Study
No. of patients implanted (n)
Follow-up: average/median months
Improvement of at least 50% in intensity and/or frequency
Adverse events
14
17.5 months
5 (36%)
Lead migration (29%)
15
36.8 months
12 (80%)
Electrode migration (6%); infection (20%)
de Quintana-Schmidt [20]
4
6 months
4 (100%)
Fontaine [21]
13
14.6 months
10 (77%)
Infection (8%)
Mueller [23]
24
20 months
21 (88%)
Explantation due to infection (22%); cable breaks (11%); lead migration (4%)
Fontaine [22]
44
12 months
26 (60%)
Hardware/stimulation dysfunction (16%); electrode migration (3%)
Leone et al. [13]
35
73.2 months
20 (57%)
Electrode migration (20%)
Miller et al. [12]
51
39.2 months
27 (53%)
Electrode erosion (4%); lead migration (2%); infection (2%)
Total
200
125 (62.5%)
13.2.3 Vagal Nerve Stimulation
13.2.3.1 Mechanism and Use of Vagal Nerve Stimulation
The vagus nerve has connections to several brain centres important in pain regulation including the spinal trigeminal nucleus and the nucleus tractus solitarius. Studies in rats have identified reduction in pain and allodynia in the trigeminal distribution through stimulation of the vagus nerve, most likely secondary to ascending antinociceptive effect of that nerve on the second order neurons of the spinoreticular and spinothalamic tract [26, 27]. Early studies suggested direct inhibition of the afferents to the caudal trigeminal nucleus with acute stimulation of the vagus nerve [28]. Recent neuroimaging studies have shown inhibition of the activation of several structures involved in the pain matric of headaches, including the thalamus, limbic system and nucleus tractus solitarius, with chronic vagus nerve stimulation [29]. Studies have also suggested that stimulation of the vagus nerve may reduce glutamate concentration in the trigeminal nucleus caudalis, in turn possibly reversing central sensitization in chronic headache [30]. Therefore, activation of parasympathetic systems during attacks supports the use of non-invasive vagus nerve stimulator (nVNS) through the inhibition of afferent networks and neurotransmitters [26, 28, 30].
The gammaCore device is a non-invasive handheld transcutaneous vagal nerve stimulator applied to the neck (Electrocore©).
13.2.3.2 Evidence Base for Vagal Nerve Stimulation
The current evidence for the use in prevention of cluster headache attacks is limited to a manufacturer-sponsored trial involving 97 subjects. This trial of standard of care plus vagal nerve stimulation versus standard of care alone was conducted on the preventative and acute treatment of CCH using the gammaCore device Regular use of gammaCore for 4 weeks was associated with a significant reduction in attack frequency in the active compared to standard of care group (5.9 vs. 2.1 less attacks per week; p = 0.02) [31]. Similarly, the rate of subjects reporting more than 50% reduction in weekly attacks was higher in the active group (40% vs. 8.3%; p < 0.001). For comparison, the responder rate for verapamil 360 mg daily was 80% versus 0% for placebo in the single small randomized controlled trial available [32]. There are no well-controlled trial data on the use of VNS as a preventive treatment in ECH.
There are two randomized sham-controlled trials of vagus nerve stimulation in acute treatment of cluster headache [33, 34]. These studies were performed in episodic and chronic cluster headaches. Both studies failed to meet the primary end points of the trials. However, a post-hoc analysis showed superiority of nVNS in ECH. In ACT1, gammaCore resulted in a higher response rate (RR) (RR, 3.2; P = 0.014), higher pain-free rate for >50% of attacks (RR, 2.3; P = 0.045), and shorter duration of attacks (mean difference [MD], −30 min; P < 0.01) compared with the sham group [33]. In ACT2, gammaCore resulted in higher odds of achieving pain-free attacks in 15 min (OR, 9.8; P = 0.01), lower pain intensity in 15 min (MD, −1.1; P < 0.01) and higher rate of achieving responder status at 15 min for ≥50% of treated attacks (RR, 2.8; P = 0.058) compared with the sham group [34]. These data suggest that nVNS stimulation may be beneficial as an acute treatment in ECH but not CCH.
Reported side effects of nVNS are mild and include transient hoarseness, voice change, skin irritation, muscle ache and uncomfortable paraesthesia.
From the current evidence, the nVNS can be considered for the preventative treatment of chronic cluster headache as well as acute treatment of ECH but not CCH.
13.3 Central Neuromodulation Techniques
13.3.1 Cervical Spinal Cord Stimulation (SCS)
13.3.1.1 Mechanism and Use of SCS
Application of high cervical spinal cord stimulation (SCS) to treat TACs is based on clinical data from studies using SCS to treat other chronic pain conditions, in particular chronic back pain [35, 36]. In animal spinal cord models, afferent nociceptive inputs have been found to be inhibited by modulating a wide range of neuronal activity. For example, in chronic pain states, wide dynamic range (WDR) neurons are frequently hyperactive. Preclinical models have demonstrated that stimulation of these neurons at high frequency results in desensitization and decreased neuronal output, subsequently restoring them closer to their preinjury condition [35].
13.3.1.2 Operative Technique
SCS implantation for CH is similar to the techniques used for chronic back pain. Patients initially undergo a test stimulation phase for 7–14 days, where either one or two octad leads are placed in the epidural space. Fluoroscopy is used to determine the appropriate entry point on the skin, based on accessing the upper thoracic spine (usually the T2–3 interspace). After local anaesthetic is injected, a small incision is made under conscious sedation. Using fluoroscopic guidance, a 14-gauge Touhy needle is inserted into the T2–3 interspace and advanced cranially into the dorsal epidural space. Epidural placement is confirmed using a saline probe with loss of resistance technique.
Electrode(s) are advanced cranially in the dorsal epidural space until the distal lead tip reaches the area between the occiput and the C2 vertebral body. For normal frequency stimulation, intraoperative test stimulation is performed to confirm the presence of ipsilateral paraesthesia over the neck, occipital, parietal and frontal scalp areas, as well as the facial areas encompassing the C2 root sensory supply and V1–V2 trigeminal division. Test stimulation is not performed for high-frequency, paraesthesia-free stimulation systems.
Leads are anchored by suturing them to the supraspinal ligament and temporary extensions are connected and tunnelled under the skin surface. The extensions are then connected to an external stimulator during the trial period. High-frequency stimulation targets the dorsal columns at the C2–3 level, with parameters performed at 10 kHz frequency, 30 μs pulse width and 1.4–4 mA. If test stimulation is successful, permanent extensions and an internal pulse generator are implanted, typically in the gluteal region [37, 38].
13.3.1.3 Evidence for SCS
High cervical spinal cord stimulation in medically resistant chronic cluster headache
Study | No. of patients implanted (n) | Follow-up: average months | Pain-free patients (n) | Improvement of at least 50% in intensity and/or frequency | Adverse events |
---|---|---|---|---|---|
Wolter [37] | 7 | 23 (3–78) | 1 | 5 | Dislocated lead requiring revision [2], lead fracture requiring revision [1] |
Lambru [38] | 1 | 11 | 0 | 1 | Lead migration requiring revision |
13.3.2 Deep Brain Stimulation (DBS)
13.3.2.1 Mechanism and Use of DBS
CH shows a striking periodicity. This consists of a circadian periodicity of single attacks often occurring at given hours of night and day, as well as a circannual periodicity of the cluster headache periods (the bouts) that typically follow a seasonal pattern. This periodicity strongly suggests that the biological clock mainly located in the hypothalamus has a role in the pathophysiology of cluster headache. A number of neuroendocrinological studies showed abnormalities confirming a derangement in some hypothalamic functions as the regulation of circadian hormone secretion, melatonin, cortisol and others.
The role of the hypothalamus in cluster headache was confirmed in a positron emission tomography (PET) study that showed activation in the ipsilateral inferior hypothalamic grey matter during a cluster headache attack. However, a comparison of the PET and fMRI studies reveals that the diencephalic/mesencephalic activation is more posteroinferior in the PET studies, straddling the hypothalamus and midbrain tegmentum, whereas the activation is centred on the hypothalamus in the higher spatial resolution fMRI studies [39]. A voxel-based morphometry study demonstrated increased neuronal density in the same area, but the study design was poor and another larger better designed study failed to reproduce this finding [40, 41].
These observations led to hypothesize that high-frequency hypothalamic stimulation could inhibit activation seen in cluster headache [7]. This was the case when a completely refractory chronic cluster headache patient received high-frequency hypothalamic stimulation with complete remission of attacks [7]; when stimulation was interrupted, attacks recurred, confirming that placebo effect was not behind the efficacy [8].
Potential DBS candidates should be evaluated at a specialized DBS centre by a multidisciplinary team consisting of neurologists, neurosurgeons and a neuropsychologist. In large-volume DBS centres, overall risks of the procedure can be lower than 1% for intracranial haemorrhage [42–44] and 2% for hardware infection [43, 45]. Other potential complications include seizure, hardware discomfort and hardware failure. Seizures are rare and typically transient, occurring only in the immediate post-operative period. Transient side effects associated with stimulation in the hypothalamic and ventral tegmental area may include vertical diplopia, dizziness, vertigo and emotional disturbances (i.e. panic, anxiety) [46, 47].
13.3.2.2 Operative Technique
DBS leads can be implanted with myriad stereotactic techniques and utilizing magnetic resonance imaging (MRI)-guided techniques or MRI-computerized tomography (CT) fusion. Most studies for TACs use frame-based (Leksell) stereotaxy with intraoperative microelectrode recording and test stimulation. Many centres target the posterior hypothalamus using atlas coordinates based on the midcommisural point (MCP). Target location varied between 2 and 6 mm posterior to the MCP, 0–2 mm lateral to the MCP and 1–3 mm below the mid-commissural plane. The procedure is performed under conscious sedation, and the electrode is introduced in a rigid cannula, 10 mm to target. Intraoperative test stimulation is performed typically at 60 μs, 180 or 185 Hz. Side effects seen with higher voltage macrostimulation of the posterior hypothalamus include diplopia, subjective mood changes (i.e. feelings of anxiety, fear and/or panic), vertigo and changes in blood pressure or pulse rate [48–57].
The institute of one of the authors, The National Hospital for Neurology and Neurosurgery, Queen Square, UK, has adopted an MRI-guided, MRI-verified approach, without microelectrode recording, utilizing frame-based stereotaxy (Leksell frame model G) under general anaesthesia. This technique has been previously published for other DBS targets used in movement disorders [58, 59] and was used in our recent reports of chronic cluster headache and SUNA patients treated with ventral tegmental area (VTA) DBS. The most distal contact on the Medtronic 3389 lead is placed in the ventral tegmental area, which is visualized on a 1.5 T T2-weighted axial MRI sequence at a level immediately superior to the mammillary bodies, anteromedial to the red nucleus, and posterolateral to the mammillothalamic tract. An immediate post-implantation stereotactic MRI is obtained for patients without ONS implants to confirm lead positioning, and a stereotactic computerized tomography scan (CT) is obtained for patients with existing ONS hardware. Internal pulse generators are implanted in the infraclavicular area either in the same procedure or within a week after surgery [46].
After implantation, stimulators are programmed at 60 μs, 180–185 Hz, and the voltage is titrated based on clinical benefit and side effect profiles. The stimulation is delivered chronically, and patients are not typically given adjustable parameters, as is sometimes done during therapy for movement disorders such as Parkinson’s disease or essential tremor. Patients are usually evaluated more frequently in the initial 2–3 months. Similar to occipital nerve stimulation, if there has been no improvement after 6–12 months of stimulation, it is unlikely that stimulation will provide any clinical benefit [6].
13.3.2.3 Evidence for DBS
Deep brain stimulation for the treatment of medically resistant chronic cluster headache
Study | Patients (n) | Follow-up (years) | Pain-free patients (n) | Improvement of at least 50% in intensity and/or frequency | Surgical or device-related adverse events |
---|---|---|---|---|---|
19 | 8.7 | 6 | 12 (63%) | Electrode displacement (N = 2), infection (N = 4), electrode malpositioning (N = 1), third ventricle haemorrhage (N = 1), unilateral weakness (N = 1), seizure (N = 1) | |
Schoenen [7] | 6 | 4 | 2 | 3 (50%) | Fatal intracranial haemorrhage (N = 1), aborted procedure due to intraoperative panic attack (N = 1) |
D’Andrea [62] | 3 | 2.5 | 2 | 2 (67%) | |
Starr [52] | 4 | 1 | 0 | 2 (50%) | Transient ischemic attack 5 min after intraoperative stimulation (N = 1) |
Owen [53] | 1 | 0.7 | 1 | 1 (100%) | |
Bartsch [54] | 6 | 1.4 | 2 | 3 (50%) | Hardware discomfort over connection cable requiring revision [1] |
Fontaine [56] | 11 | 1 | 3 | 6 (55%) | Subcutaneous infection requiring hardware removal [1]; transient loss of consciousness after hemiparesis (N = 1), micturition syncope (N = 1) |
Hidding [63] | 1 | NR | 0 | 0 (0%) | Constant dull headache, high frequency tremor (N = 1) |
15 | 5.1 | 5 | 15 | Intracerebral electrode breakages (N = 4), euphoria, well-being, dizziness and oculomotor disturbances, concentration difficulties, headache, cervical dystonia, increased appetite | |
Akram [46] | 21 | 1.5 | 0 | 11 | Diplopia (N = 2), superficial infection resolved with antibiotics (N = 1), sleep disturbance (N = 2) |
Total | 87 | 21 (24%) | 55 (63%) |
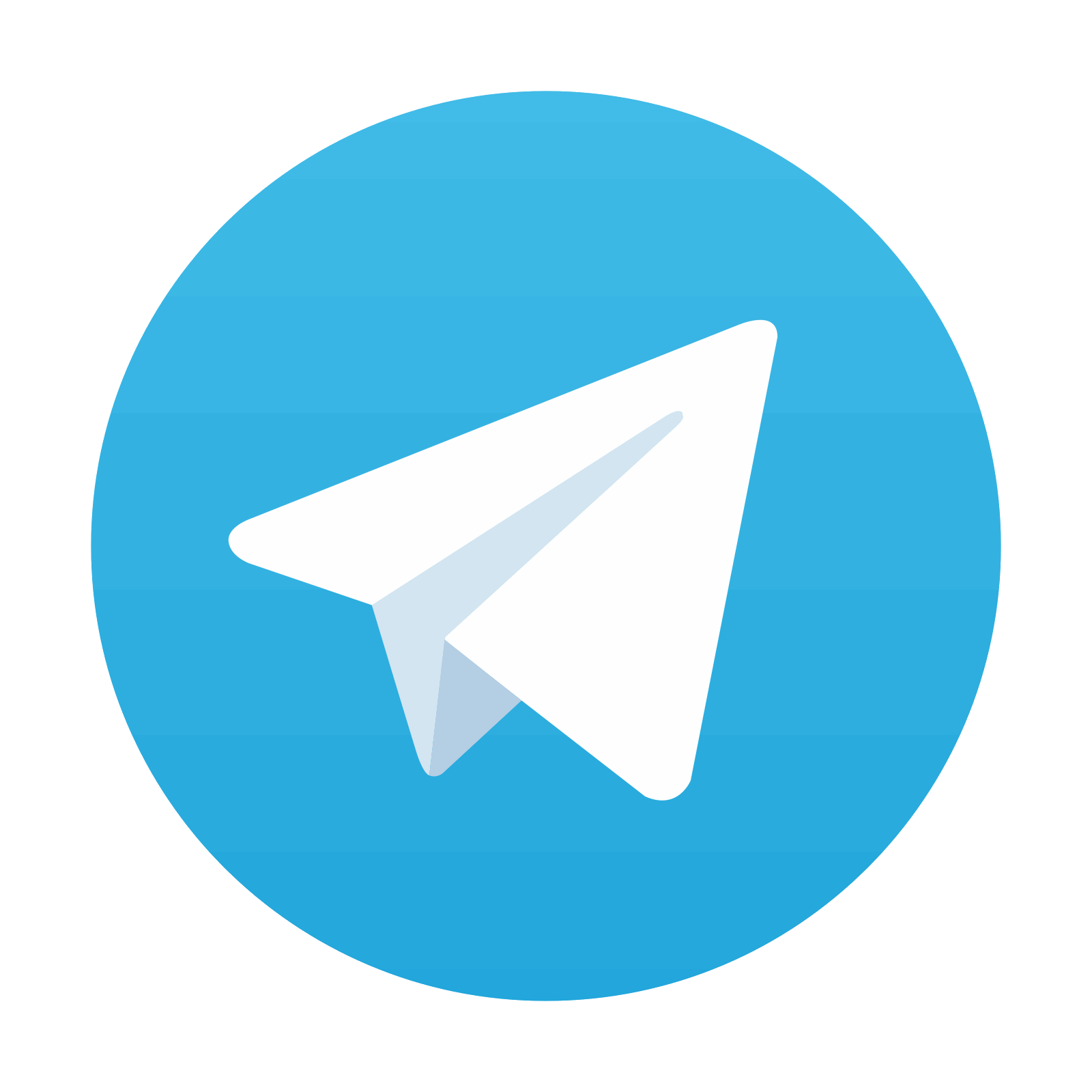
Stay updated, free articles. Join our Telegram channel

Full access? Get Clinical Tree
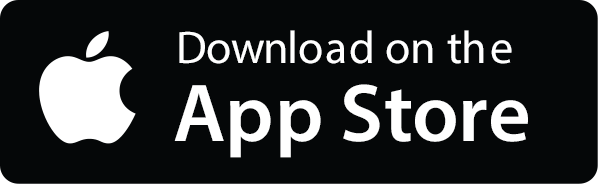
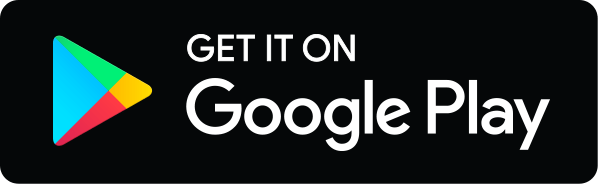