Fig. 1
(a) Head CT of a 69-year-old male status post left frontal craniotomy for subtotal skull base meningioma resection with increasing ICH and edema in the left frontal and temporal lobes causing left-to-right midline shift. (b) The interictal EEG showed preceding continuous LPDs with spiky morphology independently over the left centrotemporal and left posterior temporal-parietal regions at a frequency of 0.5–1 Hz. The ictal discharge evolves up to 1.5–2 Hz and changes into a rhythmic delta frequency discharge plus superimposed fast activity over the left frontocentral region which slows down to 1 Hz then ends
Acute Subarachnoid Hemorrhage
Acute subarachnoid hemorrhage (SAH) is complicated by seizures in 4–19 % of patients, and SE occurs in 10–14 % of patients. One study reported that seizures occurred in 19 % of 108 patients with an admission diagnosis of SAH undergoing cEEG. NCS and NCSE were present in 18 % and 13 % of this cohort, respectively [4]. NCSE has been associated with poor clinical outcome. In one study, NCS were observed in 15 % of 116 patients with SAH monitored with cEEG. NCSE occurred in 11 %. The clinical outcome was poor in 92 % of the 12 patients with NCSE. NCSE within the first 24 h had 100 % specificity and 100 % positive predictive value for poor outcome at 3 months (defined as a modified Rankin Scale Score ≥ 4) [12]. Seizures within the first 24 h after SAH may lead to poor scores on a severity scale upon initial presentation; however, these can achieve a good outcome. In this study, seizures were observed in 13 % of 425 patients with SAH. Early-onset seizures (within the first 24 h) negatively influenced the World Federation of Neurosurgical Societies grading regarding the severity of the SAH. Early-onset seizures were significantly associated with poor grades on this scale [13]. All SAH patients should be monitored with cEEG for at least the first 24–48 h for the detection of NCS and NCSE with longer duration monitoring used for the detection of delayed cerebral ischemia due to vasospasm after SAH.
Acute Subdural Hemorrhage
Seizures and epileptiform discharges are relatively frequent in patients with acute subdural hemorrhage (SDH), and seizures can have a negative impact on early functional outcome. One study reported clinical seizures in 22 % of 134 patients with acute or acute-on-chronic SDH. Epileptiform discharges were present in 21 % of 134 patients. Seizures worsened early functional outcome in these patients [14]. In a smaller series based on the previous study, the EEGs of 24 patients who underwent evacuation for acute or acute-on-chronic SDH were analyzed. Eighty-seven percent of these 24 patients had epileptiform discharges on EEG, with 62 % of the discharges originating from the midline regions. Lateralized periodic discharges (LPDs) were present in 43 % of those patients with epileptiform discharges. NCS were present in 12 % of 24 patients, and the seizures were characterized as focal or multifocal in onset. Both LPDs and midline epileptiform discharges were associated with the degree of midline shift on neuroimaging. Poor early outcomes were associated with the presence of bilateral, bilateral independent, multifocal, and midline epileptiform discharges [15]. LPDs are known to be highly associated with focal-onset seizures. In a small case series of five patients with acute SDH who underwent surgical evacuation, focal motor or sensory seizures were present in all of these patients. In addition, all five patients had LPDs on EEG that were ipsilateral to the side of the SDH [16]. An illustrative case is shown in Fig. 2.

Fig. 2
(a) Head CT of a 73-year-old male who presented with altered mental status and an acute left hemispheric SDH. (b) The ictal EEG shows ictal LPDs consisting of low-amplitude spikes with superimposed rhythmic delta activity at a frequency of 1 Hz over the left frontal region. The ictal LPDs correspond to rhythmic clonic right facial twitching which is seen on the EEG as muscle artifact over the right hemispheric electrodes, maximally involving the right temporal electrodes, which is time locked with the left frontal LPDs
Acute Traumatic Brain Injury
Seizures have been reported in 12–50 % of patients with traumatic brain injury (TBI), and SE occurs in 8–35 % of patients. cEEG monitoring is being used more often in these patients for the detection of subclinical electrographic seizures. One study reported that any seizure occurred in 18 % of 51 patients with an admission diagnosis of TBI undergoing cEEG. NCS and NCSE were present in 18 % and 8 % of this cohort, respectively [4]. Seizures are associated with increased intracranial pressure (ICP) and metabolic derangements and may cause worsening neuronal damage in patients with severe TBI. In another study using cEEG, 50 % of 20 patients with severe TBI monitored for seven days after injury had NCS. Thirty-five percent of these patients were in NCSE. Seizures were focal in onset with secondary generalization in 78 % of cases, and most were from the frontotemporal regions. There was an early peak seizure period at 29 h and a later peak seizure period at 140 h after injury.
NCS were associated with increased ICP, especially delayed increases in ICP beyond 96 h. The mean ICP was higher in the seizure group compared with the non-seizure group. The ICP nearly doubled with the occurrence of seizures. Metabolic derangements also occurred in the seizure group. The mean lactate/pyruvate ratio (LPR) was higher in patients with seizures, which is evidence that posttraumatic electrographic seizures negatively affect brain metabolism and may lead to permanent cellular injury. Animal models have shown that AEDs given to stop posttraumatic seizures improve outcome [17]. In fact, the current standard of care in patients with severe TBI is to give IV fosphenytoin for the first 7 days after injury to reduce the incidence of early posttraumatic seizures. In patients with severe TBI, phenytoin was associated with a 73 % decrease in the risk of seizures in the first week, but there was no protective effect from day 8 up to the end of the second year of the study. Phenytoin was thought to have an early suppressive effect but not a true prophylactic effect [18].
Seizures after moderate to severe TBI may be generalized or focal in onset with characteristic EEG patterns and clinical signs. SE in this population is associated with a high mortality rate with superimposed early hypoxic injury often present. Another study reported that 22 % of 94 patients with moderate to severe brain injury had seizures (clinical or electrographic), and 6 % of patients were in SE. Of patients with electrographic seizures, 55 % were of generalized onset, and 45 % were of focal onset. The electrographic patterns seen in SE patients consisted of secondarily generalized polyspike and wave, secondarily generalized repetitive spikes, and focal status epilepticus with clinical symptoms consisting of rhythmic facial twitching, eyelid fluttering, and irregular myoclonus. All patients with posttraumatic status epilepticus died, but the majority of these patients had early hypoxic injury. Therefore, status epilepticus in these cases with possible superimposed hypoxic-ischemic insult may have been a marker of severe injury and portended death. Some EEG patterns consisting of sudden-onset rhythmic epileptiform discharges that did not evolve were seen but not considered to be seizures because they did not meet the criteria [19]. These EEG patterns may now be characterized as on the ictal-interictal continuum, discussed elsewhere in the book.
Acute Brain Abscess/Subdural Empyema
Brain abscesses may occur in isolation or in association with meningitis. EEG findings in patients with focal abscesses may include focal polymorphic delta frequency slowing, loss of faster frequency activity, and occasional epileptiform activity consisting of LPDs or focal spikes. Focal seizures may also occur [20]. Subdural empyemas may lead to focal seizures in at least 80 % of patients. The seizures are often related to underlying cortical vein thrombosis [21].
Posterior Reversible Encephalopathy Syndrome
Posterior reversible encephalopathy syndrome (PRES) presents as altered mental status, headache, and visual disturbances resulting from vasogenic leakage associated with certain clinical conditions (e.g., eclampsia, hypertensive encephalopathy, neurotoxic substances, and immunosuppressants). The resulting edema affects the white matter and cortex usually in a posterior maximum, symmetrical distribution. Seizures are common in PRES. In a case series of 49 patients diagnosed with PRES, 78 % suffered from seizures. Two patients had epileptiform activity present on their EEGs, either LPDs or occipital sharp and slow waves. These two patients had a focal seizure presentation either with focal motor seizures or seizures affecting the visual hemifield. The most frequent seizure type however was a single short “grand mal” seizure, with no seizures present beyond the first day. Focal interictal EEG abnormalities correlated with focal clinical seizures. Seizures did not recur beyond 24 h, and chronic epilepsy did not develop in these patients [22].
Chronic Focal Injuries and EEG Changes
The major forms of chronic focal lesions that cause epileptiform abnormalities on EEG are tumors, trauma, old cerebrovascular events, arteriovenous malformations, and malformations of cortical development. The EEG abnormalities seen in these pathophysiological states are described below. While there is no clear one-to-one correspondence between the specific pathology and the abnormalities seen on the EEG, the propensity to cause different abnormalities varies depending on the underlying pathology and the anatomical location of the lesion.
Tumors
Epileptic seizures are known to be a common presenting symptom in patients with brain neoplasms (between 15 and 100 % of patients with brain tumors). Recently, NCS or NCSE is increasingly being recognized as a cause of neurological worsening and less commonly as a presenting symptom of patients with brain tumors. In a case series involving 147 patients newly diagnosed with brain tumors, 38 % of the patients with a primary neoplasm and 20 % of patients with metastatic lesions had seizures as presenting symptom [23]. Oligodendrogliomas and grade 2 astrocytomas are more likely to present with seizures [23], and gliomas are thought to contribute to NCS and NCSE either at the onset or over the course of disease progression [24]. Patients with gliomas who have NCS can present with confusion, aphasia, and disorientation [25]. One recent study on patients hospitalized with a diagnosis of brain tumor demonstrated that 2 % of these patients had NCSE and 54 % had NCS. Treatment resulted in clinical improvement in 75 % of these patients [26]. The authors concluded that the NCSE may be underdiagnosed in patients with tumors because of the absence of obvious clinical manifestations. This study suggested that aggressive treatment of the NCS could improve clinical outcome in patients with tumors. Illustrative cases are shown in Figs. 3 and 4.

Fig. 3
(a) MRI of a 10-year-old male with complex partial seizures and an epidermoid cyst over the left anterior temporal region which was resected 3 years ago. (b) The ictal EEG shows a seizure originating from the left temporal region consisting of a rhythmic theta frequency discharge

Fig. 4
(a) Brain MRI (axial and coronal views) of a 72-year-old female with a history of new-onset epilepsy presenting as status epilepticus 3 months earlier who was readmitted with altered mental status and left upper-extremity weakness and developed convulsive status epilepticus. The MRI shows increased signal with mass effect involving the right frontotemporal region. Brain biopsy of this region confirmed anaplastic astrocytoma. (b) The ictal EEG shows ictal LPDs consisting of low-amplitude sharp waves with superimposed rhythmic delta activity at a frequency of 1 Hz over the right centroparietal region. The ictal LPDs correspond to rhythmic clonic twitching of the left neck, arm, and trunk which is seen on the EEG as muscle artifact over the left hemispheric electrodes, maximally involving the left parietal-posterior temporal electrodes, which is time locked to the right centroparietal LPDs
One of the major triggers for NCS noted in patients with intracranial neoplasms is fluctuation in AED levels. This can occur due to noncompliance or drug interactions. Anticancer drugs, including nitrosoureas, cyclophosphamide, vincristine, and methotrexate, interact with some older AEDs and can cause sudden changes in the medication levels. In some cases, patients with seizures related to brain neoplasms will have seizures triggered by an immediate cause such as systemic infection, brain tumor edema, acute exacerbation of pre-existing brain disease, alcohol use, or lack of sleep. Given these risk factors, patients with brain tumors presenting with alteration of mental status should be evaluated with an EEG early in the course of the workup. The pathophysiology of seizure development in patients with focal lesions like tumors includes peritumoral amino acid disturbances, local metabolic imbalances, edema, disordered neurotransmitter and receptor balances, and pH alterations [27, 28].
As a result of the abovementioned processes in the brain, abnormal EEG patterns can be seen in the patients with brain tumors early in the course of the disease. EEGs performed in the evaluation of new brain tumors commonly show monomorphic or polymorphic delta activity with reduced fast activity. The presence of slow waves on the EEG signifies loss of function or disturbances of the surrounding region. Periodic discharges can be seen on the EEG as the tumor progresses and involves more areas of brain tissue. Sometimes focal delta activity or periodic discharges may be the only initial indication of a focal abnormality that triggers further workup. In one study, out of 282 patients with LPDs, 18 % had focal tumors. Depending on the location of the tumors, different types of intermittent rhythmic delta activity (IRDA) may be noted (frontal, FIRDA; temporal, TIRDA; occipital, OIRDA). In addition, occipital tumors will be associated with a depression in the posterior-dominant rhythm. In the case of temporal gliomas, the delta activity is more continuous compared to the other locations.
EEG manifestations in patients developing NCS or NCSE depend primarily on the location of the tumor. The tumor itself may not produce much electrical activity, but the tissue surrounding it is capable of producing electrical activity. The patterns of EEG changes range from slow waves and PDs to frank seizures. In addition, high-grade tumors can cause local tissue necrosis and hemosiderin deposition (which is a trigger for epileptogenesis) while the tumor is present or sometimes long after it has been removed. Since the tumor itself may not contain functional neuronal tissue, the brain tissue adjoining the tumor usually produces the epileptiform discharges or NCS. Depending on the functional role of the cortex involved, this electrical activity may or may not produce clinical seizures.
An often-noted finding in ICU patients undergoing cEEG, especially with intracranial tumors, is the presence of PDs with or without NCS. In a study done on patients discharged from the ICU with these findings, it was demonstrated that among the patients who had PDs without NCS, 25 % of them developed seizures during the follow-up period (11.9 ± 6 months), compared to 61 % of the patients with NCS during the ICU stay [29]. In this population, 100 % of the patients with tumors had PDs. Also, patients with LPDs were ten times more likely to have focal lesions.
Trauma
The EEG changes after trauma depend on the areas affected by trauma, the severity of the trauma, and the stage of healing after the trauma. Trauma to the white matter results mainly in slowing of the EEG rhythms with an increase in delta frequency activity. The EEG changes related to white mater injury usually persist for longer periods of time compared to changes seen after gray matter injuries. In the case of gray mater injuries, because of the significant neuroplasticity, the changes are usually dynamic with a normal or increase in the persistence of alpha activity in the early stages. This alpha rhythm is thought to represent the idling rhythms of the cortex and appears more persistent in the early stages of trauma, as the cortex is not functionally integrated into the rest of the brain. As the regeneration of the cortex improves and is better integrated into the rest of the cortex, the rhythm changes into more beta and theta activities. Previous studies on the EEG changes related to mild traumatic injuries showed that there will be a slowing of the posterior-dominant rhythm and increase in the theta rhythm in the early stages [30]. In an earlier study done on 344 patients admitted with head injury undergoing serial EEGs, up to 51 % of the patients continued to show the initial EEG abnormalities 3 days after injury, and the majority of EEG abnormalities resolved in 3 months [31]. Additional EEG changes related to focal damage to the brain include background slowing as well as PDs. Quantitative EEG studies have shown reduced power in all frequency bands, increased power in the delta frequency range, and changes in coherence and phase delays after concussions [32]. In addition to the milder EEG changes noted above, old trauma and related pathophysiological changes, including hemosiderin deposition, can result in convulsive and nonconvulsive seizures [33]. As mentioned in the case of tumors, the nature of the seizures depends on the location of the injury. Regarding the epileptogenicity of head trauma, it is reported that TBI accounts for up to 20 % of symptomatic epilepsy. EEG changes and epileptiform discharges related to the specific areas affected may be expected in patients having posttraumatic epilepsy. Immediate posttraumatic NCS can result in both episodic and long-lasting increases in intracranial pressure and changes in the lactate/pyruvate ratio measured with microdialysis [17]. Also over the long term, NCS are associated with hippocampal atrophy.
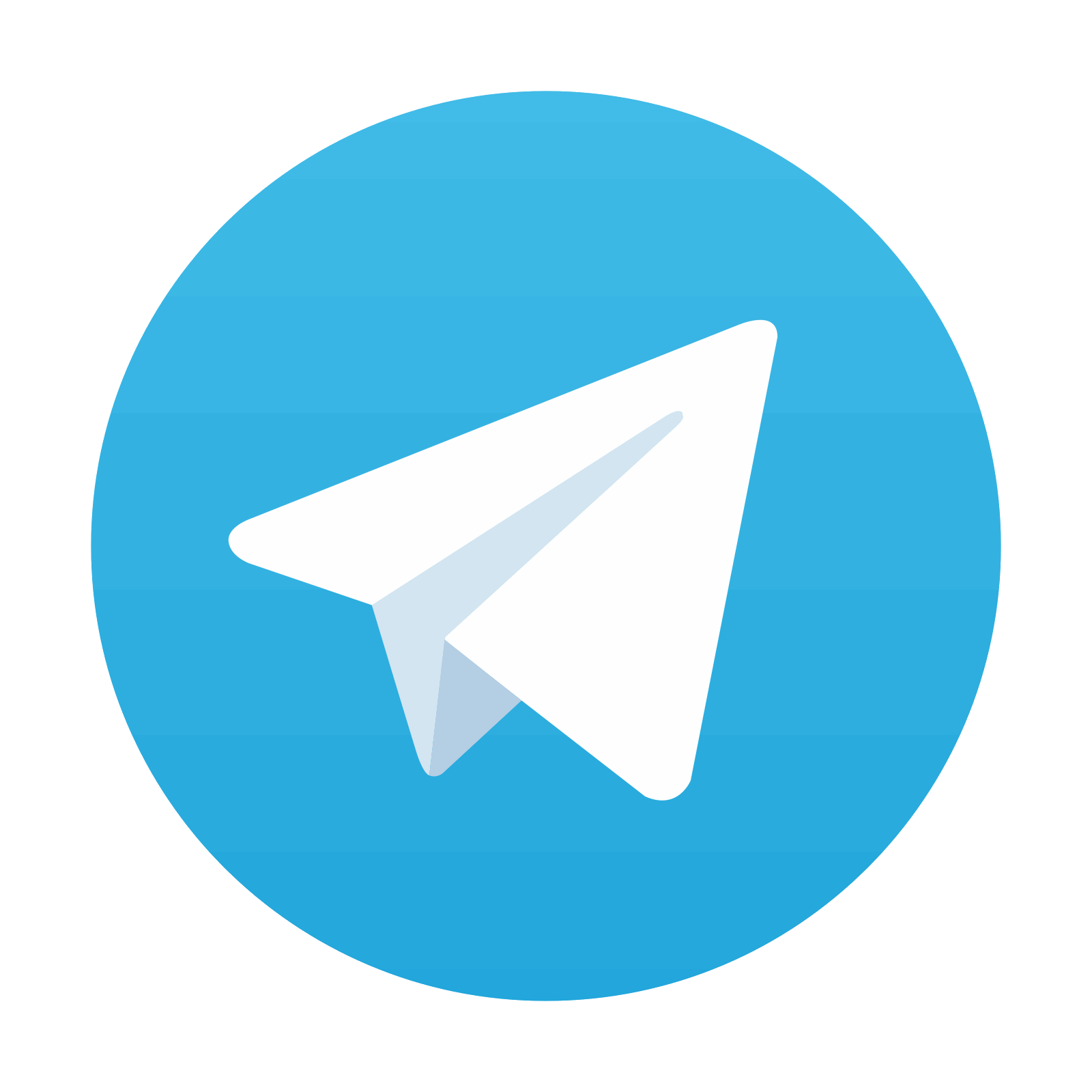
Stay updated, free articles. Join our Telegram channel

Full access? Get Clinical Tree
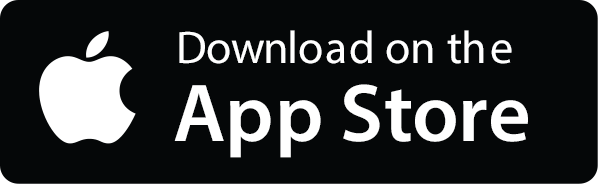
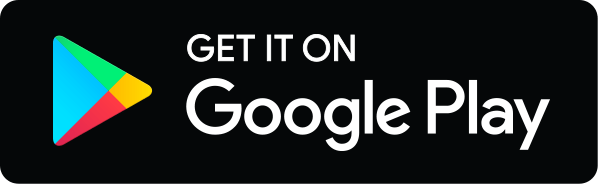