Fig. 6.1
Scheme illustrating the nature of fluorescence and how it is visualized
A Brief History
Historically, the use of a fluorophore for highlighting malignant glioma tissue was first described by Moore et al. [10] as a means of localizing brain tumors. Equipped only with topographical information from neurological examinations and vague morphological–anatomical data from ventriculographic studies, and intra-operatively with a brain needle for finding tumor-afflicted brain and biopsies of tissue, these authors employed a mercury vapor lamp and a Wood’s filter together with intravenous fluorescein to locate and resect tumors. They noted that in some cases, they were able to identify additional small regions of tumor, which escaped notice when viewed with ordinary illumination, thus first defining the two key elements of the use of fluorescence for surgery of brain tumors:
initial detection of the tumor in the brain
optimizing resection based on tissue fluorescence
Regarding fluorescein, they acknowledged, however, that “the brain tissue immediately surrounding the tumor is edematous and edematous brain tissue is shown to retain a greater amount of dye than otherwise normal brain” and “edematous tissue surrounding the tumor does fluoresce, but to a lesser degree…” thus already pointing out possible limitations of dyes which reach the brain tumor via a breached blood–brain barrier in the tumor, i.e., of passive permeability markers. At that point of time, the concept of a blood–brain barrier was long accepted, but the lack of such in tumor was still in the realm of theory and put forward by Moore et al. [10] as a possible explanation for the differential uptake of dye when comparing tumor, edematous tissue, and normal brain. In summary however, the authors felt fluorescence to have “definitive clinical value.” On a by note, this paper already envisages a coupling of 131-iodine to fluorescein dyes for better diagnosis of malignant brain tumors.
After this first report little was heard about fluorescence in neurosurgery until the late 1990s when Kuoriwa and co-workers from Japan presented three publications on fluorescein for glioma surgery the last in 1999 [11–13].
In 1998, we published our first reports [14–16] on 5-aminolevulinic acid (5-ALA) for fluorescence-guided resections providing data on both experimental data and data on the first use of this compound in humans in a small phase I/II patient cohort. In contradistinction to fluorescein, 5-ALA is a prodrug, which is converted into a fluorescing fluorophore within tumor cells [17, 18] and thus is unique so far, compared to other fluorophores that have been investigated. 5-ALA has been studied extensively for fluorescence-guided resections since then.
Recently, with new filters integrated into operating microscopes, fluorescein is again receiving some, albeit controversial, interest [19–22].
Both fluorochromes will be discussed in detail in the course of this paper. Currently, new approaches with fluorophores targeted to surface markers of tumors cells are being investigated and are on the verge of clinical translation.
ALA for Fluorescence-Guided Resections
5-Aminolevulinic acid (ALA) is a natural metabolite, which is involved in heme-biosynthesis. When given in excess, this compound results in the accumulation and/or selective retention of fluorescing porphyrins, particularly protoporphyrin IX (PPIX) in epithelia and more predominantly in cancers arising from such epithelia and in many brain tumors [17, 18, 23]. Under physiological circumstances, PPIX is converted into non-fluorescent proto-heme by iron chelation and from there into hemoglobin. In experiments utilizing the C6 glioma cell line in vitro and in vitro we were able to first demonstrate selective retention in malignant glioma cells [15], an observation, which was safely extended to human malignant gliomas [16]. The reasons for this selectivity are not completely understood. Relationships have been postulated to decreased expression of ferrochelatase in tumors [24], an enzyme which converts PPIX to proto-heme by chelating iron into the porphyrin ring. Other possible explanations have been put forward, such as a decreased activity of the ATP-binding cassette transporter (ABCB6) [25] which eliminates porphyrins from cells, as well as tumor cell density, tumor cell proliferative activity and a disturbed blood–brain barrier permeability in the region of the tumor [26, 27].
PPIX is strongly fluorescent with an excitation maximum at approximately 405 nm (the so-called Soret band) with two emission peaks at 635 and 704 nm (Fig. 6.2) [28]. In vivo, the spectrum detected from tissue has an additional peak at between 450 and 500 nm, i.e., in the green range, resulting from underlying brain tissue autofluorescence from NADH, riboflavins and carotenoids and other autofluorophores in normal tissue. This autofluorescence is equally stimulated by blue excitation light [29]. Surgical microscopes adapted for protoporphyrin IX detection currently use xenon excitation light filtered for violet-blue with a wavelength peak at 375–440 nm. For observing fluorescence they do not simply use a long-pass or barrier filter for visualizing the 635 nm peak of protoporphyrin IX, that is, cutting away all wavelengths below for instance 600 nm. This would result in PPIX being visible on a black background, without allowing any background discrimination. We considered the possibility of background discrimination to be important for enabling resection and tissue manipulation by the surgeon using the fluorescence mode. Rather, microscopes by the major manufactures use barrier filters for fluorescence detection, which open at slightly below 440 nm. This allows a small fraction of the excitation light to be included in the observation pathway of the microscopes. In addition, tissue autofluorescence, which is located in the blue-green range, is also visualized. Remitted excitation light and tissue autofluorescence together enable background brain tissue to become visible with a green-blue tone [14].


Fig. 6.2
Fluorescence excitation and emission in tissues containing PPIX, compared to the visible light spectrum. PPIX has an absorption maximum at 405 nm, the so-called Soret band, which is located in the blue range. Excitation light sources typically used for microscopes feature a wavelength range of 375 to about 440 nm. PPIX emission peaks are at 635 and 705 nm, both in the red range. Brain tissue additionally contains autofluorophores (carotenoids, riboflavin, NADH). In vivo, autofluorescence is superimposed over the pure PPIX signal. By choosing a barrier filter, which allows the passage of light of longer wavelengths than about 440 nm, a small part of the excitation light and autofluorescence together provide background “illumination” with superimposed red tumor fluorescence
5-ALA is currently approved for resections of malignant gliomas in many countries of the world after initial approval by the European Medicines Agency (EMA). Approval was granted based on a study [30], which randomized patients with malignant gliomas to be operated on either with or without 5-ALA for fluorescence-guided resections. Groups were compared for extent of resection and safety as well as for progression-free survival. Extents of resection were greater in the 5-ALA group, resulting in a prolongation of progression-free survival without significantly more frequent neurological deficits. Rates of complete resection of contrast-enhancing tumor were 65% for the 5-ALA versus 35% for the control arm with conventional white light illumination.
On a by note, the rate of 65% is frequently cited as the limit to what can be achieved using 5-ALA. This may not be the case however, since patients operated on using 5-ALA in the context of the study were the first dozen or so patients ever to be operated on by surgeons with relatively little experience with the novel technology. Newer studies put forward by experienced surgeons indicate a much higher potential of 5-ALA for facilitating gross total resections of enhancing tumors in up to 96% of cases (Table 6.1), when used in conjunction with neurophysiological mapping and monitoring [31–35]. In one recent study, intra-operative MRI in combination with neurophysiological methods resulted in 82% “complete” resections [35]. When augmented with 5-ALA-induced fluorescence, gross total tumor resection rates were stated to have been reached in 100%. Overall, a meta-analysis by Eljamel [36] indicated a rate of gross total resection, i.e., resection of more than 98% of enhancing tumor in over 75% of a total of 565 patients.
Table 6.1
Resection rates using 5-ALA as reported in the literature
Publication | n | Location | ioMR | Monitoring mapping | Study type | Resection rate (%) | Comment |
---|---|---|---|---|---|---|---|
Stummer et al. [37] | 50 | Eloquent and non-eloquent | No | No | Prospective monocentric cohort | 65 | |
Stummer et al. [30] | 135 | Eloquent and non-eloquent | No | No | Prospective multicentric two-arm randomized | 65 | |
De la Puppa et al. [34] | 25 | Eloquent only (motor, language) | No | Yes | Prospective monocentric cohort | 80 | |
Diez Valle et al. [33] | 36 | Eloquent and non-eloquent | No | Yes | Prospective monocentric cohort | 83.3 | |
Coburger et al. [35] | 33 | Eloquent and non-eloquent | Yes | Yes | Prospective Monocentric cohort, historical matched pair | 100 | ioMR alone 82% |
Schucht et al. [32] | 67 | Eloquent only (motor) | No | Yes | Prospective monocentric cohort | 76 | |
Schucht et al. [31] | 103 | Eloquent and non-eloquent | No | Yes | Prospective Monocentric cohort | 96 |
In retrospect, utilizing resection rates as a primary study aim for a randomized study may not have been a well-chosen endpoint for testing the usefulness of 5-ALA. Resection depends on many factors other than visualizing the tumor, foremost the worry about maintaining function, which depends strongly on the surgeon, the surgeon’s convictions regarding the value of resection and the use of mapping/monitoring for safely extending resections. Particularly, the concepts and views regarding these factors have changed over time. Today the value of safe resection is more generally accepted in the neurosurgical community and mapping/monitoring has become a common adjunct for surgery of malignant gliomas. Thus, it may have been more appropriate to test 5-ALA as an intra-operative diagnostic agent or contrast medium. This would have involved testing the compound for toxicological safety as well as for measures of diagnostic accuracy only, e.g., looking at the positive predictive value of fluorescence for demonstrating tumor rather than attempting to prove a therapeutic value of the use of 5-ALA. This potential therapeutic value is the sum of patient selection and intra-operative tumor detection but mostly of the surgeon performing the surgery and utilizing any available technology, the surgeon’s role being highly uncontrollable and variable.
5-ALA is presently being marketed as “Gliolan®” in the EU and other parts of the world, and surgical microscopes modified for fluorescence-guided resections are available from the leading microscope manufacturers, for which fluorescence modules can be purchased as accessories. These microscopes uniformly feature xenon light sources generating conventional white light and are switchable to blue light illumination and the appropriate observation filters, usually by operating a switch or foot pedal, thus enabling free shuttling between both illumination modes.
What Does Visible PPIX Fluorescence Signify in the Context of Glioma Surgery?
Selectivity
Relying on 5-ALA-induced PPIX for fluorescence-guided resection requires knowing how accurately fluorescence indicates bulk tumor or infiltrated brain. The authors have almost unanimously determined a high positive predictive value (PPV, the probability that fluorescence indicates tumor) of over 95% [33, 35–42]. The values for specificity and sensitivity are more varied in the literature, the reason being that both these traditional measures depend on tissue sampling in non–fluorescing, normally appearing tissue (Fig. 6.3, adapted from Stummer et al. [43]).


Fig. 6.3
Scheme for illustrating the calculation of measures of diagnostic accuracy, which are frequently used for determining the value of a diagnostic method. In studies on fluorescence-guided resection, “specificity” and “sensitivity” are highly variable when comparing the results of different investigators, since these measures require random biopsies from “normal” appearing brain. The distance to the main tumor mass at which these biopsies are obtained is crucial, because with increasing distance from the tumor cell density rapidly drops. This distance is rarely defined in various studies (adapted from [43])
Importantly, the results of such sampling strongly depend on the distance from the tumor the samples are collected because in malignant gliomas cell density rapidly decreases with distance from the tumor mass along with likelihood for finding tumor-infiltrated biopsies. Therefore, determining specificity and sensitivity depends critically on the distance at which samples from non-fluorescent marginal tissue are taken. Since authors of different studies employ different sampling algorithms, they will understandably determine different values for these traditional measures of diagnostic accuracy.
The PPV, on the other hand, is the only measure of diagnostic accuracy that does not require biopsies from non-fluorescing, normally appearing tissue (Fig. 6.3). The negative predictive value (NPV, the probability that non-fluorescing tissue shows tumor cells) similarly depends on the location in non-fluorescing tissue from which samples are taken. If investigators collect samples close to macroscopically fluorescing tissue, the NPV will be lower compared to the NPV determined by investigators who collect samples at a larger distance from macroscopically fluorescing tissue. The varying values calculated by different authors are reflected in Table 6.2.
Table 6.2
Accuracy of intra-operative fluorescence for predicting tumor
Publication | PPV | NPV | Sensitivity | Specificity |
---|---|---|---|---|
Stummer et al. [37] | 99 | 50 | 89 | 96 |
Coburger et al. [35] | 99 | 22 | 91 | 80 |
Yamada et al. [41] | 92 | 69 | 95 | 53 |
Diez Valle et al. [33] | 100 (strong) 97 (weak) | 66 | N.g. | N.g. |
Idoate et al. [38] | 100 (solid tumor) 97 (infiltrating tumor) | 67 | N.g. | N.g. |
Stummer et al. [42] | 96 | 40 | N.g. | N.g. |
According to our recent experience [42], fluorescence can be visualized using the microscope down to a cell density of 10% in malignant gliomas. Using more sensitive spectrometry, more infiltrating cells can be detected [42, 44], which are not visible through the microscope due the low intensity of fluorescence.
Although we were able to establish a relationship between WHO grade and fluorescence as well as the MIB (Ki-67) index and fluorescence in gliomas, we were unable to detect a relationship to other molecular markers, which are currently being determined on a routine basis for gliomas, e.g., 1p19q co-deletions, MGMT promoter methylation, or IDH1 mutations [45].
False Positive Fluorescence Induced by 5-ALA
False positive fluorescence, when described, was usually related to tissue areas in close proximity to the resection cavity [37, 39, 46, 47] or on a microscopic basis [48] but was never observed at a meaningful distance from the tumor cavity. Such fluorescence has been attributed to reactive astrocytes or inflammation, especially in patients with recurrent malignant gliomas, but not to normal brain tissue [46–49]. In 313 patients with imaging suggestive of recurrence of glioblastomas after multimodal therapy, 3% of patients showed fluorescence in tissue where pathological examination revealed radiation necrosis [49]. This fluorescence was described as weak. Fluorescence in normal brain was not described in that report.
Relationship Between 5-ALA-Induced Fluorescence and Enhancement on the MRI
Fluorescence has been related to contrast-enhancement of malignant gliomas on the MRI [39]. However, a number of investigators have provided data demonstrating fluorescence to extend beyond contrast-enhancement [35, 37, 42, 50–52] (Fig. 6.4). Resection of this area of fluorescence tissue, which extends beyond contrast-enhancing tumor, has been associated with extended survival [51]. A number of groups have provided evidence that fluorescence even in non-enhancing tumors can be predicted by amino acid positron emission tomography (PET) with 18F-fluoroethyl-tyrosine (FET) or 11C-methionine [45, 52–55].


Fig. 6.4
5-ALA-induced tumor fluorescence extends beyond enhancing tumor. This patient with a left angular gyrus glioblastoma was operated on first without fluorescence. Residual enhancing tumor is visible and the resection cavity is slightly smaller than the original tumor (yellow insert), due to decompression. Bottom row surgery was repeated using 5-ALA and mapping/monitoring. All fluorescing tissue was removed safely. The final resection cavity (red insert) extends considerably further than the enhancing tumor regions
In Which Tumors Is 5-ALA of Value?
Use in Gliomas Apart from Gbm
The use of 5-ALA for fluorescence-guided resections of brain tumors was first described for malignant gliomas [16], and the use in glioblastoma is the most common use described so far. Other gliomas may be amenable to fluorescence-guided resections as well, as is the case for approximately 16–20% of grade II gliomas [45, 53, 56, 57], despite the accepted lack of significant blood–brain perturbations in such tumors. In gliomas, which do not show typical imaging characteristics of glioblastoma, tumor size and patient age were predictive of finding useful fluorescence intra-operatively, either for fluorescence-guided resections or for finding an anaplastic focus [45]. In the case of gliomas without imaging features suggestive of glioblastoma (i.e., necrosis marginal enhancement and perifocal edema), any enhancement on the MRI resulted in the likelihood of useful fluorescence of 78%; if patients were older than 44 years and tumors were larger than 7 cm3, this likelihood was 97%. In non-enhancing tumors, tumor size and the FET-PET ratio were predictive of fluorescence. Patients with non-enhancing tumors and a FET-PET uptake ratio of at least 1.85 demonstrated a likelihood of 23% for showing fluorescence. If tumors were additionally larger than 11 cm3, this probability was increased to 46% [45].
Thus, in our practice all patients with non-enhancing tumors are subjected to FET-PET for assessing whether 5-ALA would be of value for surgery. FET-PET is also helpful for determining the location of anaplastic foci in otherwise apparently low-grade gliomas [53–55], which would be identified during surgery based on fluorescence and be specifically biopsied for targeted histological interrogation. Such hot spots, if macroscopically fluorescent, were found to indicate high-grade areas in otherwise low-grade tumors [54], thus avoiding undergrading of these tumors.
It is, however, important to understand that the fluorescence visible to the surgeon through the microscope is actually quite strong, and too strong to be absorbed by the microscope lenses and filters. Thus, the observation of fluorescence in 16–20% of LGG gliomas alludes to macroscopic fluorescence, i.e., the fluorescence visible to the surgeon through the microscope. In addition, light from the tumor cavity is divided between surgeon, observer, and camera system.
Using spectrometry or confocal microscopy [42, 58, 59], porphyrins can be detected even when no macroscopic fluorescence can be observed. These findings indicate that low-grade gliomas commonly accumulate porphyrins which are difficult to perceive using the surgical microscope, based in part on the comparative amount of accumulation per cell but also on the low cell density observed in low-grade gliomas.
Tumors Other Than Gliomas that Accumulate Useful Fluorescence
Multiple groups have investigated brain tumors apart from gliomas regarding their capability of accumulating visible porphyrin fluorescence (e.g., Marbacher et al. [60]). Also, several reports provide data on children [61–64] regarding the specific pathologies in this patient population. In children with high-grade gliomas, similar usefulness of 5-ALA-induced porphyrins has been reported as with adults. Grade II and III ependymomas seem to avidly accumulate porphyrins. Medulloblastomas, pilocytic astrocytomas, and PNETs were found to display fluorescence in up to 50% of cases, which was found useful by surgeons in about 20% of patients (Table 6.3).
Table 6.3
Non-glioma brain tumors and ALA versatility
Tumor type | Visible fluorescence | Useful? | Reference examples |
---|---|---|---|
Meningioma Low-grade High-grade Dural infiltration Bony infiltration | +++ +++ ++ ++ | −+a +++ ++ ++ | |
Ependymoma Grade I Grade II Grade III | +++ +++ +++ | ++ ++ ++ | |
Hemangioblastoma | +++ | + | Utsuki et al. [94] |
PNET | +, 40% | −+, 20% | Stummer et al. [64] |
Ganglioglioma | +, 40% | +, 40% | Stummer et al. [64] |
Medulloblastoma | +, 50% | +−, 20% | |
Pilocytic astrocytoma | +, 22% | a−, 18% | Stummer et al. [64] |
Pituitary | + | + | Eljamel et al. [79] |
Metastasis | ++, 70% | + | Kamp et al. [77] |
Stereotactic biopsy (lymphoma, inflammation, HGG, metastasis) | +++ | +++ |
As is our own experience and from the literature available so far, meningiomas have a strong propensity for accumulating protoporphyrin IX (Table 6.3). This characteristic may not be of much practical value in the typical low-grade convexity meningioma that is easily identified and resected using conventional illumination. On the other hand, porphyrins have been observed in zones of bony invasion as well as in the dural infiltration zone [65–73]. In recurrent atypical or anaplastic meningiomas, especially after surgery and radiotherapy with inherent scarring, 5-ALA-induced porphyrins help identify residual tumor cells [70]. The use of ALA has been reported for pituitary adenoma, hemangioblastoma, ependymomas, metastasis, and gangliogliomas (Table 6.3). Schwannomas have been found not to accumulate significant fluorescence [60].
It is of interest to note that all investigators of tumors apart from malignant gliomas use the same dose and timing of application for 5-ALA as initially published by our group in 1998, i.e., 20 mg/kg orally 3 h prior to induction of anesthesia. Particularly in low-grade gliomas or other benign cerebral tumors, metabolism of 5-ALA to porphyrins may well differ from its metabolism in highly proliferative malignant gliomas. The question of using different doses or timelines of application of 5-ALA has not been addressed so far for these tumors. Even in high-grade gliomas, we are now giving 5-ALA earlier, 4–5 h prior to induction of anesthesia. Our recent data (manuscript in preparation) suggest that porphyrin accumulation peaks at 7–8 h rather than 6 h after ingestion. Thus, assuming a time period of 3 h from induction of anesthesia to surgically approaching the marginal tumor after positioning, draping, craniotomy, and debulking, administration 4–5 h prior to surgery appears recommendable.
5-ALA has been suggested to be useful in the context of stereotactic biopsies [74–76]. Investigators argue that detecting fluorescence in a stereotactic biopsy will prove that the target has been correctly located, thus reducing the need for intra-operative pathology or serial sampling. While this may be true for malignant gliomas and lymphomas, lesions such as abscesses or metastasis may show unspecific fluorescence in perifocal tissue [77].
Practical Implementation of 5-ALA
Patient Selection
If a patient presents with imaging suggestive of a malignant glioma (contrast-enhancement, necrosis, edema) and a metastasis is unlikely, these patients will be given 5-ALA 4–5 h prior to induction of anesthesia. In suspected gliomas without contrast-enhancement, we will base our decision to give 5-ALA on FET-PET. If the uptake ratio in the lesion exceeds 1.85, such patients will also be treated with 5-ALA. We do not restrict ourselves to tumors where gross total resections can be expected. Investigators currently assume that resection rates beyond 70% will improve outcome in patients with glioblastomas [6] and that above this threshold, survival will depend on the amount of tumor additional resected, optimally all of contrast-enhancing tumor. Thus, whenever we perform resections for malignant gliomas, we utilize 5-ALA for maximizing resection even if we are at times forced to leave infiltrating or gross tumor behind, the latter if there is a risk that perforators are involved in the tumor or due to the proximity to important tracts or areas of cortex.
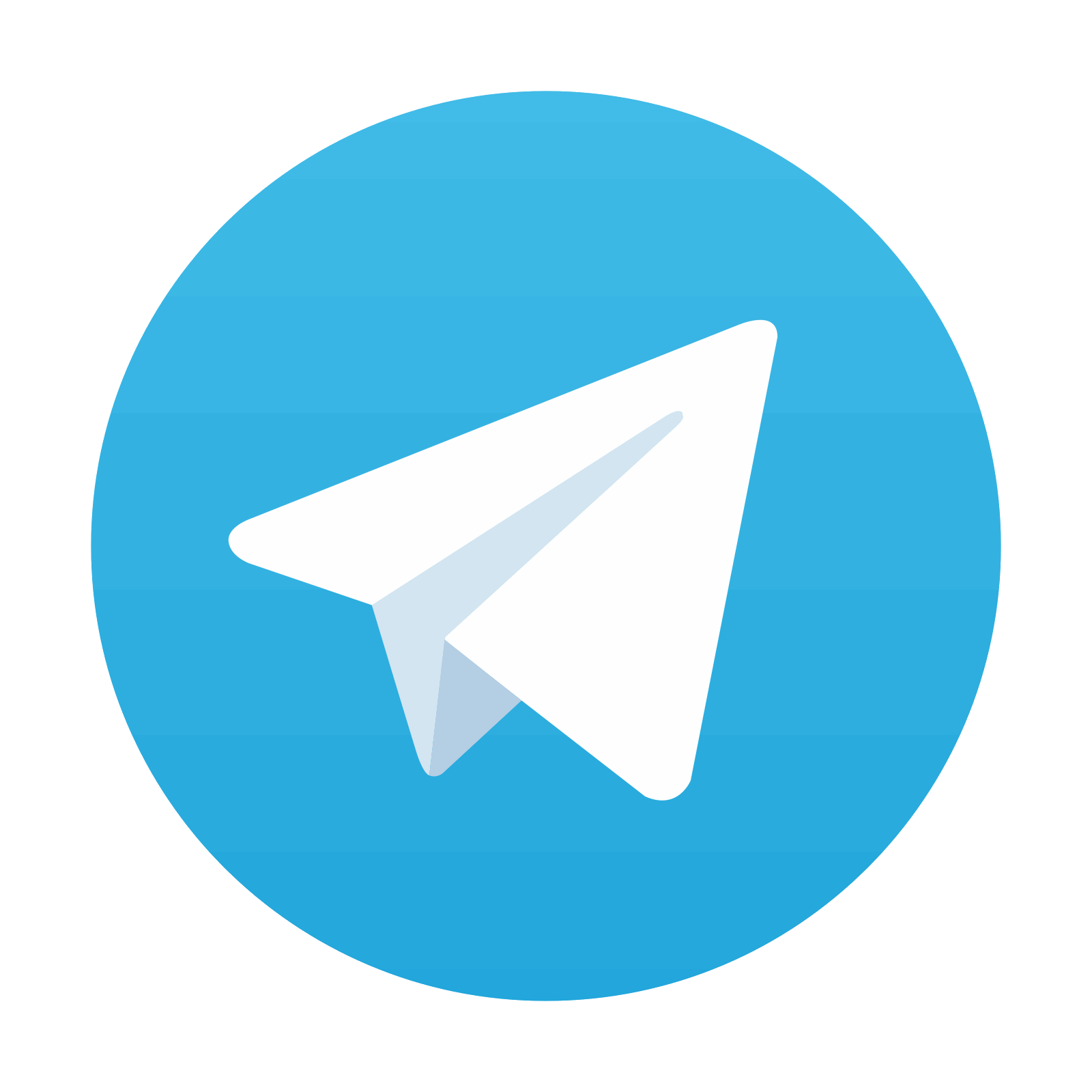
Stay updated, free articles. Join our Telegram channel

Full access? Get Clinical Tree
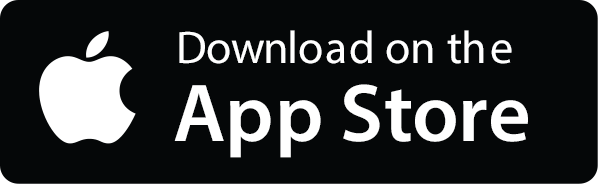
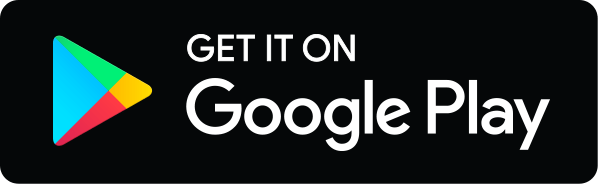