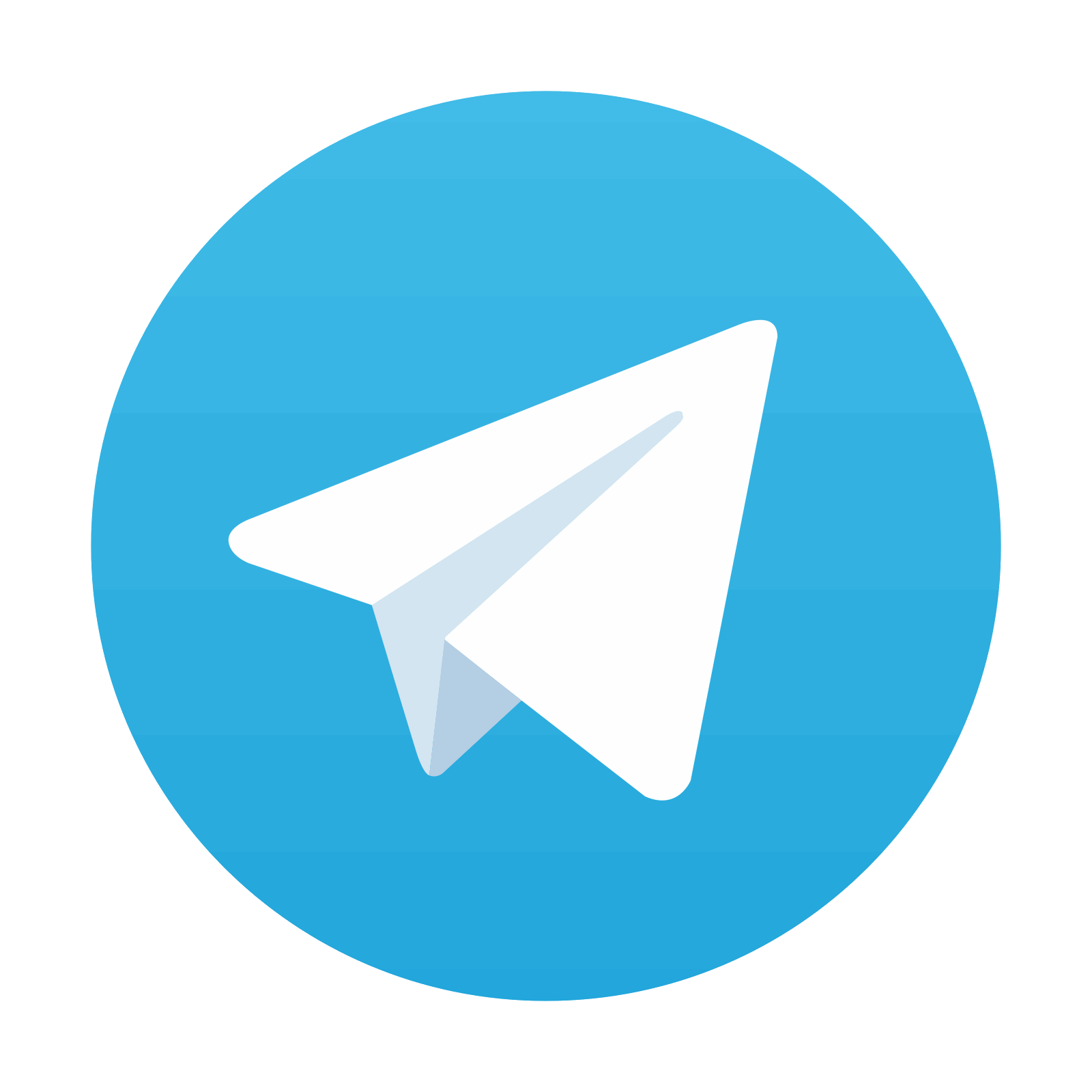
Stay updated, free articles. Join our Telegram channel

Full access? Get Clinical Tree
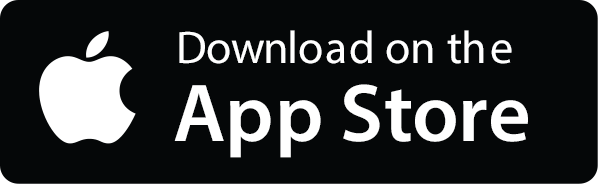
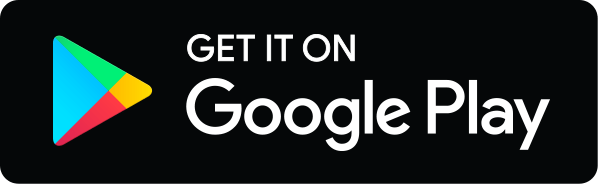