Pure cerebellar syndrome
SCA5, SCA6, SCA11, SCA26
Cerebellar ataxia combined with extra-cerebellar deficits (“ataxia plus”)
Cognitive deficits and/or behavioral symptoms
SCA1, SCA2, SCA3, SCA10, SCA12, SCA13, SCA14, SCA17, SCA19, SCA21, SCA27, DRPLA
Seizures
SCA10, SCA17, DRPLA
Oculomotor deficits and/or involvement of eyes
Downbeat nystagmus
SCA6
Ocular dyskinesia
SCA10
Slow saccades
SCA1, SCA2, SCA3, SCA7, SCA28
Ophthalmoplegia
SCA1, SCA2, SCA3, SCA28, SCA30
Retinopathy
SCA7
Pyramidal deficits
SCA1, SCA2, SCA3, SCA4, SCA7, SCA8, SCA10, SCA11, SCA12, SCA13, SCA14, SCA15, SCA28, SCA30
Movement disorders
Dystonia
SCA3, SCA14, SCA17
Parkinsonism
SCA1, SCA2, SCA3, SCA12, SCA17, SCA21
Tremor
SCA8, SCA12, SCA16, SCA19, SCA20
Dyskinesias
SCA27
Chorea
SCA1, SCA17, DRPLA
Myoclonic jerks
SCA2, SCA14, SCA19, DRPLA
Peripheral neuropathy
SCA1, SCA2, SCA3, SCA4, SCA6, SCA8, SCA27, SCA12, SCA18, SCA22, SCA25
Establishing the Diagnosis of Dominant Ataxias
Establishing the diagnosis of hereditary ataxia requires:
1.
A detailed neurological examination and detection of typical clinical signs including:
(a)
Poorly coordinated gait and finger/hand movements
(b)
Dysarthria (incoordination of speech)
(c)
Eye movement abnormalities such as nystagmus, abnormal ocular saccades, and ophthalmoplegia
2.
Brain imaging studies (especially MRI studies)
3.
Documentation of the hereditary nature of the disease:
(a)
Positive family history of ataxia
(b)
Identification of an ataxia-causing mutation
(c)
To recognize a clinical phenotype characteristic of a genetic form of ataxia
4.
Exclusion of nongenetic causes of ataxia.
Diagnosis
The diagnostic thus relies on the family history, the clinical presentation, the neuroimaging pattern, and the genetic tests. Overall, the genetic tests are currently successful in about 60 % of SCAs (see below). Time should be devoted to construct the family tree in order to determine the mode of inheritance. Families with a history of a predominant cerebellar syndrome during successive generations have a high likelihood to present a SCA when both sexes are affected. Anticipation (discussed below) is an additional argument. However, the family tree may be difficult to set when the other members of the family cannot be examined (following death, for instance) or in case of no apparent symptom in some family members (patients at a presymptomatic stage). The variable penetrance and a possible false paternity or adoption also need to be taken into account. The ethnic and geographical background is also a clue for the diagnosis.
Additional tests may be valuable for the differential diagnosis. Neurophysiological studies such as nerve conduction velocities (NCV) and visual-evoked potentials (VEP) are useful to determine the presence of a peripheral neuropathy and optical nerve disease, respectively. Motor-evoked potentials (MEP) confirm the involvement of the corticospinal tract, especially in SCA1. The presence of periodic leg movements (PLM) during sleep is detected by polysomnography.
Brain Imaging
Brain MRI is noninvasive and is considered as a technique of choice to assess the anatomy of the brain in dominant ataxias (Fig. 11.1). It is important to exclude other causes of ataxia which could mimic a degenerative disorder (brain tumor, stroke, malformation, immune disease, etc.). Although brain MRI may be totally normal at the beginning of the symptoms, it becomes a sensitive tool to quantify atrophy as the disorder evolves with time. The main morphological patterns are the following:


Fig. 11.1
Brain imaging in SCAs. (a–c) Brain MRI in a 52-year-old man presenting a SCA2. Atrophy of the medulla, pons, and cerebellum on T2-weighted axial images (a, b). Flattening of the pons on T1-weighted sagittal image (c; see white arrow). Atrophy of the cerebellar cortex on coronal T2-weighted image (black arrow, d) in a 44-year-old man with a SCA2. Atrophy of the vermis (white arrow) and slight flattening of the pons (arrowhead) in a 23-year-old woman presenting a SCA8 (e; T1-weighted sagittal image). The atrophy of the cerebellar cortex is well identified on T2-weighted axial images in this patient (f–g; black arrows). Slight atrophy of the upper vermis in a patient presenting EA2 (h; sagittal T1-weighted image; white arrow)
A pattern of pure cerebellar atrophy (in particular in SCA6).
A pattern of OPCA with atrophy of the cerebellum and brainstem (typical for SCA1, SCA2, SCA3, and SCA7). The atrophy can extend to the upper segments of the spinal cord.
A pattern of global brain atrophy (for instance, in DRPLA).
Some findings may be very suggestive of a given SCA. Dentate nuclei calcifications are observed in SCA20.
Volumetric studies are now available as routine procedures and allow to quantify the degree of regional atrophy and the progression with time.
Differential Diagnosis
Differential diagnosis of hereditary ataxia includes:
Acquired, nongenetic causes of ataxia: alcoholism, vitamin deficiencies, multiple sclerosis, cerebrovascular disease, primary or metastatic tumors, and paraneoplastic diseases associated with occult carcinoma of the ovary, breast, or lung and the idiopathic degenerative disease multiple system atrophy (MSA). An acquired cause of ataxia should be considered in all cases of ataxia.
Molecular Genetic Testing of SCAs
ADCAs are associated to mutations that include nucleotide expansions occurring in either expressed or non-expressed regions of the gene, point mutations, duplications, and deletions. The normal size of CAG repeat allele and of the full-penetrance disease-causing CAG expansion varies among the disorders (for reviews, see GeneReviews.org). Today, 50–60 % of the dominant hereditary ataxias can be identified with accurate and specific molecular genetic testing. This is the case for SCA1, SCA2, SCA3, SCA6, SCA7, SCA8, SCA10, SCA12, SCA17, and DRPLA. These disorders are characterized by CAG repeats within the coding region of the genes, translating into an elongated polyglutamine tract in the protein. Molecular genetic tests for CAG repeat length are highly specific and sensitive diagnostic tools, and further they are commercially available. However, pursuing tests for multiple genes simultaneously may seem less optimal than serial genetic testing, but their cost is decreasing. Molecular genetic testing results more specific than MRI findings in the hereditary ataxias and guidelines for genetic testing of hereditary ataxia have been published [13]. Testing is also available for some autosomal dominant forms of SCA that are not associated with repeat expansions (SCA5, SCA13, SCA14, SCA15, SCA27, SCA28, and 16q22-linked SCA). Interpretation of results can be an issue in the same case and should be done with caution, and the following aspects should be taken into account:
The exact range for the abnormal repeat expansion has not been fully established for many of these disorders.
In some cases, there may be an overlap between the upper range of normal values and the lower range of abnormal CAG repeat size. Such alleles are classified as mutable normal or reduced penetrance. Mutable normal alleles do not cause disease themselves but can expand during the genetic transmission to a reduced or fully penetrant allele. In a few words, children of an individual carrying a mutable normal allele have increased risk of inheriting a disease-causing allele. Therefore, interpretation of results in which the CAG repeat length is at the interface between the allele categories mutable normal/reduced penetrance and reduced penetrance/disease-causing results can be difficult. In such cases, a consultation with the testing laboratory should be considered.
SCA2, SCA7, SCA8, and SCA10 mutations may present with extremely large CAG expansions in length, only detectable by Southern blot analysis. For these disorders, a test of apparent homozygosity (detection of a single allele by PCR analysis) should be associated to the clinical findings, the family history, and the age of onset of symptoms (to determine whether Southern blot analysis is needed).
The Risk for Family Members
Generally autosomal dominant ataxia patients have an affected parent, although in some cases the family history is negative. For example, family history may not be obvious because of:
An early death of a parent or a late-onset disorder
A failure to recognize autosomal dominant ataxia in family members
A reduced penetrance of the mutant allele in an asymptomatic parent or a de novo mutation
The risk to siblings depends on the genetic status of the proband’s parents. If one of the proband’s parents has a mutant allele, the risk for the sibs of inheriting the mutant allele is 50 %. Individuals with autosomal dominant ataxia have a 50 % chance of transmitting the mutant allele to each child.
Related Genetic Counseling Issues
At-risk asymptomatic adult can be tested for autosomal dominant cerebellar ataxia after identification of a specific disorder and mutation in their family. Such testing should be performed in the context of formal genetic counseling and will remain a predictive testing and not a diagnostic testing. The test will not predict the age of onset, the severity, the type of symptoms, or the rate of progression. However, molecular genetic testing of asymptomatic individuals younger than 18 years who are at risk for adult-onset disorders for which no effective treatment exists is not considered appropriate. Concern exists regarding the potential unhealthy adverse effects that such information may have on family dynamics, the risk of discrimination and stigmatization in the future, and the anxiety that such information may cause. For more information, see the recommendations of the National Society of Genetic Counselors resolution on genetic testing of children and the American Society of Human Genetics and American College of Medical Genetics points.
New Genetic Technologies
Looking for the exact genetic mutation in patients with a cerebellar ataxia may be difficult, time consuming, and costly. The relatively uniform phenotype of many patients with cerebellar ataxia makes it difficult to decide which gene should be investigated first. Since conventional testing is expensive, many genes that are rarely involved in disease causation may not be tested. Thus, the development of novel technologies such as exome capture and next-generation DNA sequencing (NGS) is very interesting to screen the whole coding part of the genome in one experiment at reduced costs. This has been demonstrated to be a powerful diagnostic approach in the case of ataxia and Charcot–Marie–Tooth disease, which is a similarly heterogeneous disease [14]. The costs for this test decreased dramatically over the last year (it is about 1,000 USD). However, limitations need to be considered, in particular in the diagnosis of ataxia. In fact, currently NGS has a poor ability to sequence stretches of repetitive DNA such as the polyglutamine repeats.
Recently, systems biology approach based on whole-transcriptome gene expression analysis has been used to address the possible relationships among known SCA genes, predict their functions, identify overlapping pathways, and provide a framework for candidate gene discovery. Published results showed that two cerebellar gene coexpression modules were statistically enriched in SCA transcripts and contained established granule and Purkinje cell markers, respectively. One module includes genes involved in the ubiquitin–proteasome system and contains SCA genes usually associated with a complex phenotype, while the other module encloses many genes important for calcium homeostasis and signaling and contains SCA genes associated mostly with pure ataxia [15].
DNA Banking
DNA banking is the storage of DNA (generally from white blood cells) for possible future use. Because it is likely that testing methodology will improve in the future, consideration should be given to banking DNA of affected individuals with dominant ataxias.
Prenatal Testing
Prenatal diagnosis for some of the hereditary ataxias is possible by analyzing fetal DNA (from chorionic villus sampling at about 10–12 weeks’ gestation or amniocentesis, usually performed at about 15–18 weeks’ gestation) for disease-causing mutations. The disease-causing allele(s) of an affected family member must be identified before prenatal testing. Although most centers would consider decisions about prenatal testing to be the choice of the parents, discussion regarding the purpose of the testing should be considered, like pregnancy termination rather than early diagnosis. Preimplantation genetic diagnosis may also be available when the disease-causing mutation has been identified.
Genetics of SCAs and DRPLA
Historically, SCA1 was linked to chromosome 6 in 1977. SCA1 was identified as the first disorder associated with a trinucleotide repeat expansion [16]. Current numbering of SCAs is based on the chronological order of the gene discovery.
Table 11.2 lists the SCAs and their respective locus, and Table 11.3 provides the normal repeat range, the intermediate repeat range, and the pathological repeat range. A number of 32 genetic loci have been discovered:
Table 11.2
Loci of the SCAs
Disease | Chromosomal locus (mutation) | MIM number |
---|---|---|
SCA1 | 6p23 (CAG expansion) | 164400 |
SCA2 | 12q24.1 (CAG expansion) | 183090 |
SCA3 | 14q24.3-q31 (CAG expansion) | 607407 |
SCA4 | 16q22.1 | 600223 |
SCA5 | 11q13 (point mutations, in-frame deletions) | 600224 |
SCA6 | 19p13 (CAG expansion) | 601011 |
SCA7 | 3p14-p21.1 (CAG expansion) | 164500 |
SCA8 | 13q21 (CTG–CAG expansion) | 608768 |
SCA10 | 22q13 (ATTCT expansion) | 603516 |
SCA11 | 15q14-21.3 (point mutations, insertions, deletions) | 604432 |
SCA12 | 5q31-q33 (CAG expansion) | 604326 |
SCA13 | 19q13.3-q13.4 (point mutations) | 605259 |
SCA14 | 19q13.4-qter (point mutations) | 605361 |
SCA15 | 3p26-p25 (point mutations, deletions) | 606658 |
SCA17 | 6q27 (CAG expansion) | 607136 |
SCA18 | 7q22-q32 | 607458 |
SCA19 | 1p21-q21 | 607346 |
SCA20 | 11p13-q11 (duplication) | 608687 |
SCA21 | 7p21.3-p15.1 | 607454 |
SCA22 | 1p21-q23 | 607346 |
SCA23 | 20p13-12.3 (point mutations) | 610245 |
SCA25 | 2p15-p21 | 608703 |
SCA26 | 19p13.3 | 609306 |
SCA27 (FGF14) | 13q34 (point mutations) | 609307 |
SCA28 | 18p11.22-q11.2 (point mutations) | 610246 |
SCA29 | 3p26 | 117360 |
SCA30 | 4q34-q35 | 613371 |
SCA31 | 16q22.1 (TGGAAn repeat) | 117210 |
SCA32 | 7q32-q33 | 613909 |
SCA35 | 20p13 (point mutations) | 613908 |
SCA36 | 20p13 (point mutations) | 614153 |
DRPLA | 12p13.31 (CAG expansion) | 125370 |
Table 11.3
Ranges of repeats in SCAs
Disease | Normal range | Intermediate range | Pathological range |
---|---|---|---|
SCA1 | 6–35 | 35–39 | 39–83 |
SCA2 | 14–31 | 31–34 | 34–77 |
SCA3 | 12–44 | 44–52 | 52–86 |
SCA6 | 4–18 | 18–20 | 20–33 |
SCA7 | 7–19 | 19–37 | 37 to >400 |
SCA8 | 15–50 | 50–80 | 80 to >1,300 |
SCA10 | 10–29 | 280–800 | 800–4,500 |
SCA12 | 4–32 | 32–51 | 51–78 |
SCA17 | 25–42 | 42–49 | 49–66 |
SCA31 | 1.2–2.0 kb | 2.5–3.8 kb | |
SCA36 | 3–8 | 1,500–2,500 | |
DRPLA | 3–35 | 35–48 | 48–93 |
SCA1 to SCA8.
SCA9 is not attributed.
SCA10 to SCA15/16 (SCA15 and SCA16 are identical).
SCA17 to SCA23 (SCA22 is an allelic variant of SCA19).
SCA24 is not attributed.
SCA25 to SCA28.
SCA29 is an allelic variant of SCA15.
SCA30 to SCA32.
SCA35 to SCA36.
DRPLA.
On the basis of the genetic mutation, three groups of SCAs can be considered (Fig. 11.2).


Fig. 11.2
Mutations in SCAs. NCRE noncoding repeat expansions, CRE coding repeat expansions, CM conventional mutations (missense, in-frame deletions, frameshift, nonsense, duplication)
Due to Repeat Expansion
This group is characterized by a CAG repeat expansion within the coding or noncoding parts of the relevant genes [18]. This includes SCA1, SCA2, SCA3, SCA6, SCA7, SCA17, and DRPLA. Because the CAG codon codes for glutamine (Q), the mutation results in the production of mutant protein characterized by a polyQ (polyglutamine, hence the terminology of polyglutaminopathies). Genotype–phenotype correlations of these disorders are well described [3] with the disease manifesting above a threshold of CAG repeats. The proteins are named ataxin-1 (ATXN1) for SCA1, ATXN2 for SCA2, ATXN3 for SCA3, ATXN7 for SCA7, and atrophin-1 for DRPLA. In SCA6, the mutation involves the calcium channel CACNA1A and in SCA17 the TATA-binding protein (TBP). Three important features are found in this group: (a) there is an inverse relationship between the number of CAG repeats and the age of onset of the clinical deficits, (b) the age of onset is characterized by anticipation, (c) and above a certain threshold in terms of number of CAG repeats, a full penetrance of the disorder occurs. It should be noted that CAG expansions are also found in two disorders usually not classified within ataxias: spinobulbar muscular atrophy (SBMA) and Huntington’s disease (HD).
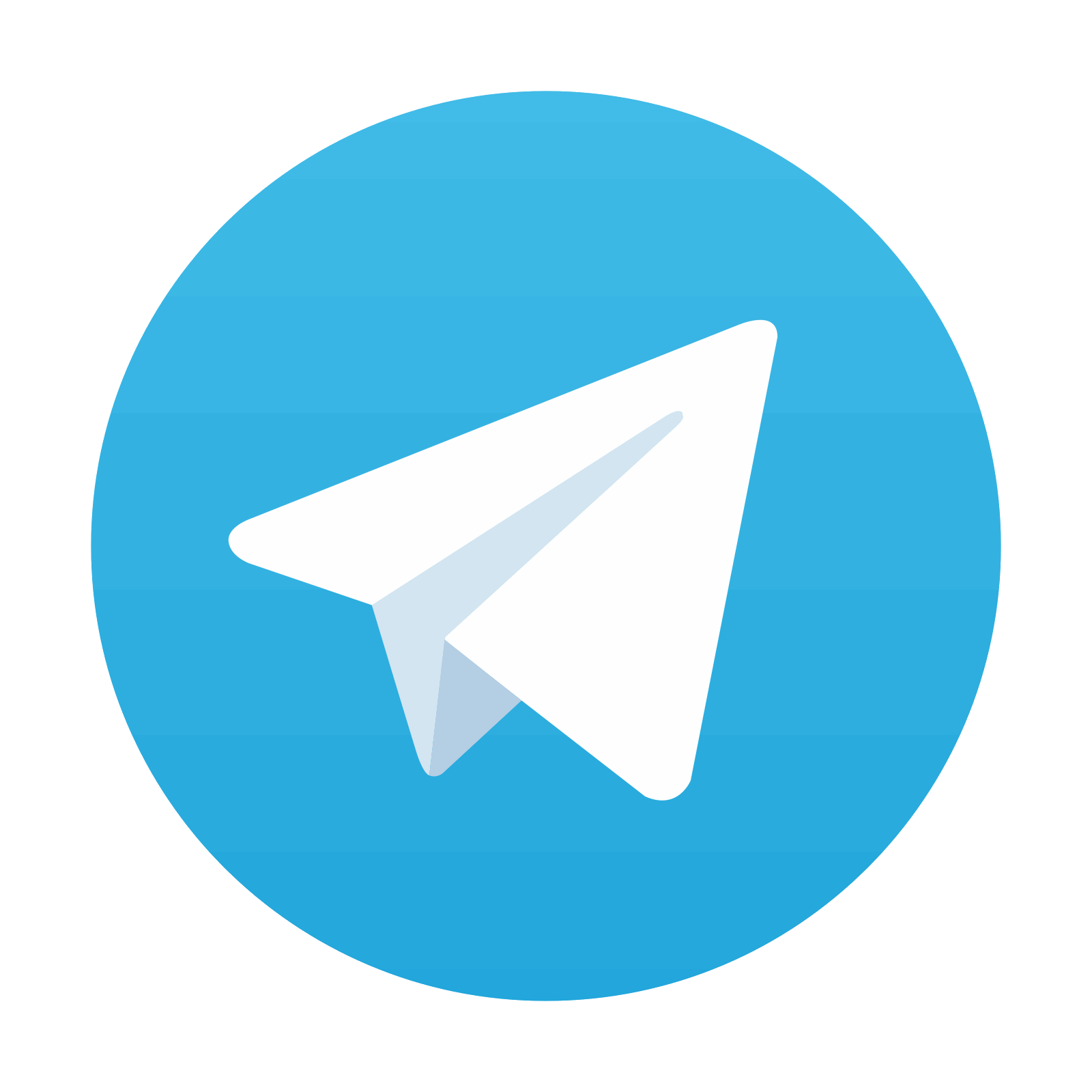
Stay updated, free articles. Join our Telegram channel

Full access? Get Clinical Tree
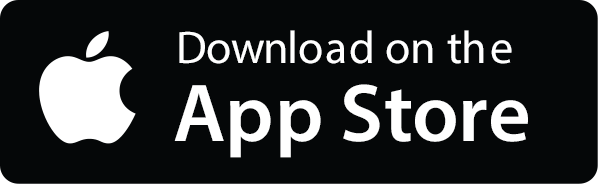
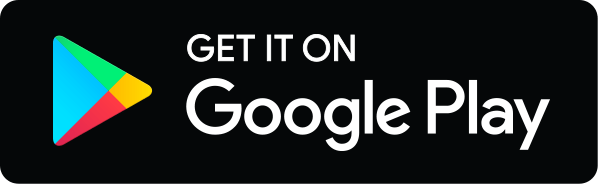