Fig. 1
Time-course analysis of early GL26-Cit glioma growth in the striatum in mice. To visualize perivascular glioma growth GL26-Cit cells were implanted in RA/EGxdelCre mice that express GFP in the brain endothelium. Invasive glioma cells maintain close vascular contact at all time-points analyzed over 120 h as they disseminate throughout the brain. GFP+ brain microvasculature has been pseudocolored red. Corresponding high-magnification micrographs (insets) detail perivascular invasion at the tumor border. White asterisks (*) relate the image area shown in the high-magnification micrographs with the corresponding area in the low-magnification micrographs. Perivascular tumor invasion begins 24 h post-implantation. Inset denoted by the carrot (^) in the 120 h micrograph is included to demonstrate the trapping of normal brain microvessels within the growing tumor mass as perivascular invasion is followed by tumor cell proliferation within the perivascular space. Time-points progress from top to bottom and from left to right
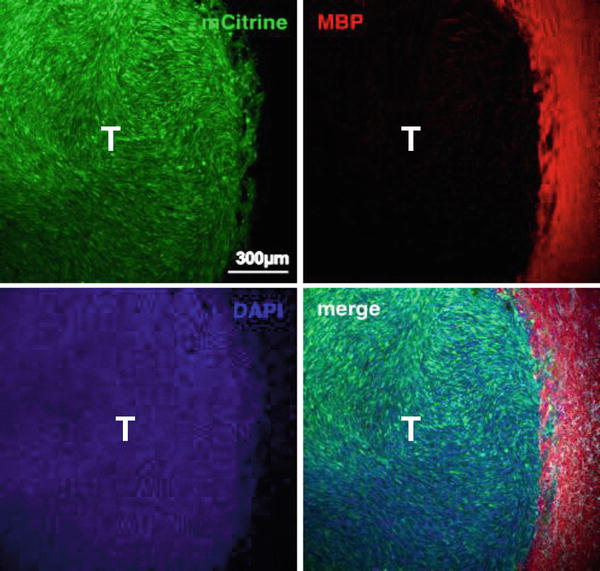
Fig. 2
Late-stage GL26-Cit intracranial glioma tumor. Scanning fluorescence confocal micrograph of syngeneic GL26-Cit mouse glioma (shown in green) 21-days post-tumor implantation into the striatum. Glioma cells were genetically modified to express mCitrine fluorescent protein prior to tumor implantation to facilitate direct tumor visualization by fluorescence microscopy. Individual and merged channels are shown. Tumor-bearing brain tissue was sectioned 50 μm thick and immunolabeled with anti-myelin basic protein (MBP) antibodies (shown in red) then counterstained with 4′,6-diamidino-2-phenylindole (DAPI) (shown in blue). Note that this late-stage tumor has well-defined borders compared to GL26-Cit tumors at earlier stages, which lack definition in their tumor borders and which exhibit extensive perivascular invasion. Also note that large myelinated axonal bundles that become compressed towards the outside of the growing tumor mass. Scale bar corresponds to 300 μm. T denotes tumor area
A number of key physiological processes such as the relative paucity of dendritic cells (DCs) in the brain , lack of lymphatic drainage, production of anti-inflammatory mediators such as TGF-β and nitric oxide (NO) by cells in the central nervous system (CNS ), as well as low MHCII expression on infiltrating microglia, protect the brain from an immune-mediated attack but at the same time, also contribute to its immune suppressive environment [6–9]. We have succeeded in developing an adenoviral mediated immunotherapy for brain tumors that is dependent on the expression of two genes: Thymidine kinase (TK), that phosphorylates the prodrug Ganciclovir which induces DNA cross-linking followed by cell death and fms-like tyrosine kinase-3 ligand (Flt3L), a potent DC growth factor that recruits DCs within the tumor microenvironment [10–12]. Tumor cell death induced by TK causes the release of intracellular proinflammatory molecules and tumor antigens, which are taken up by surveying DCs that subsequently prime the T cells to elicit an antigen specific cytotoxic antitumor immune response [10–12]. Combination therapy using these two adenoviruses induces tumor regression, long-term survival, and immunological memory in several mouse and rat GBM model s [10, 12, 13].
Gene therapy vectors can also be used for the treatment of neurodegenerative diseases. In order to be useful for gene therapy of chronic neurological disorders, vector systems should allow long-term transduction of brain cells in the absence of undesirable long-term side effects. Recombinant adenovirus vectors are powerful tools for gene delivery to the CNS [14, 15]. Adenoviruses can be easily purified to high titers and efficiently transduce differentiated cells such as neurons and glial cells. Additionally, transgene expression is restricted to the area of vector administration or areas that project to the injection site [16, 17]. The majority of current adenoviral-mediated gene therapy protocols utilize first-generation vectors, which are recombinant vectors that are non-replicative because of the deletion of the E1 region from the viral genome [18, 19]. While in the absence of prior immune priming to adenovirus, first-generation adenoviral vectors injected into the brain parenchyma can sustain prolonged transgene expression for months, activation of antiviral T cells by peripheral immunization leads to loss of vector-mediated transgene expression [17, 20]. To overcome this instability, “gutless,” high-capacity adenoviral (HC-Ad) vectors have been engineered in which all viral encoding genes have been eliminated and replaced with noncoding stuffer DNA sequences. Even in the presence of an anti-adenoviral immune response, expression from HC-Ad vectors remains stable for at least 1 year [21, 22]. Critical parameters that determine the efficiency of transgene expression are (1) dose and volume of adenoviruses administered, (2) vector backbone and the choice of promoter, (3) immune status of the animal, and (4) purity of the adenoviral vector stock [23, 24]. In this unit we provide detailed instructions for the implantation of various syngeneic GBM model s in rodents and adenoviral-mediated gene therapy using Ad-TK and Ad-Flt3L. Adenoviral-mediated transgene expression can be readily detected in treated brain tumors by immunocytochemistry. Figure 3 shows representative images from syngeneic rat CNS-1 model, U251 and U87 xenografts models injected intratumorally with Ad adenoviral vectors encoding TK. TK expression was assessed by immunohistochemistry and immunofluorescence. ELISPOT and T cell proliferation are extremely useful assays for testing the quality and quantity of T cell antitumor responses induced by immunotherapeutic approaches; hence the protocols for setting up these assays are also described [10, 12, 13, 25–29].
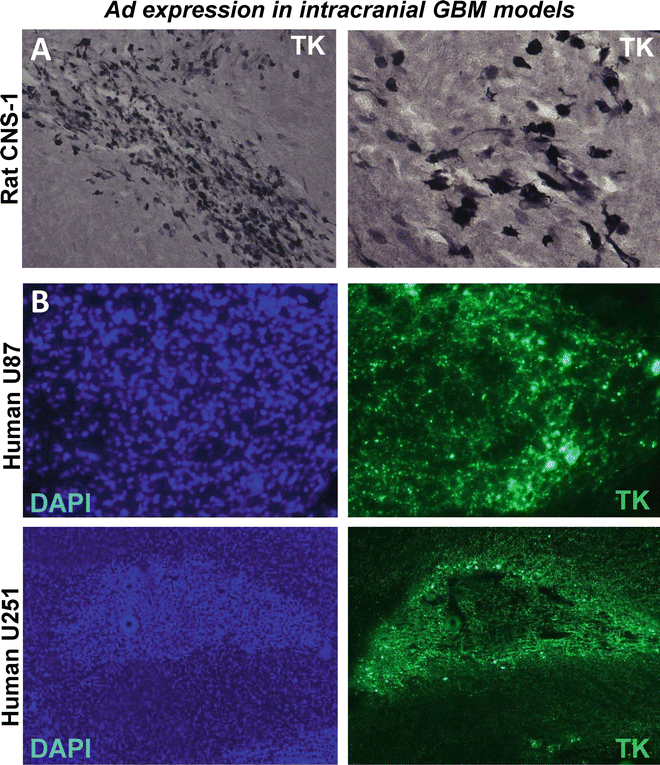
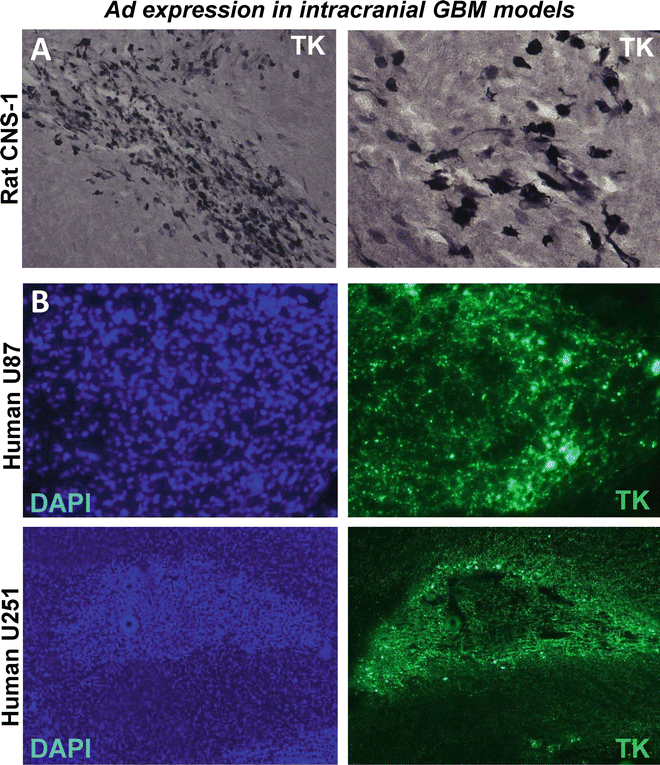
Fig. 3
Adenoviral-mediated transgene delivery into intracranial GBM in rodent models. Microphotographs show TK expression within intracranial GBMs implanted in the rodent brain . Lewis rats bearing intracranial syngeneic CNS -1 GBMs (a) and nude mice bearing intracranial human U251 or U87 GBM (b) were intratumorally injected with Ad vectors encoding thymidine kinase (TK). TK expression was assessed by immunocytochemistry (a) and immunofluorescence (b). Nuclei were stained with DAPI (b, left panels)
2 Materials
2.1 For Intracranial Tumor Implantation
1.
Anesthetics/analgesics and other drugs: ketamine (75 mg/kg body weight, Orion Pharma), Dexdomitor (0.25 mg/kg body weight; Orion Pharma, Espoo, Finland), Rimadyl/carprofen (5 mg/kg body weight; Orion Pharma, Espoo, Finland), atipamezole (1 mg/kg body weight; Orion Pharma, Espoo, Finland), buprenorphine (20 μl/g body weight; MWI Veterinary Supply, Boise, ID, USA), povidone–iodine solution, Paralube eye ointment, saline solution (0.9 % sodium chloride).
2.
Surgical equipment: Stereotactic frame with adaptor for rats and mice and blunt ear bars (Stoelting, Wood Dale, IL, USA), Zeiss Stemi 1000 Zoom stereomicroscope (Zeiss, Jena, Germany) or equivalent, equipped with 16× eye-pieces and 0.4× auxiliary objective lens and mounted on hinged coupling arm and a heavy foot stand, surgical lamp, electric drill with 0.6 mm drill bit for mice and 1.75 mm drill bit for rats (Stoelting, Wood Dale, IL, USA), surgical shavers, scalpel and blades, skin retractors, ethanol swabs, curved and straight forceps, holding scissors, sharp scissors, 3-0 nylon sutures, 5 μl Hamilton syringe with 26G needle for rats and 33G needle for mice.
2.2 For Adenoviral Gene Therapy
1.
Anesthetics/analgesics and other drugs: as above.
2.
Surgical equipment: as above.
3.
Adenoviral vectors: Detailed methodologies to clone, purify, and quantitate adenoviral vectors for gene therapy are detailed elsewhere [16, 23] (see chapter 9). Also included in these references are protocols for the analysis of transgene expression using immunohistochemistry (Fig. 3), enzymatic assays, and flow cytometry. Protocols for the detection of anti-adenoviral immune responses are also described in this chapter. The adenoviral vectors used in this protocol are first generation Ad.hCMV.hsFLT3L + Ad.hCMV.TK.
4.
Phosphate buffered saline: Dulbecco’s PBS, pH 7.4, without calcium or magnesium (Life Technologies, Carlsbad, CA, USA).
5.
Ganciclovir: To prepare 7 mg/ml stock solution, weigh 250 mg of Ganciclovir (Biotang USA, Lexington, MA, USA) and add 20 ml of Milli-Q H2O to it. Adjust pH to 12 with 1 M NaOH. The solution will clear once the pH reaches 12. Next lower the pH to 11 using 1 N HCl and add Milli-Q H2O to a final volume of 35.71 ml. Dilute further with saline for injections.
2.3 For Functional Assays Post Gene Therapy Administration
1.
Tyrodes solution: 132 mM NaCl, 1.8 mM CaCl2·H2O, 0.32 mM NaH2PO4, 5.56 mM glucose, 11.6 mM NaHCO3, and 2.68 mM KCl. To prepare 1 L add 8 g NaCl, 0.264 g CaCl2·H2O, 0.05 g NaH2PO4, 1 g glucose, 1 g NaHCO3, and 0.2 g of KCl. Add 100 μl of heparin to prevent blood from clotting while perfusing.
2.
ACK buffer: Add 8.29 g NH4Cl, 1 g KHCO3, 37.2 mg Na2EDTA to 800 ml of pure H2O. Adjust pH to 7.4 with 1 N HCl and top up the volume to 1 L with H2O.
3.
Complete media: RPMI/DMEM medium supplemented with 10 % FBS, 50 units/ml penicillin and 50 μg/ml streptomycin.
4.
ELISPOT reagents: Mouse IFN-γ ELISPOT Ready-SET-Go kit (eBioscience, San Diego, CA, USA), Immobilon-P 96 well plates, Ethanol (USP grade, mixed 35 % vol/vol in sterile Milli-Q H2O), ELISPOT Wash Buffer (0.01 % Tween 20 in PBS), Cell stimulation cocktail (eBioscience, San Diego, CA, USA), TMB substrate (Mabtech, Cincinnati, OH, USA), ELISPOT plate reader (AID Autoimmun Diagnostika GmbH, Strassberg, Germany).
5.
For T cell proliferation: 5-(and 6)-carboxyfluorescein diacetate succinimidyl ester (CFSE) cell proliferation kit (Life Technologies, Carlsbad, CA, USA), bovine serum albumin, anti-mouse and anti-rat CD3, CD4 and CD8 fluorochrome conjugated antibodies for flow staining, flow staining buffer (2 % FBS in PBS).
3 Methods
3.1 Intracranial GBM Implantation
1.
Resuspend glioma cell lines in serum free media at the required concentration (Injectable volume 1–5 μl).
2.
The surgical area needs to be clean and organized with all the required instruments, drugs and sterile tools (see Note 1 ).
3.
Place the animal under anesthesia by intraperitoneal (i.p.) injection of ketamine and Dexdomitor. Once the animal has lost the footpad reflex it is sedated enough to start the surgical procedure. Administer a subcutaneous injection of Carprofen to ensure analgesia during and after surgery (see Note 2 ).
4.
Shave the fur from the head of the animal.
5.
Mount the animal onto the stereotactic frame, immobilize its head in the incisor bar and tighten it gently with the ear bars and the nose clamp (see Note 3 ).
6.
Clean the incision area thoroughly with alcohol wipes and povidone–iodine solution.
7.
Make a ~1 cm (for mice) or ~1.5 cm (for rats) midline incision along the top of the head between the eyes and the ears. Using a mouse or rat skin retractor hold back the skin on both sides of the incision.
8.
Direct the light beams onto the exposed skull and focus the microscope on the bregma, the junction of the sagittal and transverse sutures (see Note 4 ).
9.
Position the Hamilton syringe using the manipulator arms so that the tip of the needle is exactly over bregma. To inject into the striatum of a mouse or rat, move the manipulator arm forward x mm for the anteroposterior coordinate and then y mm lateral away from the bregma. (Refer to Table 1 for the x and y coordinates for the various cell lines.) Watching through the microscope eyepiece, use a bent 26G1/2 needle to itch a small mark in the skull where the needle will penetrate. Lift the needle so that it does not obstruct the area.
Table 1
Cell numbers, implantation coordinates, and survival duration for various GBM model s are shown
Cell line | Host | Cell number for implantation | Injection coordinates | Median survival |
---|---|---|---|---|
GL261 | C57BL/6 | 2 × 104 | +0.5 mm AP, +2.2 mm ML from bregma and −3.2 mm DV from dura (1 μl) | 28–35 days |
GL26 | C57BL/6 | 2 × 104 | +0.5 mm AP, +2.1 mm ML from bregma and −3.2 mm DV from dura (1 μl) | 26–32 days |
SMA560 | VM/Dk | 5 × 103 | +0.5 mm AP, +2.1 mm ML from bregma and −3.2 mm DV from dura (1 μl) | 30 days |
CNS1 | Lewis rat | 5 × 104 | +1.0 mm AP, +3.0 mm ML from bregma and −5.5, −5.0, and −4.5 mm DV from dura (1 μl per site) | 13–19 days |
9 L | Fisher rat | 5 × 105 | +1.0 mm AP, +3.2 mm ML from bregma and −5.5, −5.0 and −4.5 mm DV from dura (1 μl per site) | 28.1 ±1.3 days |
F98 | Fisher rat | 5 × 104 | +1.0 mm AP, +3.2 mm ML from bregma and −6.5, −5.5 and −4.5 mm DV from the dura (1 μl per site) | 29 days |
RG2 | Fisher rat | 2 × 104 | +1.0 mm AP, +3.0 mm ML from bregma and +6.0 mm DV from dura (3 μl)
![]() Stay updated, free articles. Join our Telegram channel![]() Full access? Get Clinical Tree![]() ![]() ![]() |