INTRODUCTION
Modern imaging techniques such as functional magnetic resonance imaging (fMRI), positron emission tomography (PET) and single photon emission tomography (SPECT) provide the groundwork to test mechanistic hypotheses derived from basic neuroscience studies in healthy individuals and patients with neurological and psychiatric disorders. Apart from these scientific applications, functional imaging studies can also provide important diagnostic information in the field of geriatric psychiatry. Therefore, functional imaging scans are recommended by diagnostic guidelines to resolve certain differential diagnostic issues1, and costs are also reimbursed for these special cases in selected countries (e.g. Medicare Coverage Criteria for FDG-PET for Alzheimer’s disease, http://nps.cardinal.com/nps/PETFoundations/documents/PETADCovFinal.pdf). In addition to its benefits for the diagnosis and differential diagnosis of neurological and psychiatric disorders, functional brain imaging has also been shown to increase the confidence of experts in their clinical diagnosis. For example, Foster et al.2 reported that visual rating of 18F-fluoro-2-deoxy-glucose (FDG) PET scans after a short training period was more reliable and accurate in distinguishing Alzheimer’s disease from frontotemporal dementia (FTD) than clinical assessment on its own. This was particularly true when the raters were uncertain with their clinical diagnosis. Hence, FDG PET is able to add important diagnostic information that appropriately improves diagnostic confidence.
Biomarkers including functional imaging are also gaining importance for the evaluation and monitoring of treatment effects. Modern, sensitive techniques are able to detect subtle brain functional changes resulting from therapeutic interventions, even if the therapeutic effect is not immediately mirrored by clinical improvement. This could lead to the identification of treatment responders in very early treatment phases3 or before the actual treatment period has even started. Furthermore, for some potentially preventive treatments to have their greatest impact, patients need to be identified at the presymptomatic stage. This means that individuals with an increased risk for a certain disease, but who do not yet show any clinical signs, need to be identified. Persons at risk for Alzheimer’s disease, for example, can already be identified on the basis of brain metabolic deficits in their FDG PET scans before first symptoms, such as forgetfulness, appear4.
This chapter provides an overview of a selection of functional imaging techniques and brain functional changes associated with ageing, some prevalent neurodegenerative and psychiatric disorders, and those due to treatment. Important clinical aspects and future research perspectives will be discussed.
Functional neuroimaging is broadly defined as techniques that provide measures of brain activity. Commonly used techniques are electroencephalography (EEG), magnetoencephalography (MEG), PET, SPECT and fMRI. Here we focus on PET and fMRI, which primarily aim at the regional mapping of mental processes. These techniques measure metabolic and haemodynamic brain processes closely linked with neuronal activity. PET and fMRI allow both the detection of regional activity changes in response to experimentally presented stimuli or cognitive tasks and the detection of synchronous co-activity changes across brain regions in response on stimuli or during rest. In this section we review the biological basis of functional neuroimaging signals, then we describe the physics of PET and fMRI signals, and finally we discuss two basic principles underlying functional neuroimaging data analysis: functional specialization and functional integration.
Behaviourally evoked changes in blood flow are at the heart of functional neuroimaging5. Blood flow changes reflect metabolic changes, which in turn reflect changes in neuronal activity. From a metabolic point of view neuronal activity is dominated by transmitter recycling. Eighty per cent of brain energy is used for glutamate recycling. Central to glutamate recycling are aerobic glycolysis and oxidative phosphorylation. These activity-dependent processes with their main substrates H2O, O2 and glucose provide the basis for15 O-H2O-PET, FDG PET, and blood oxygen level-dependent (BOLD) fMRI.
Oxygen-15 and fluorine-18 are isotopes with short half-lives that are introduced into the body incorporated into H2O and glucose. As the tracers decay, emitted gamma rays are detected by the PET scanner and used for image construction. The spatial resolution is about 1.5 cm and the temporal resolution is about 60 s; both values strongly influence the experimental design of neurocognitive paradigms. The fundamental signal for BOLD fMRI comes from hydrogen atoms mainly bonded to H2O within the brain. In the presence of a static magnetic field, these hydrogen atoms absorb energy that is applied at a characteristic radio frequency. After radiofrequency excitation, hydrogen atoms emit energy at the same radiofrequency until they gradually return to their equilibrium state (resonance phenomenon). The MRI scanner measures the emitted radiofrequency energy. The measured radiofrequency signal decays over time, influenced by distinct factors, including the presence of inhomogeneities in the magnetic field. BOLD fMRI techniques are designed to measure primarily changes of inhomogeneity within a small volume of tissue that result from changes in blood oxygenation. Deoxy-and oxyhaemoglobin (Hb-CO2, Hb-O2) have different magnetic properties; Hb-CO2 is paramagnetic and introduces an inhomogeneity into the nearby magnetic field, whereas Hb-O2 is weakly diamagnetic with little effect. Hence, an increase in the concentration of Hb-CO2 causes a decrease in image intensity, and a decrease in Hb-CO2 causes an increase in image intensity (BOLD effect). The spatial resolution of BOLD fMRI is about 0.5 cm and temporal resolution about 1-4 seconds.
There are two key topics in the analysis of functional imaging data, reflecting the long-standing debate in neuroscience about functional specialization versus functional integration in the brain6. The first is brain ‘mapping’, where three-dimensional images of neuronal activation are generated showing which parts of the brain respond to a given sensory stimulus or cognitive task. This is also known as the study of functional specialization and generally proceeds using some form of statistical parametric mapping (SPM) based on a voxel-wise analysis. A classic example here is the identification of human V4 and V5, the areas specialized for the processing of colour and motion. The second topic is ‘functional integration’, where models are used to analyse how different brain areas interact. A classic example is the use of models to find increased connectivity between dorsal and ventral visual streams after subjects learn object-place associations. A large number of statistical techniques are used to detect interregional connectivity (e.g. independent component analysis, support vector machine, dynamic causal modelling).
AGE-RELATED FUNCTIONAL CHANGES
Brain Functional Decline
‘Normal ageing’ is associated with numerous biological deteriorations in the human body, which also include brain functional changes. However, ‘normal ageing’ is a rather fuzzy concept and it is sometimes difficult to separate age-related and disease-related brain changes. All the same, with age the brain undergoes both structural and functional changes that underlie subjective complaints. The pre-frontal cortex shows the most prominent functional deterioration in most studies, whereas other regions including the anterior hippocampus, the thalamus and the posterior cingulate cortex are affected to a much lesser extent7. These results support the theory that the first areas to emerge phylo-and ontogenetically are the most resistant to age effects and the last to emerge are the most vulnerable. Furthermore, the relative functional preservation of brain structures during ‘normal ageing’ that are affected early in Alzheimer’s disease, such as the hippocampus and the posterior cingulate cortex8, seems to mark the parting of the ways between ageing and brain disease. This is also supported by the finding that cognitively normal elderly individuals with a pathological brain metabolic pattern are more likely to experience memory decline9 and are at elevated risk of developing symptoms of Alzheimer’s disease10. Also in line with these findings, Alzheimer’s disease-like brain functional patterns are also found in cognitively normal elderly individuals with an increased genetic risk for Alzheimer’s disease (i.e. carriers of the apolipoprotein E allele ε4 (APOE4)11). Alzheimer’s disease-like changes are already present at even younger ages in pre-symptomatic individuals from families with known early-onset autosomal dominant Alzheimer’s disease-carrying mutations in the PRESENILIN1 gene12. Interestingly, a maternal history of Alzheimer’s disease also predisposes cognitively normal individuals to progressive metabolic deficits in Alzheimer’s disease-vulnerable brain regions, which may be related to a higher risk of developing the disease4. Although the exact causes of these abnormalities are not yet known, these results suggest the involvement of maternally inherited predisposition to brain metabolic failure, possibly connected to mitochondrial DNA. The facts that mitochondrial DNA is entirely maternally inherited in humans, and diseases associated with mitochondrial DNA mutations often present as sporadic disorders, support this hypothesis.
Adaptation and Compensation
Age-and disease-related changes are not only associated with functional deterioration but also with counteractive measures. Accessory neural networks are activated to solve increasingly difficult tasks (adaptation). Similar mechanisms are deployed if a task cannot be solved by the usual means because of damage to the brain regions normally involved due to age-related changes or neurodegeneration (compensation)13. To offset brain functional deterioration, networks and cognitive patterns less susceptible to interference are activated; furthermore, additional networks not involved in young healthy individuals for the same task are recruited. These activity adjustments are exemplified by two well-described phenomena. The PASA (posterior-anterior shift in aging) phenomenon describes one possible reaction of the human brain to age-associated cognitive impairment. An increased activation of pre-frontal areas in conjunction with a decreased activation of occipital areas is found for the same cognitive paradigm in elderly individuals compared to healthy young persons. The degree of this activity shift is positively associated with task performance14. The HAROLD (hemispheric asymmetry reduction in older adults) phenomenon, on the other hand, delineates a reduction of hemispheric asymmetry in elderly individuals15; compared to young individuals, elderly persons tend to activate additional contralateral and to deactivate ipsilateral brain regions for the same cognitive task. Again, task performance positively correlates with the degree of the activity change. Taken together, PASA and HAROLD are good examples of the three possible mechanisms of cerebral activity changes associated with efficient information processing during ageing: (i) increased activation of brain regions already involved at the same task difficulty level in young individuals; (ii) activation of additional networks in other brain regions; (iii) more efficient deactivation of interfering networks.
FUNCTIONAL CHANGES IN NEURODEGENERATIVE DISORDERS
Neurodegenerative disorders are associated with distinct brain functional patterns. These patterns help to separate different disorders and to identify patients at oligosymptomatic stages.
Mild Cognitive Impairment and Alzheimer’s Disease
In Alzheimer’s disease functional brain damage is typically found in the hippocampus, and the pre-frontal, temporoparietal and posterior cingulate association cortices8,16. The hippocampus and the posterior cingulate cortex seem to be affected in very early disease stages; metabolic deterioration of these two regions is already present in patients with mild cognitive impairment (MCI)17,18. Furthermore, an FDG PET suggestive of Alzheimer’s disease in patients with MCI is a strong predictor for progression to dementia, especially in the presence of other risk factors such as an APOE e4 allele19. The degree of posterior cingulate cortex hypometabolism is also positively correlated with clinical disease severity in MCI20. However, several studies show that there is no linear association between brain damage and clinical symptoms, and that individual characteristics related to cognitive reserve modify the association between neurodegener-ative damage and clinical signs21-23. Therefore, clinical symptoms tend to be less severe in individuals with higher estimated cognitive reserve than would be predicted by the amount of their brain damage.
Frontotemporal Lobar Degenerations
The clinical syndromes FTD, semantic dementia (SD) and non-fluent progressive aphasia (NFPA) have distinct morphological and functional imaging correlates. Furthermore, associations between specific clinical symptoms and focal functional brain pathology can be established. For example, metabolic reductions in brain regions related to micturition can be found in patients with FTD who suffer from urinary incontinence in contrast to those patients who do not experience this distressing symptom24.
In FTD, bi-hemispheric functional brain pathology, which predominantly affects the pre-frontal cortex, the anterior cingulate cortex, the caudate nucleus and the insula, is already present in early clinical stages25. As the disease progresses, the functional pathology crosses the boundaries of the pre-frontal cortex and spreads to temporal and parietal regions26. Davies et al.21 recently reported a group of patients with FTD without any structural brain changes that seem to have a better prognosis. However, brain functional alterations and the clinical relevance of this finding have yet to be explored. It is interesting to note that the degree of functional brain pathology is also influenced by genetic characteristics28 and cognitive reserve29,30. In SD, initially asymmetric, in most cases left-sided, functional lesions of the temporal cortex, more specifically the temporal pole, are found25
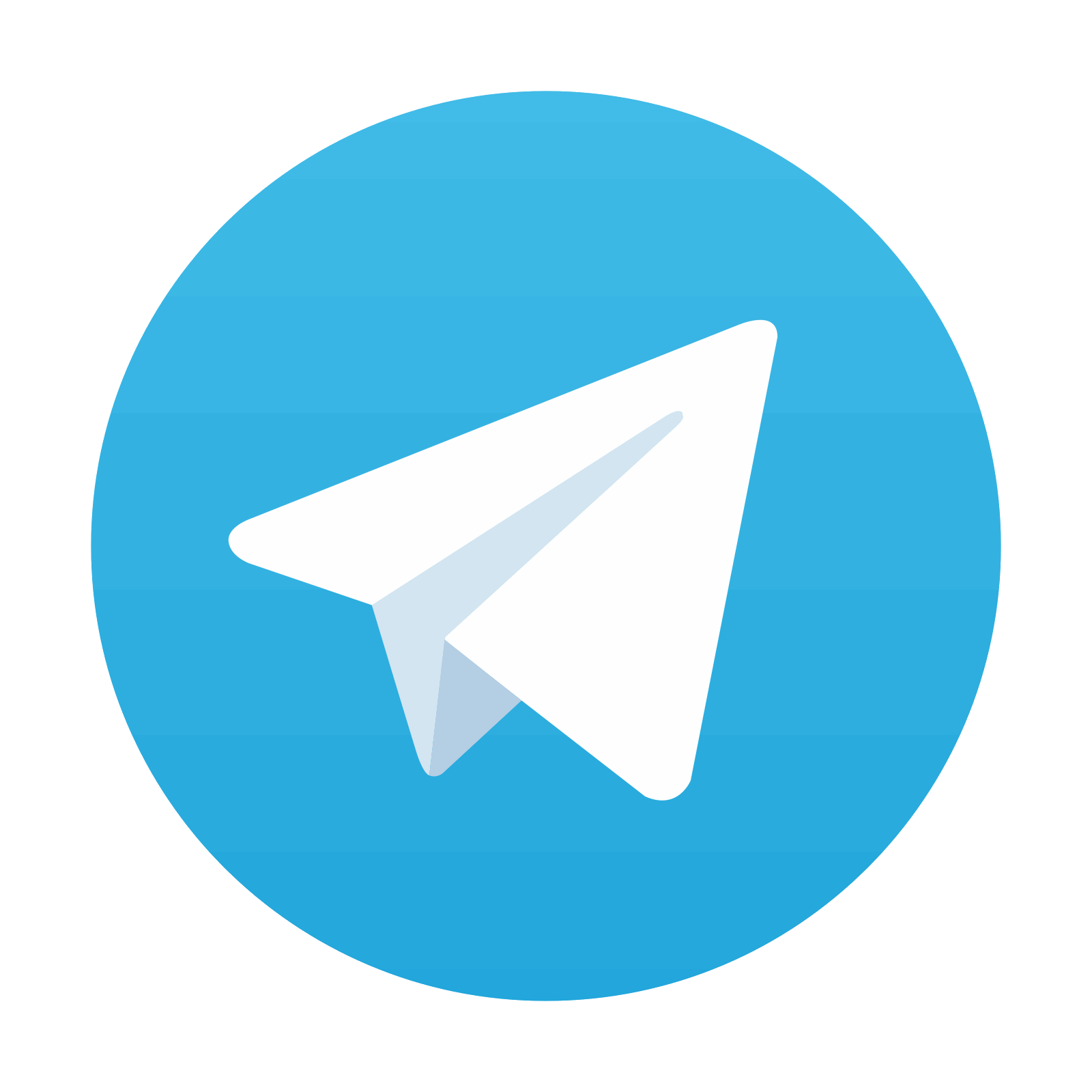
Stay updated, free articles. Join our Telegram channel

Full access? Get Clinical Tree
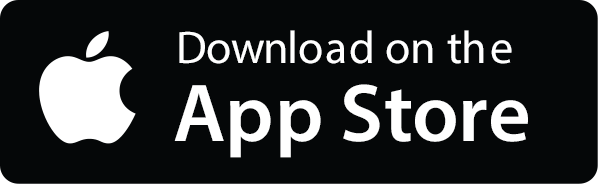
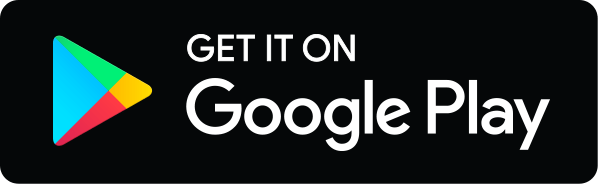