Abstract
Monitoring of the central nervous system using evoked responses allows assessment of neural tracts that are normally silent during coma or anesthesia. This chapter will discuss the techniques of the auditory brainstem response (ABR), somatosensory evoked potential (SSEP), motor evoked potential (MEP), electromyography (EMG), and a few related techniques. These have been applied widely in a large number of surgical and neuroradiological procedures involving head, spine, neck, and vascular procedures among others and are a standard of care in selected procedures. The implications for anesthesia management will be mentioned, as the anesthesiologist plays a key role in facilitating the monitoring.
Keywords
Auditory Brainstem Response, Electromyography, Intraoperative electrophysiological monitoring, Motor evoked potentials, Somatosensory evoked potentials
Contents
Introduction 148
Basic Principles 148
General Overview of Monitoring 148
Auditory Brainstem Response 149
Monitoring Other Cranial Nerves 150
Somatosensory Evoked Potentials 150
Motor Evoked Potentials 152
Electromyography 154
Other Specialized EMG Responses 157
Understanding the Equipment 157
Indications and Contraindications 158
Monitoring and Mapping for Brain Tumors 158
Monitoring for Cerebral Vascular Lesions 159
Brainstem Procedures 160
Monitoring Spinal Corrective Surgery 161
Intramedullary Spinal Tumors 163
Monitoring the Lumbosacral Spine 163
Thoracic Aorta Aneurysm Surgery 164
Contraindication for Monitoring 164
Effect of Anesthetics 165
Effects of Inhalation Agents 167
Effects of Intravenous Agents 167
Effects of Adjunct Medications 168
Readings and Interpretation 168
Advantages and Disadvantages 170
Current Evidence 171
Conclusion 171
Suggested Readings 171
References 172
Introduction
Monitoring of the central nervous system using evoked responses allows assessment of neural tracts that are normally silent during coma or anesthesia. This differs from the electroencephalogram (EEG), which monitors spontaneous activity. They are not a replacement for awake testing such as performed during awake craniotomy or under local anesthesia, but they allow examination during general anesthesia. This chapter will discuss the techniques of the auditory brainstem response (ABR), somatosensory evoked potential (SSEP), motor evoked potential (MEP), electromyography (EMG), and a few related techniques. These have been applied widely in a large number of surgical and neuroradiological procedures and are a standard of care in selected procedures. The implications for anesthesia management will be mentioned as the anesthesiologist plays a key role in facilitating the monitoring.
Basic Principles
General Overview of Monitoring
Using these techniques, a stimulus is applied to the neural tract, and a response is “evoked” that indicates the tract is functioning. This allows probing tissue to locate or “map” the tract, and it allows repeated testing of a tract during procedures to identify conditions of impending neural injury (“monitoring”). This allows improved decision-making during procedures and facilitates improved outcome. It also allows an assessment of the physiological environment of the neural tissue (e.g., perfusion pressure) to facilitate improved outcome.
In general, evoked responses are measured along the neural tract after the stimulus is applied. The response consists of series of peaks, each of which is produced by the neural tissue in the tract. For mapping, the presence of the response indicates that the tract has been located in the vicinity of the stimulator. For monitoring, repeated measurements are taken to watch for significant peak changes. Changes are usually noted from peak measurements such as the time from stimulation to the peak (referred to as “latency”) and the “amplitude” of the peak as measured to an adjacent peak of opposite polarity. Frequently, several monitoring techniques are utilized during procedures recognizing risk in multiple areas (“multimodality monitoring”).
Auditory Brainstem Response
The ABR is produced when the cochlea is stimulated using sound delivered into the external ear canal. It has also been referred to as the brainstem auditory evoked response (BAER) and the brainstem auditory evoked potential. Traditionally, broad-spectrum “clicks” are used with white noise (like a television tuned to an empty channel) delivered to the opposite ear to prevent the other cochlea from being activated by sound vibrations transmitted through the skull.
The response of the eighth cranial nerve can be measured using electrodes placed during surgery on the nerve or near the cochlear nucleus; however, it is usually monitored using electrodes at the external ear and top of head. The response consists of a series of five peaks produced within the 10 ms following stimulation. The latency of peaks I, III, and V and the amplitude of peak V are usually monitored. Wave I is produced by the extracranial portion of CN VIII, wave III by acoustic relay nuclei and tracts deep in the midline of the lower pons, and wave V by the lateral lemniscus and inferior colliculus contralateral to the side of stimulation ( Fig. 6.1 ). The response of the auditory cortex is referred to as the midlatency auditory evoked potential (MLAEP).

Experience has shown that increases in latency of 10% or more of peaks (or between peaks, interwave latency), decrease in peak amplitude of more than 50%, or loss of peaks is associated with significant dysfunction in the pathway, which correlate with changes in useful hearing. Hence the ABR is used to reduce the risk of hearing loss in surgery in the cerebellopontine angle of the brainstem. It has also been used as a general monitor of brainstem viability during surgery on the midbrain and pons. The MLAEP has been used as a measure of anesthetic effect but is rarely used for procedural monitoring.
Monitoring Other Cranial Nerves
The ABR is the only evoked sensory response testing routinely utilizing a sensory cranial nerve. Methods for testing the olfactory nerve (CN 1) have not been adequately developed for monitoring. Methods for monitoring the evoked response of the optic nerve (CN 2) (visual evoked potentials) have been developed using flash stimulation to closed eyes; however, routine use has not found utility similar to ABR. Finally, methods for evoked response testing of the trigeminal nerve (CN 5) have been developed and are occasionally used.
Somatosensory Evoked Potentials
The somatosensory evoked potential (SSEP) is one of the most commonly monitored evoked responses. The response is produced by applying an electrical stimulus to a peripheral nerve (much like that used for assessing train of four for neuromuscular blockade). The nerves (and their component nerve roots) usually utilized for monitoring are major motor and sensory nerves to the hands and feet (median n. (C6-T1), ulnar n. (C8-T1), and posterior tibial n. (L4-S2)). Stimulation activates large-diameter, fast-conducting Ia muscle afferent fibers producing a muscle movement and group II cutaneous nerve sensory fibers that produce cephalad traveling SSEP signals.
The major sensory response travels through the component roots of the nerves in the brachial and lumbar plexus ( Fig. 6.2 ). Entering the spinal cord via the dorsal root, the response ascends the spinal cord via the dorsal columns in the pathway of joint proprioception and vibration. A first synapse occurs near the nucleus cuneatus and nucleus gracilis, and it ascends the brainstem in the medial lemniscus after crossing the midline. After a second synapse in the ventroposterolateral nucleus of the thalamus, it travels to the primary somatosensory cortex contralateral to the side of stimulation.

For mapping purposes, stimulation of the posterior surface of the spinal cord can be used to locate the midline, and recording on the surface of the brain can be used to locate the gyrus between the sensory and motor cortex. For procedure monitoring, the response can be monitored along the peripheral nerve (verifying stimulation similar to seeing the motor response), over the brachial plexus (Erb point), epidurally near the spinal cord, and, most commonly, over the cervical spine (referred to as the subcortical response) and over the sensory cortex.
Monitoring has been used for surgery on peripheral nerves and plexus, monitoring for unfavorable positioning of the arm (brachial plexus), spine procedures, general brainstem viability, and procedures placing the subcortical and cortical pathways at risk. Experience indicates that more than 50% decrease in amplitude of response peaks or increase in latency of 10% or more are associated with concerns of unfavorable neural environment.
Motor Evoked Potentials
MEP are the most recent addition to the techniques used for monitoring. Of the various methods that have been used to stimulate the motor pathways, electrical stimulation of the motor cortex has emerged as the most commonly used technique that unquestionably allows motor tract assessment. The pyramidal cells of the motor cortex can be stimulated directly through a craniotomy (direct cortical stimulation); however the most commonly employed technique utilizes multipulse electrical stimuli applied via scalp electrodes (transcranial motor evoked potentials, TcMEP). Enhanced stimulation techniques have also been developed using a priming stimulus prior to the main stimulus to assist in young children, adults with neural pathology, and with partial neuromuscular blockade. Transcranial stimulation utilizing a brief magnetic stimulation has largely been abandoned due to depression by anesthesia.
This technique activates 4%–5% of the fast-conducting fibers of the cortico-spinal tract (CST), which descends the brain, brainstem (crossing in the brainstem), and spinal cord until it synapses on anterior horn cells ( Fig. 6.3 ). The descending response in the spinal cord consists of a D wave from the direct stimulation of the motor cortex and is considered an approximation of the volume of motor pathway activated. Its amplitude varies less than 10%. I waves accompany the D wave and represent the contribution of the response from transsynaptic activation of internuncial pathways in the motor cortex. I waves are sensitive to anesthesia.

Temporal summation of the D wave and I waves activates the anterior horn cells to produce a response in motor fibers of lower motor neurons. This response travels to the neuromuscular junction and produces a compound muscle action potential (CMAP) in about 4%–5% of muscle fibers. Not all of the descending spinal fibers directly innervate anterior horn cells; many have intervening synapses. In addition, several other motor pathways are either activated or influence the sensitivity of the anterior horn cell to activation. These additional synapses and other pathways give rise to wide variability of the CMAP produced under anesthesia. Of note, individual muscles are often innervated by muscle fibers from more than one nerve root.
MEP stimulation techniques can be utilized for mapping of the location of the motor cortex, and for the location of the CST in the subcortical tissue, brainstem, and spinal cord. The MEP response can be monitored utilizing an epidural electrode (monitoring the D wave) or using electrodes placed in peripheral muscles (monitoring the CMAP). The most commonly monitored muscles include those of the distal upper (e.g., abductor or flexor pollicis brevis) and lower extremity (e.g., abductor hallucis brevis and tibialis anterior), although muscles related to specific spinal cord regions can be used. Since the MEP has good correlation with motor outcome, it has been used to monitor motor cortex and general brainstem viability and procedures on the spine and spinal cord. It is frequently monitored along with SSEP, where it is thought to be more sensitive than the SSEP to ischemia in areas of the cortex, brainstem, and spinal cord.
Because of the stability of the D wave, a 50% reduction in amplitude has been used as criteria for concern. However, the wide variability of the CMAP response has created controversy about warning criteria. Suggestions have included changes in the configuration of the multipeaked CMAP response (indicating changes in the motor units responding), the need for increased cortical stimulation voltage (50–100 V) or current, and very substantial declines in the CMAP amplitude (i.e., >70%). One of the most commonly utilized MEP criteria is simply the loss of a CMAP response.
Electromyography
Electromyography (EMG) is the recording of activity using needle pairs placed in muscles. This differs from MEP in that the muscle responses are spontaneous or evoked by stimulation of cranial or peripheral nerves rather than central motor stimulation of the cerebral cortex. Two basic types of recording are used. Electrical stimulation of nerves leading to a muscle response is referred to as triggered EMG and is used to map the location or assess the continuity of nerve structures. The second type of recording is referred to as spontaneous or “free-run” EMG. In this case, muscle activity is seen in a usually silent background caused by other forms of stimulation such as mechanical or thermal irritations. Some of these stimuli are usually innocuous (e.g., inadvertent mechanical irritation); however, some are potentially injurious and signal the need to reassess the procedure (e.g., nerve stretching). In addition to seeing the response on the recording device, the response may be played on a loudspeaker.
The nerves innervating the muscles involved in EMG recording include cranial and peripheral nerves. For cranial nerves, any nerve with a motor component can potentially be monitored using the muscles innervated by the nerve ( Table 6.1 ). Mapping techniques have been developed to locate cranial nerve nuclei and the cranial nerve as it traverses the brainstem to the muscles recorded. Mapping of cranial nerve nuclei on the brainstem surface allows surgeons to approach deeper structures through designated safe entry zones.
Cranial | Nerve | Muscles used for monitoring and mapping |
---|---|---|
III | Oculomotor | Superior, medial, inferior rectus |
IV | Trochlear | Superior oblique |
V | Trigeminal | Masseter |
VI | Abducens | Lateral rectus |
VII | Facial | Orbicularis oculi, oris, mentalis |
IX | Glossopharyngeal | Stylopharyngeus muscle (posterior soft palate) |
X | Vagus | Vocal folds |
XI | Spinal accessory | Trapezius, sternocleidomastoid |
XII | Hypoglossal | Tongue |
Monitoring usually involves repeated intermittent stimulation in the operative field. Techniques which are not dependent on the surgeon providing the stimulation have been developed that are similar to MEP where transcranial stimuli evoke the muscle activity, thereby allowing monitoring independent of surgical stimulation (corticobulbar techniques). Such techniques have been described for facial nerve and vagus/recurrent laryngeal nerve monitoring. This latter technique also allows assessment of the cranial nerve function proximal to the surgical site.
For peripheral nerves a large number of muscles can be used depending on the specific nerve root desired ( Table 6.2 ). For these nerves, mapping can be utilized in injured peripheral nerves (neuroma in continuity) or to assess locations of injury in brachial plexus lesions. Mapping is also used to identify component rootlets of nerve roots to be sacrificed in dorsal rhizotomy for spasticity. Mapping is also used in surgery on the cauda equina (e.g., release of tethered cord) to identify functional nerve tissue to be preserved and nonfunctional tissue for sacrifice. Finally, as discussed subsequently, pedicle screw testing is a form of mapping the proximity of screws to nerve roots.
Spinal cord nerve(s) | Muscle(s) | |
---|---|---|
Cervical | C2-4 | Trapezoids, sternocleidomastoid |
C5-6 | Biceps, deltoid | |
C6-7 | Flexor carpi radialis | |
Thoracic | C8-T 1 | Adductor pollicis brevis, abductor digitiminimi |
T2-6 | Specific intercostals | |
T5-T12 | Specific areas of rectus abdominis | |
Lumbar | L2 | Adductor longus |
L2-4 | Vastus medialis | |
Lumbosacral | L4-S1 | Tibialis anterior |
L5-S1 | Peroneus longus | |
Sacral | S1-2 | Gastrocnemius |
S2-4 | Anal sphincter |
Monitoring of peripheral nerves using EMG is frequently done to identify the injurious stimuli mentioned earlier during procedures where nerve roots, the cauda equina, or peripheral nerves are at risk. Of note, when a particular nerve root is at risk, monitoring using intermittent triggered EMG of that root is more frequently utilized than free-run EMG since muscles used with MEP are usually innervated by more than one nerve root.
Several forms of evoked response can be seen in EMG. Triggered responses are usually brief CMAP responses linked in time to the stimulus as mentioned before. Free-run EMG has two basic forms of response ( Fig. 6.4 ). High-frequency CMAP bursts can identify blunt mechanical trauma or irritation to motor nerves. Causes of irritation include mechanical stimulation (e.g., nearby dissection; ultrasonic aspiration or drilling), nerve retraction, thermal irritation (e.g., heating from irrigation, lasers, drilling, or electrocautery), and chemical or metabolic insults.

More continuous EMG activity is referred to as “trains”or “neurotonic discharges,” and may be associated with impending nerve injury (nerve compression, traction, or ischemia of the nerve). When played on a loudspeaker, they have musical qualities like the sound of an outboard motor boat engine, swarming bees, popping corn (“popcorn”), or an aircraft engine (“bomber”) potentials. These neurotonic discharge trains raise concern for nerve injury as does very high amplitude bust responses. Unfortunately, nerve injury can occur without EMG activity such as when the nerve is severed.
Other Specialized EMG Responses
A specialized type of triggered EMG response is reflex testing. In this case the stimulus is applied to a peripheral sensory nerve that sends a volley of activity to the spinal cord via the dorsal root. It triggers reflex pathways in the spinal cord that activates motor fibers in the ventral root and peripheral nerve resulting in a CMAP. Three CMAP responses can be seen. The first “M” response is from direct activation of motor fibers in the stimulated nerve (like used for train of four assessment). The second “Hoffmann” (H) response is the reflex response. The last is an “F” response that is produced by the reflection of the incoming sensory volley at the spinal cord that sends a motor response back to the muscle via the stimulated nerve. Of note, the H reflex pathway in the spinal cord is dependent on normal cephalad spinal cord function. As such, a spinal cord injury will depress the H reflex activity caudal to the injury, even when the reflex occurs in uninjured spinal cord. As such the H reflex has been used in spinal surgery as a measure of spinal integrity cephalad to the region of reflex.
Another commonly utilized reflex is the bulbocavernosus reflex. This reflex results from stimulation of the pudendal nerve at the genitalia, reflex activity in the S2–S4 spinal cord segments, and efferent activity also via the pudendal nerve resulting in a CMAP in the external anal sphincter. This has found utility for testing during cauda equina surgery to preserve neural innervation of the bowel and bladder, which travel in the pudendal nerve.
Understanding the Equipment
Equipment for conducting evoked response mapping and monitoring largely emerged in the 1960s and 1970s when digital computers became widely available. As such, the current equipment usually consists of a digital computer connected to a set of stimulating devices, a set of recording amplifiers, a display, and a storage device. The technology is sufficiently complex that a dedicated professional is needed to conduct the technological component of the mapping and monitoring. In addition, the equipment usually has the capability of HIPPA-compliant real-time data transfer to a remote computer for shadow observation and consultation by another monitoring professional. Since the equipment is electrically connected to the patient, methods to prevent electrical risk to the patient are employed, and the equipment is routinely checked for leakage current similar to other equipment in the procedure room.
Stimulation, Wiring, and Connections
As before, each type of evoked response testing requires specialized stimulation devices. The specifics of each stimulator device applied to the patient and the electrical characteristics of the stimulation are designed for the specific response being tested.
Recording, Electrical Contaminations, and Storage
The response is recorded from electrodes attached to the patient and amplified prior to being processed by the digital computer. Since the responses are very small compared to other physiological signals (e.g., the electrocardiogram and EEG), a preamplifier is usually placed near the patient, and other efforts are made to reduce the interference of other signals. The processing of the signal usually involves filters to remove frequencies of electrical activity not characteristic for the response being measured and conversion to digital data for processing and storage. The conversion to digital information is particularly important for signal averaging required for the ABR and SSEP. For these responses, their very small size requires that many signals be averaged by adding the responses time locked to the stimulus. The need for this signal averaging is why the routine application of SSEP testing awaited the availability of digital computers. For responses, such as muscle CMAP, which are very large compared to the background noise, signal processing is not required, and the response can be displayed similar to the EEG.
Indications and Contraindications
Monitoring and Mapping for Brain Tumors
Mapping and monitoring have found utility in removing brain tumors and cavernous angiomas near the motor cortex, motor tracts, and insula. Mapping with the SSEP and MEP has been used to locate the sensory and motor cortex, so undesired motor injury is avoided when the presence of a tumor distorts the usual surface anatomy. Direct cortical stimulation has been used to map the edge of the tumor as it interfaces with the motor cortex. Similarly, monitoring, especially using the MEP, has been used to warn of encroachment on motor tracts during resection. Mapping and monitoring has been shown to improve outcome and allow more extensive tumor resection in both children and adults for all supratentorial tumors.
Monitoring for Cerebral Vascular Lesions
Intracranial Vascular Surgery
Since monitoring is sensitive to ischemia, monitoring can signal unfavorable reductions in blood flow during vascular surgery. For example, during carotid endarterectomy (CEA) the SSEP can be used complementary to the EEG since the SSEP will be sensitive to ischemia in the subcortical pathways not monitored by the EEG.
The SSEP and MEP have also found utility during surgery of intracranial aneurysms and arteriovenous malformations (AVM) where outcome has correlated with monitoring changes. With aneurysms, changes have been seen with inadvertent vessel occlusion, temporary clipping, vasospasm, retractor pressure, suboptimal clip application, and relative hypotension. The SSEP has found substantial utility to assess ischemia downstream from intracranial aneurysm surgery and improve outcome.
A growing appreciation of the value of MEP has been gained by the realization that perforating arteries (especially with middle and anterior cerebral artery aneurysms) can be inadvertently occluded by clips, which do not produce SSEP change, but rather produce ischemia in the motor tracts that are detected by the MEP. Of note the stimulation intensity of the MEP is critical in this monitoring to insure the motor tracts are only stimulated cephalad to the area of potential ischemia. In addition to complementing the SSEP by monitoring different tissue, the MEP appears to be more sensitive to ischemia than the SSEP, suggesting their use should be considered in all intracranial vascular surgery.
Interventional Vascular Procedures
Evoked response monitoring has found utility in interventional procedures for the detection of ischemia. As with the intracranial procedures, SSEP has been widely utilized. MEP is similarly valuable; however, its use has been somewhat reduced due to concerns about movement interfering with radiographic imaging (masking). As with intracranial procedures, MEP can be used with attention to detail to reduce movement.
Differing from intracranial procedures, monitoring during interventional procedures may also detect ischemia due to the intravascular nature of the procedure. Hence, they include placement of vascular stents (e.g., carotid, cerebral arteries, thoracic aorta), aneurysm coiling, embolization of AVMs (intracranial and spinal cord), and balloon angioplasty (e.g., post subarachnoid hemorrhage vasospasm).
With AVMs, monitoring has found utility for assessment of the impact of arterial flow prior to permanent occlusion. Also, occlusion can be used to assess feeding arteries of AVMs. Alternatively, for AVMs, provocative testing using synaptic blockade with a barbiturate (e.g., sodium amytal) or white matter conduction blockade using lidocaine can be done to assess if the artery is critical to the monitored pathway. Similarly, an assessment can be made of blood vessels planned to be embolized to reduce the vascularity of tumors prior to resection.
Brainstem Procedures
For procedures in the brainstem, EMG mapping and monitoring of cranial nerves are frequently used. The cranial nerves are susceptible to damage due to their small size, limited epineurium, and that they may be intertwined or obscured by the pathology to be resected. Mapping techniques are of assistance in defining the safe entry zone for approaching deeper tumors. One of the most frequently monitored cranial nerves is the facial nerve. Its monitoring has allowed significant improvement in outcome, so an NIH consensus panel recommended it be used with vestibular schwannoma (acoustic neuroma). It is thought that monitoring assists to maintain the structural integrity of the facial nerve, which increases the chance of functional recovery.
Monitoring of other cranial nerves has been used in surgery on the base of the skull, cavernous sinus, and posterior fossa with the specific nerves monitored based on the risks and brainstem region of the procedure. One unique monitoring technique is called the lateral spread response and is used during microvascular decompression. This technique involves stimulation of one branch of the facial nerve and monitoring for a response in other branches of the nerve, which is normally not seen except with pathology. In patients with hemifacial spasm, this anomalous response will be seen, which disappears after successful decompression.
ABR has been utilized to attempt a preservation of hearing with tumors where the cochlear nerve is involved. In general, if waves I and V are preserved during surgery, hearing is usually preserved. ABR has also been utilized as a monitor to assess the general viability of the brainstem when mechanical or vascular compromise may occur. The ABR is more sensitive than heart rate or blood pressure for detecting brainstem injury.
In general, the use of multiple modalities is usually used with brainstem surgery and has allowed procedures that previously had been considered unresectable. The SSEP and MEP have also been used like the ABR to assess general brainstem viability. It is thought that the combination of ABR with SSEP assesses about 20% of the brainstem. The MEP is thought to be particularly important with surgery close to the cerebral peduncles or in the ventral medulla, and mapping to locate the CST has been described in this region.
In addition to the NIH consensus panel noted for facial nerve monitoring, several other studies have shown a correlation of monitoring with outcome in brainstem surgery. For example, the amount of abnormal EMG activity correlates with outcome after tumor surgery.
Monitoring Spinal Corrective Surgery
One of the early applications of evoked response monitoring was the use of SSEP during Harrington rod correction of scoliosis. In these early cases, distraction by the rod produced a mechanical stress on the spinal cord that affected the posterior column function (SSEP pathway) and anterior spinal cord (motor pathways). As such, SSEP monitoring changes reflected motor outcome. An assessment by the Scoliosis Research Society and European Spinal Deformities Society demonstrated a reduction in the rate of paralysis to 0.55% from 0.7% to 4%. A position statement made monitoring a standard of care. Of note, since the SSEP and MEP are conducted in different anatomic and vascular regions of the spinal cord, paralysis was not completely eliminated, leading to interest in monitoring the motor tracts.
Since that over time, spinal column surgeries and instrumentation have become more complex with multiple potential injurious components (e.g., distraction or compression of the spinal cord from correction by instrumentation such as hooks, wires, and pedicle screws, direct spinal cord trauma, epidural hematoma, and derotation, and ischemia from tension on blood vessels, relative hypotension, anemia, and ligation of anterior segmental vessels). Since many of these factors could differentially effect the motor tracts, the addition of MEP to the SSEP has become routine. Further, these multitude of potential insults have stressed the value of continuous monitoring assessment making the once used wake-up test less valuable because the latter is a one-time assessment. Since many of these factors are reversible (such as ischemia), the ability to detect neural compromise and correct it has led to excellent correlation with and improved outcome.
The success with scoliosis has also led to interest in monitoring with a large variety of spinal column surgeries and a wide variety of pathologies. The combination of SSEP, EMG, and MEP has been shown to be extremely effective in detecting spinal compromise and improving outcome. A recent evidence-based review by the American Academy of Neurology and American Clinical Neurophysiology Society found sufficient evidence to publish a guideline supportive of multimodality monitoring in spinal surgery.
An important addition to SSEP and MEP has been EMG because of the risk to nerve roots from instrumentation such as pedicle screws (injury in 15%–25% of cases). The EMG is important because it allows assessment of nerve roots not included in the SSEP or MEP and can allow assessment of an individual nerve root where the SSEP and MEP cannot. For pedicle screws, medial misalignment can breach the pedicle wall and place the screw threads adjacent to the nerve roots causing injury and/or postoperative pain. This latter situation has been detected by stimulating the pilot hole or screw and determining the proximity of the screw to the nerve roots by measuring the current necessary to trigger a CMAP response in the muscle innervated by nerve root (threshold). If this current is low, then misalignment has likely occurred, and realignment should be considered. Numerous studies show good utility for detecting misalignment, notably in screws placed in the lumbar spine. Challenges with this technique include poor bone density–lowering thresholds and increases in threshold due to newer screws, which are poor electrical conductors, chronically compressed nerve roots, or pathology in the nerve roots.
Another challenge with threshold testing is assessing screw placement in the thoracic spine where fewer muscles are available for recording. Since the spinal cord is close to the pedicle, an alternative approach has been to assess the threshold to stimulation of the CST using CMAP responses in lower extremity muscles.
During cervical spine surgery, MEP monitoring is desirable because it is believed to improve outcome by differentiating cervical cord myelopathy from peripheral neuropathy. EMG is also used to detect problems with lateral mass screw placement and the common occurrence of C5 radiculopathy likely due to its shorter length and obtuse angle of exit from the spine. Spontaneous EMG is also used during anterior cervical spinal surgery to assess the vagal and recurrent laryngeal nerves that are at risk due to the retractor used to expose the spine. This is typically recorded continuously using electrodes on the surface of the endotracheal tube, which are placed to oppose the vocal cords and record EMG from the vocalis muscle. This technique is similar to that used with neck dissections and thyroid and parathyroid removal, except these other procedures typically use a handheld stimulator to map the location of the nerve and periodically verify its integrity.
Intramedullary Spinal Tumors
Intramedullary spinal cord tumors are usually benign tumors within the spinal cord that produce damage as they grow. In particular, there are substantial risks to the motor tracts as the surgeon attempts to resect the tumor. Prior to evoked response monitoring the risks of motor injury were substantial and limited the resection. The SSEP is used to map the midline since the left and right dorsal column responses straddle the midline. This minimizes the damage on entering the spinal cord but frequently causes a loss of the SSEP precluding its use for further monitoring. The CST can be mapped within the spinal cord using a collision technique where stimulation in the spinal cord blocks the CMAP response from transcranial stimulation.
Monitoring during tumor resection usually focuses on the MEP D wave recorded from epidural electrodes and CMAP responses from muscles innervated by nerve roots caudal to the surgical site to warn when the resection is encroaching into the motor tracts. Since the D wave is a semiquantitative measure of stimulated CST fibers, a loss of 50% amplitude of the D wave has been found to be an adequate warning that further resection will risk loss of motor function. If the CMAP response is lost, then temporary loss of motor function occurs, but function returns long-term if the D wave changes less than 50%. Studies show an excellent correlation of monitoring with and improvement in motor outcome.
Monitoring the Lumbosacral Spine
For spine surgery below the end of the spinal cord (usually about L1), surgery places the cauda equina and nerve roots at risk. As such, monitoring using the EMG has been useful. Because of the multiroot nature of the SSEP and MEP mentioned earlier, they are less specific for risk assessment of individual nerve roots. Nevertheless, studies have demonstrated their utility in improving outcome. EMG is also considered useful to assess peripheral nerves that may be injured due to limited vision with minimally invasive techniques or injured by techniques that traverse the lumbar plexus during the extreme lateral approach to the spine.
For procedures involving the cauda equina (e.g., tethered cord or tumors of the cauda equina) mapping and monitoring shifts to identifying and retaining functional tissue (especially the innervation of the lower extremity, bowel, bladder, and sexual function). For this, EMG is utilized, including monitoring the anal musculature and the bulbocavernosus reflex. Bladder pressure manometry has also been used to assess detrusor muscle stimulation. These techniques have shown an improvement in outcome.
Thoracic Aorta Aneurysm Surgery
Monitoring has also been utilized during the repair of thoracic aortic aneurysms where the risks of paralysis are extremely high due to the risks to the blood supply of the spinal cord. The SSEP and MEP can be used for monitoring to detect ischemia in the white or grey matter tracts of the spinal cord. Both MEP and SSEP can be used during an occlusion test to evaluate the importance of intercostal and other perforators. The MEP is more sensitive due to the greater sensitivity of the grey matter, and it has a better correlation with motor outcome.
Contraindication for Monitoring
In general, there are very few contraindications for these monitoring techniques, but complications do occur. In essence, the evaluation of risk and benefit needs to be applied with each patient to weigh the risks of the technique versus the potential benefit for surgical decision-making and patient outcome. As mentioned earlier, all of these techniques utilize electrical connections to the patient, but properly maintained equipment is associated with a rare risk of electrical burn. Also, many of these techniques use needle electrodes for stimulation or recording; the risks of needle injury may be more to providers than the patient.
The technique with the most likely complication is the MEP. A variety of potential complications have been described (e.g., electrical injury from stimulation); however, in practice the actual incidence is small, dominated by bite injuries to the tongue (prevented by the use of soft bite blocks between the molars). Contraindications usually mentioned often include electrical wires or devices implanted in the brain or defects in the skull that might change the usual flow of stimulation current. Others such as the presence of a seizure disorder do not appear to be a contraindication. This is an area that is still evolving with many possible contraindications being found not to be a reason to withhold monitoring when it is beneficial. Finally, some raise concern for patient movement; however, proper choice of stimulation parameters, anesthesia, and timing with the procedure will minimize unacceptable movement.
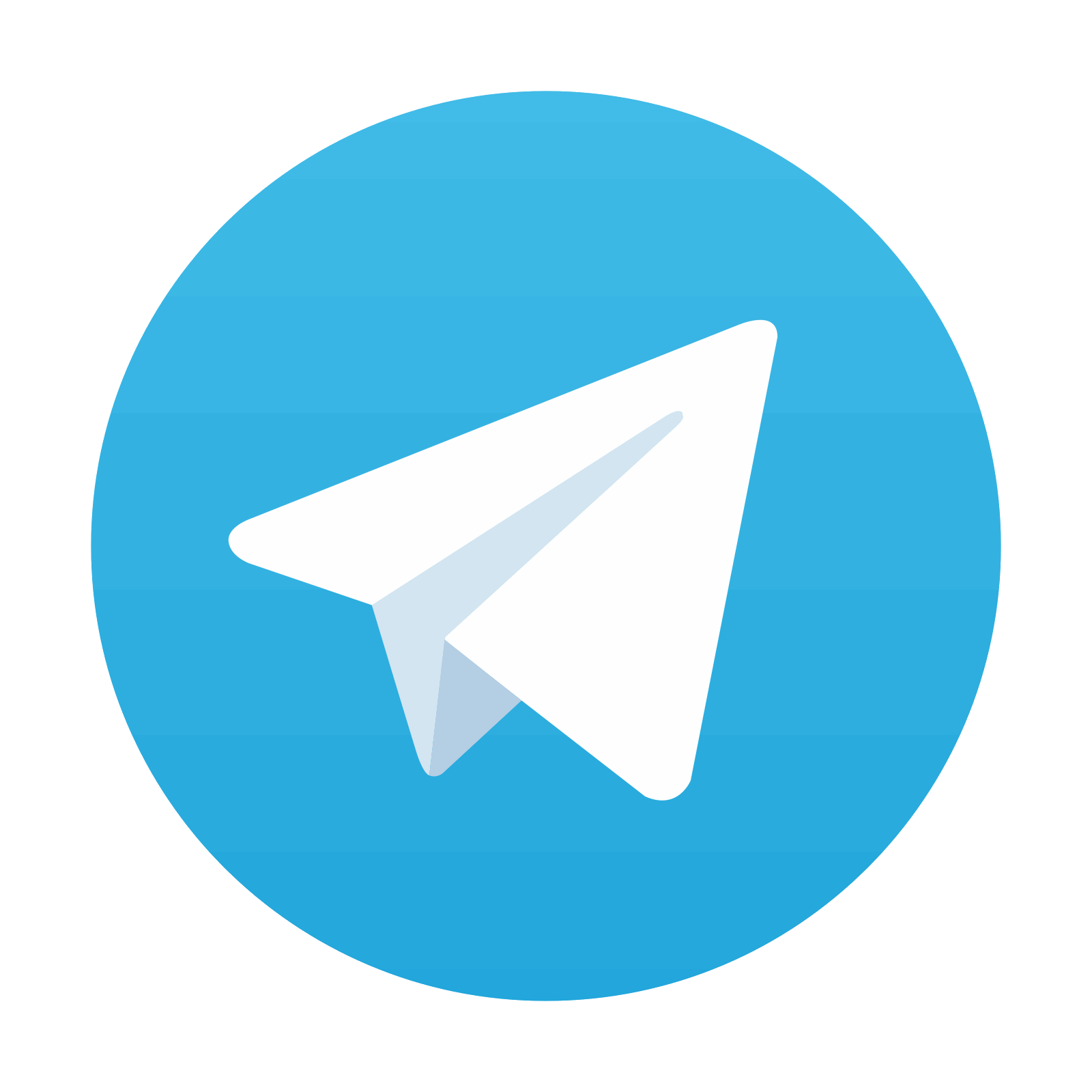
Stay updated, free articles. Join our Telegram channel

Full access? Get Clinical Tree
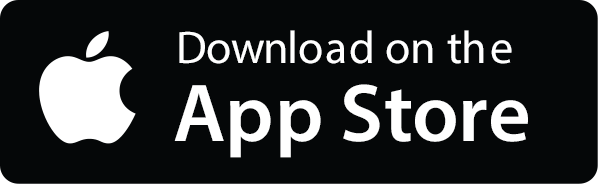
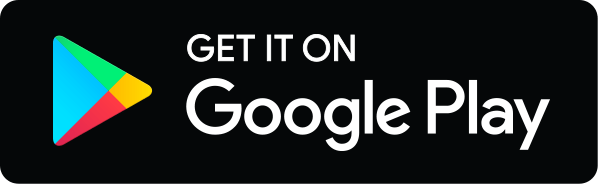