Figure 18.1. Interictal 4- to 6-Hz polyspike-and-wave discharge of frontocentral predominance in JME. No clinical changes were seen, and the patient could recall a word given during the discharge.
Genetics
The genetic nature of JME has been well established and is likely complex and polygenic in most patients, though some rare monogenic forms are being identified. About 40% of patients with JME report a positive family history for epilepsy (56). Genetics studies to date suggest that JME is a heterogeneous condition with multiple different mutations and susceptibility loci that have different modes of inheritance and possible mechanisms of action (21). Autosomal recessive, autosomal dominant, and complex polygenic models have been proposed in different family pedigrees (21). Analysis of JME families has identified linkages to multiple chromosomal loci that may contain genes that are causative or increase the susceptibility for developing JME. Chromosomal locations that demonstrate increased susceptibility in JME families include 6p12-p11 (EFHC1), 6p21 (BRD2/RING3), 15q13-14 (Cx-36), 5q34 (GABRA1), 2q22-23 (CACNB4), 1p36.33 (GABRD), 3q21.1 (CASR), 18q21 (ME2), 19q13 (SCN1B), and Xp11.4-11.3 (EFHC2) (20,21,57–60). Several studies have also identified mutations that are rare but appear to be causative in selected families. Mutations have been identified in EFHC1/Myoclonin1, CLCN2, GABRA1, and CACNB4 (57). In addition, single nucleotide polymorphism susceptibility alleles have been identified in the bromodomain- containing protein 2 (BRD2) and connexin 36 (Cx-36) (61–63).
EFHC1/Myoclonin1
Studies of multiple families of JME have implicated the 6p12-p11 region as a susceptibility focus, and the potential gene was referred to as EJM1. Mutational analysis was able to narrow this locus to the candidate gene EFHC1, which encodes for the protein Myoclonin1 (64). Proposed mechanisms of action for EFHC1/Myoclonin1 include promoting apoptosis, regulating R-type calcium currents, cell cycle regulation, and neuronal migration (64–66). Animal studies have shown that mice deficient in EFHC1 have spontaneous myoclonus and increased seizure susceptibility (67). Mutations of EFHC1/Myoclonin1 have been reported in 9% of JME patients in Mexico and Honduras and 3% of JME patients in Japan, which is more frequent than any other identified mutations in JME thus far (66).
CLCN2
Mutations of the CLCN2 chloride channels were found in three families of JME patients (37). The proposed mechanism was a reduction in chloride channel activity and increased neuronal excitability. The mutations were detected in patients with JME and also some apparently unaffected family members as well but not in a control population (68). The authors concluded that CLCN2 mutations may act as susceptibility factors for epilepsy among other unknown genetic alterations (68).
GABRA1
Studies of a large French–Canadian family with JME led to the discovery of a mutation in the α1 subunit of the γ-aminobutyric acid receptor subtype A (GABRA1). Studies in vitro suggested a loss of gamma-aminobutyric acid (GABA)-activated currents in receptors containing the mutant subunit (69). Expression of the mutant protein revealed a misfolded protein with impaired insertion into the plasma membrane (70). The proposed mechanism of action is a loss of inhibitory signals due to the defective GABA receptors and subsequent increased cortical excitability (69).
CACNB4
A female patient diagnosed with JME was found to have a mutation in the calcium channel β4 subunit (CACNB4) and her daughter with the same mutation had epilepsy with 3-Hz spike-and-wave complexes (71). A father and son with absence and GTC seizures have also been identified. The proposed mode of inheritance is autosomal dominant. This mutation is thought to impair the channel function by shifting the voltage dependence of activation and inactivation. Additional mutations of the same subunit were associated with epilepsy and episodic ataxia (71).
It should be noted that although progress in identifying a few of the genes involved in JME is beginning to emerge, the vast majority of genes and combinations of genes that are involved are yet to be discovered (20,21). While the clinical presentation in JME is fairly homogenous, the pathogenesis appears to be complex and diverse (41).
Treatment
Appropriate management of a patient with JME requires consideration of multiple factors including AED selection, avoiding precipitating factors, and anticipating special considerations that might impact their care (pregnancy, driving, behavioral issues). Response to medical therapy is generally good, with 60% to 80% seizure-free rate on medications. However, noncompliance and lifestyle choices that involve sleep deprivation, alcohol use, and other precipitants often lead to breakthrough seizures. AED selection is also critical as some AEDs can exacerbate JME and lead to a pseudointractable state (26,32). Quality of life dramatically improves if a patient is able to reach seizure freedom, which can lead to driving, employment, and a higher likelihood of social success. In addition to an appropriate AED choice, patients should be counseled to obtain adequate sleep, avoid alcohol, and wear polarized sunglasses if their seizures are sensitive to photic stimulation.
Valproate
Valproic acid is the treatment of choice for JME as it effectively treats absence, myoclonic, and GTC seizures in 86% to 90% of patients (72,73). A recent large, unblinded, randomized, controlled study of patients with IGE treated with Standard and New Antiepileptic Drugs (SANAD) suggested that valproate was preferred to lamotrigine due to better control of seizures and preferred over topiramate due to lower rates of discontinuation due to side effects (74). Valproate is associated with a number of side effects that can sometimes limit its use or warrant reconsideration, including weight gain, hair loss, tremor, and teratogenicity. Weight gain is a frequent reason for discontinuing valproate, with 50% to 70% of patients gaining more than 4 kg (75). The standard dosing of valproate is 20 to 30 mg/kg/day, but patients with JME often respond to low doses, such as 500 mg/day (76). Valproate at low doses (below 1000 mg/day) and with extended-release preparations has significantly less side effects and is often well tolerated.
Lamotrigine
Lamotrigine is a useful alternative for patients with JME, especially when valproate alone is not effective or not well tolerated. In a retrospective cohort study, no difference in seizure control was found between valproate monotherapy and lamotrigine monotherapy, and the authors concluded that lamotrigine was an acceptable alternative to valproate (77). When compared in a randomized, controlled trial (SANAD), valproate had better efficacy in terms of seizure control than lamotrigine (74). There have also been some reports of exacerbation of myoclonic seizures with lamotrigine (78). In a study using lamotrigine as an add-on agent in treatment-resistant generalized epilepsy, 80% of patients had a >50% reduction in seizure frequency and 25% became seizure free (75). In an open-label study of lamotrigine monotherapy in patients who had failed valproate, there was no significant change in their underlying seizures, but 67% of patients reported improvement in their global clinical status on lamotrigine and 76% of patients rated lamotrigine as better tolerated than valproate (79). The major side effect of lamotrigine reported was a rash, which in some patients can be severe. An allergic skin reaction occurs in approximately 10% of patients with severe rash (such as Steven–Johnson syndrome) occurring in 0.3% of adults and 1% of children (80). The lamotrigine-associated rash may be more severe when combined with valproic acid (80). Lamotrigine is well tolerated and effective as an adjunctive therapy for JME, but concerns over decreased seizure control compared to valproate and exacerbation of myoclonic seizures in some patients limit its use as a monotherapy.
Topiramate
Topiramate is another possible adjunctive or alternative therapy to valproate, but there are limited data on application in patients with JME. In double-blind, placebo-controlled studies of topiramate, 73% of patients in the JME subgroup had a >50% reduction in primary GTC seizures and 16% became seizure free (81). In a randomized open-label comparison, topiramate and valproate had similar rates of seizure freedom (67% and 57%, respectively), and in both treatment groups, 11% of patients stopped the medication due to adverse effects (82). In the SANAD study, topiramate had similar efficacy to valproate but was associated with more side effects (74). Typical side effects with topiramate include cognitive dysfunction, anomia, weight loss, and nephrolithiasis.
Levetiracetam
A number of studies have shown the efficacy of levetiracetam as an adjunctive therapy for IGE, and its features of no known drug interactions and overall good tolerability have made this drug a useful asset. Using a retrospective design, Kumar (83) reported 25 IGE patients treated with levetiracetam and found that 68% of patients had some improvement in their seizures and 16% became seizure free. In an open-label study, Kraus et al. (84) studied 55 patients with GTC, myoclonic, and absence seizures and found that 76% had a >50% reduction in seizures and 40% became seizure free. These authors also found that 15% of patients discontinued levetiracetam due to adverse events (with sedation being the most common). In a multicenter, double-blind, placebo-controlled study, Berkovic et al. examined 164 patients with IGE. They reported that 72.2% of patients had a >50% reduction in seizures, with 34% of patients seizure free from GTC seizures and 24% seizure free from all seizure types (85). Levetiracetam was well tolerated in this study, and only 1.3% of patients discontinued therapy due to adverse events. A randomized, double-blind, placebo-controlled, multicenter trial by Noachtar et al. addressed the effects of levetiracetam on myoclonic seizures (86). They examined 120 patients and found that 58.3% of patients with myoclonic seizures had a >50% seizure reduction within days/week. The rate of myoclonic seizure freedom was 25%, and seizure freedom from all seizure types was seen in 21%. Levetiracetam has been shown to be efficacious as an adjunctive therapy in JME for all seizure types including myoclonic seizures. Adverse events of somnolence, headache, and irritability are relatively rare in 1% to 15% of patients.
Zonisamide
There have been relatively few studies on the efficacy of zonisamide in IGE and JME, but initial data have shown efficacy as an adjunctive agent for multiple seizure types, including JME. Zonisamide has been available in Japan since 1989, and much of the data derive from the Japanese experience. In a prospective postmarketing survey, Yamauchi et al. (87) reported that 78% of patients with IGE and 50% of patients with myoclonic seizures had a >50% seizure reduction with zonisamide. Yagi reported on pooled zonisamide efficacy data of 1008 patients collected from controlled and uncontrolled phase II and phase III studies and found 59% of GTC seizures, 62% of absence seizures, and 43% of myoclonic seizures were reduced by >50% (88). In a small retrospective open-label study, Kothare et al. reported that 80% of patients with JME had a >50% reduction in seizures on zonisamide monotherapy (89). They also reported seizure freedom in 69% of GTC seizures, 62% of myoclonic seizures, and 38% of absence seizures. Preliminary data suggest that zonisamide is efficacious as an adjunctive medication for JME, but further data from randomized, controlled trials will help delineate this. The advantages of zonisamide are once-daily dosing. Common side effects include weight loss, cognitive problems, and nephrolithiasis.
A number of medications have been reported to exacerbate JME, especially myoclonic seizures (26). The most common medications causing seizure aggravation are carbamazepine and oxcarbazepine. Phenytoin, gabapentin, and vigabatrin may also exacerbate seizures in JME or do not have efficacy.
Special Considerations of Treating JME in Pregnancy
Childbearing-age women represent 25% of patients treated for epilepsy, and the possibility of pregnancy often influences AED selection (90). While all of the AEDs have some risk of teratogenicity, valproate has the highest risk, especially when given in doses >1000 mg/day (6% to 11% chance of birth defects) (91). This is balanced by the evidence that valproate is the clinically most effective medication to prevent seizures based on the SANAD study (74). Lamotrigine has been the best studied of the newer medications in pregnancy, has been followed in multiple pregnancy registries, and has a reported 2.7% risk of major congenital malformations (92,93). However, the actual risk of birth defects with lamotrigine is unclear since pregnancy induces the clearance of this drug by up to 94%, and this decrease in drug levels is not accounted for in the pregnancy registries (94). There has also been increased seizure frequency in mothers taking lamotrigine during pregnancy (94). There has been some indication of a dose-responsive risk of birth defects with lamotrigine, with an increased malformation rate in patients taking more than 200 mg/day, reported in the UK pregnancy registry but not in the International Lamotrigine Pregnancy registry (92,93). There is little information on other medications in JME during pregnancy such as topiramate, zonisamide, levetiracetam, and clobazam. Monotherapy in general had less risk of malformations (3.7%) than does polytherapy (6.0%) regardless of AEDs involved (93). There is some evidence that exposure to AEDs during pregnancy can affect the cognitive development of the fetus, with valproate associated with worse cognitive outcomes (91,95). In the Neurodevelopmental Effects of Antiepileptic Drugs study, children exposed to valproate had a lower intelligence quotient on average by 9 points compared to patients exposed to lamotrigine (95). The effect of valproate was dose dependent and was not observed at dosages below 800 mg/day.
A cautious and informed approach is needed when treating female patients in their childbearing years. Valproate is generally considered the most efficacious drug at suppressing the seizures in JME and is a reasonable choice if doses of <800 to 1000 mg/day can be used. Lamotrigine has shown fewer incidences of birth defects and potential cognitive problems but has fewer efficacies in preventing maternal seizures, and increased seizure frequency has been reported (94), in particular exacerbation of myoclonic seizures. Young women with JME should not be discouraged from having children in general, as >90% of women have normal pregnancies. However, placement on monotherapy and preconceptual planning should be encouraged to reduce the risk of fetal malformations and potential cognitive delays.
Prognosis
The prognosis for JME is generally considered excellent as the majority of patients can be treated successfully with AEDs. Seizure-freedom rates of 60% to 90% have been reported. It has been noted that while response to medication is good, patients tend to have breakthrough seizures due to noncompliance, sleep deprivation, alcohol use, or other precipitants. Achieving seizure freedom often involves lifestyle modifications as well as compliance with AEDs. Multiple studies in the past have suggested that JME is often a lifelong condition (96). Delgado-Escueta and Enrile-Bacsal have reported a 90% relapse rate with withdrawal of medications in the past. However, a more recent longitudinal study followed patients with JME for 25 years and found that 48% had voluntarily stopped their medications, 17% were without seizures on no medication, and 13% had myoclonus only, also without medication (1). It should also be noted that 36% of patients followed in the study had an episode of convulsive status epilepticus and 13% had medically intractable seizures. While a small percentage of patients with JME could likely come off medications at some time interval as suggested in the study, determining who will remain seizure free and who will continue to have seizures is less clear.
EPILEPSY WITH GENERALIZED TONIC–CLONIC SEIZURES ONLY
Epilepsy with GTC seizures only has recently been described by the ILAE in their most recent proposed diagnostic scheme for people with epileptic seizures and epilepsy as a separate syndrome (3). It includes “epilepsy with GTC seizures on awakening,” which was previously described as a separate syndrome. The diagnosis of this syndrome can be challenging as many IGEs as well as focal epilepsies have some component of GTC seizures.
Epidemiology
There is limited information on the epidemiology of patients presenting with GTC seizures only. In a population-based study, epilepsy with grand mal upon awakening was reported as a major feature in 23% of generalized epilepsies (97). A population-based study in France reported an incidence of 1.8 per 100,000 people (97).
Clinical Features
The peak age of onset is at 15 with an age range between 5 and 50 years (7). Neurologic exam and imaging is normal, and other tests except for the EEG do not reveal any other neurologic abnormalities.
The predominant seizure type is GTC. Absence seizures and myoclonic seizures are seen less frequently. Seizures are mainly provoked by alcohol and sleep deprivation and can also be brought out by photic stimulation. Seizures tend to worsen with age. Wolf reported GTC seizures on awakening in 17% to 53%, during wakefulness in 23% to 36%, during sleep in 27% to 44%, and not related to wake or sleep patterns in 13% to 26% of patients (98). In the subcategory of GTC seizures on awakening, GTC seizures occur in more than 90% within 1 to 2 hours after awakening or at the end of the day (during relaxation), with only rare myoclonic or dialeptic seizures. Unterberger et al. compared epilepsy with GTC seizures on awakening and randomly occurring GTC seizures and found that patients with early morning seizures had a longer duration of epilepsy, a higher relapse rate, and a stronger relationship to seizure provoking factors (99).
EEG
Interictal features on EEG consist of generalized epileptiform discharges presenting either as generalized 4- to 5-Hz spike-and-wave complexes or as generalized polyspikes. Discharges can be seen bilaterally with occasional asynchrony or asymmetry of bursts.
Ictal EEG is characterized by generalized fast rhythmic spiking, with a bifrontal maximum during the tonic phase of a GTC seizure. EEG activity is frequently obscured by tonic or clonic muscle artifact. Spiking can be asymmetric and asynchronous. This activity slows down and evolves into discontinuous repetitive generalized bursts of generalized (poly) spikes and waves intermingled with rhythmic slow waves. Clonic jerks start approximately at a spike frequency of 4 Hz. Postictally, electrical activity is reduced and can occasionally appear silent (<10 μV) for a brief period and is usually followed by irregular diffuse slowing.
Genetics
Patients with epilepsy with GTC seizures only frequently have a positive family history of epilepsy. Most recently, different mutations in unrelated families of the chloride channel-2 gene (CLCN2) on chromosome 3q26-qter have been found to be associated with GTC seizures on awakening (37). Interestingly, CAE, JAE, and JME were also found in families with this mutation (37).
Treatment
Monotherapy with lamotrigine or valproate is recommended, with valproate having higher efficacy and lamotrigine fewer side effects (74). Other options include topiramate, levetiracetam, and zonisamide. If the maximum tolerated dose does not reduce seizure frequency, an alternative medication should be tried. In case of monotherapy failure, combination therapy of lamotrigine and valproate may be effective (100). Gabapentin is not helpful, and tiagabine and vigabatrin may exacerbate seizures in some cases (26). Additionally, the prevention of precipitating factors of seizures such as sleep deprivation and alcohol intake drug treatment is beneficial.
Prognosis
The prognosis of patients with GTC seizures only is very good and usually better than in patients with focal epilepsy and secondary generalized seizures (101). Up to 95% of patients with GTC seizures of unknown origin will have a continuous 5-year seizure-free period within 20 years after epilepsy onset (101). Twenty-one percent of patients who have been seizure free for 5 years or longer relapse within a 20-year observation period (101). Frequency of GTC seizure at the time of diagnosis predicts outcome and remission (102). Failure to remit within 2 years of diagnosis reduces the chance of remission in the following years (103).
GENERALIZED EPILEPSY WITH FEBRILE SEIZURES PLUS (GEFS+)
Generalized epilepsy with febrile seizures plus (GEFS+) is a heterogeneous disorder with some features of an IGE. Generalized epilepsy seizure types as well as febrile seizures, focal seizures, and progressive epilepsy syndromes such as Dravet syndrome have been described (104,105). There is an underlying genetic basis for this disorder with four genes identified thus far, but the clinical features of GEFS+ are divergent from typical IGE in some patients. GEFS+ illustrates the complexity of epilepsy syndromes with multiple different phenotypes arising from the same mutation and different mutations giving rise to clinically similar phenotypes, all-in-one syndrome.
Epidemiology
The epidemiology of GEFS+ has not been studied in a large epidemiologic study, and the incidence/prevalence is unknown. It has been speculated that GEFS+ is a common childhood syndrome, and detailing this will be a challenge given the clinical heterogeneity that has been attributed to this syndrome thus far (106).
Clinical Features
Most patients with GEFS+ present with febrile seizures in the typical ages between 3 months and 6 years, then continue to either have additional febrile seizures outside of this age range or begin having afebrile seizures as well (104,105). Seizures can persist into late adolescence or longer and may remit in the early teenage years. A history of febrile seizures in other family members is crucial to the diagnosis.
Febrile seizures begin approximately at the age of 1 year, slightly earlier than in the average infant with febrile seizures. Onset of afebrile seizures may overlap with febrile seizures or may occur after a seizure-free interval between febrile and afebrile seizures.
Neurologic exam is normal in the majority of patients described but may also show cognitive impairment and developmental abnormalities (104,106,107).
Clinical seizures consist of febrile seizures in association with afebrile seizures presenting as GTC seizures or dialeptic seizures (absences). Other seizure types of myoclonic–astatic, atonic, tonic, and complex partial seizures have also been described (104,106,107). Seizures usually persist beyond 6 years of age until adolescence or longer.
EEG
The syndrome lacks a clear electroclinical pattern, and interictal EEG can be normal. However, interictal epileptiform discharges frequently consist of irregular generalized spike and waves or polyspikes with infrequent 2- to 3-Hz generalized spike-and-wave complexes (104). Due to variable clinical presentations, interictal EEG may also present with focal epileptiform discharges, for example, in the frontal, temporal, and occipital regions (108).
Genetics
Mode of inheritance has been described as autosomal dominant with incomplete penetrance in a number of family pedigrees (104–108).
Five genes have been associated with GEFS+ thus far, three genes encode for a sodium channel subunit and two encode for GABAA receptor subunit. SCN1A encodes the α1 subunit of the neuronal voltage-gated sodium channel and was implicated in GEFS+ by identified mutations (109). It is suspected to increase excitability by decreasing the inactivation of the channel. SCN1B, which encodes the β1 subunit of the neuronal voltage-gated sodium channel, has also been found to be mutated in families with GEFS+ (110). This mutation is suspected to interfere with the modulation of the gating of the sodium channel leading to neuronal hyperexcitability. SCN2A has also been implicated by a missense mutation in a patient with GEFS+ (111). The γ-2 subunit of the GABAA receptor (GABRG2) has also been shown to be mutated in patients with the clinical phenotype of GEFS+ at that benzodiazepine-binding site (112). The mutation is predicted to reduce the flow through the channel, decreasing its inhibitory effect.
Treatment
The decision to treat pharmacologically should be made based on seizure frequency and severity of afebrile seizures. Clinical presentation and individual seizure types should determine the treatment approach and selection of AED if applicable. Due to paucity of reported cases, only little information on the efficacy of specific pharmacologic treatments is available. Gerard et al. report pharmacologic treatment in 6 out of 15 affected individuals “with success and seizure control in most” after analysis of a multigeneration pedigree of GEFS+ patients in France (113). Interestingly, in one family with a mutation in the GABRG2 gene (GEFS+ type 3), decreased benzodiazepine sensitivity has been reported (114).
Prognosis
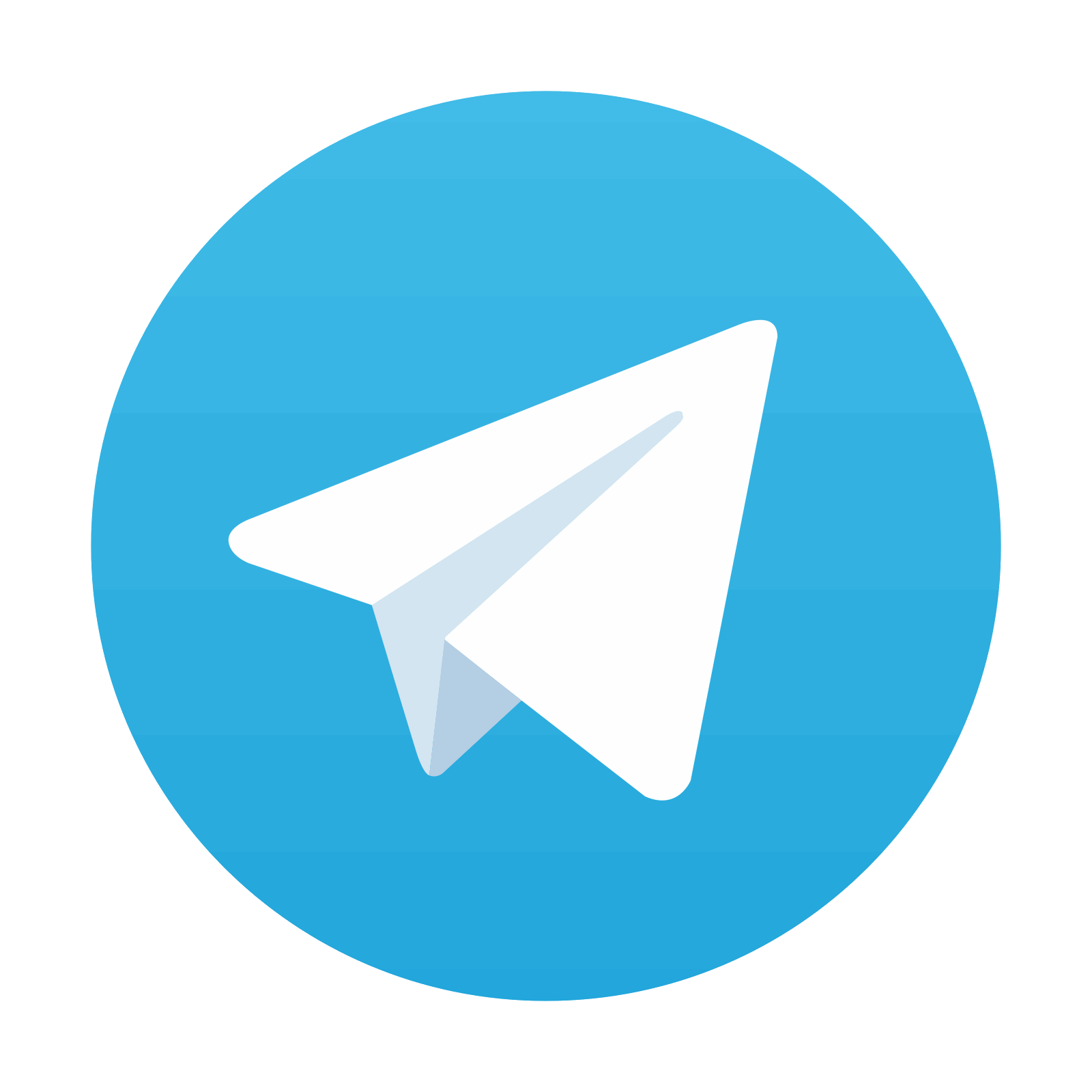
Stay updated, free articles. Join our Telegram channel

Full access? Get Clinical Tree
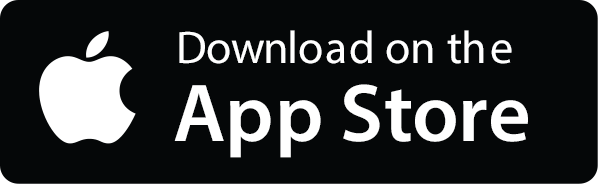
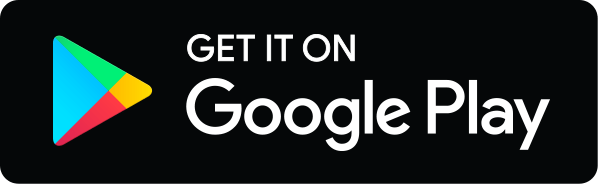