Chapter 6 Infectious and Noninfectious Inflammatory Diseases of the Brain
ANATOMICALLY BOUNDED INFECTIOUS PROCESSES
Beneath the subdural space are two other layers of connective tissue, the arachnoid mater and pia mater, which together constitute the leptomeninges. The arachnoid is a delicate outer layer that parallels the dura and is separated from the pia by the subarachnoid space, which contains the cerebrospinal fluid (CSF). The pia is closely applied to the brain and spinal cord and carries a vast network of blood vessels. Figure 6-1 illustrates this anatomy.
Epidural Abscess
Epidural abscesses can cross the midline, distinguishing them from a subdural empyema, which is usually confined by the midline falx. Epidural abscess, like other epidural lesions such as hematoma, can be confined by sutures. A specific site of origin for the infection and its contiguous spread into the extracerebral space also helps establish the diagnosis of epidural abscess (Fig. 6-2).
Subdural Empyema
Disruption of the arachnoid meningeal barrier by infection leads to the formation of CSF collections within the potential compartment of the subdural space. These may present acutely or chronically, and can be sterile or infected at time of presentation. Empyema rather than abscess is the appropriate term for a purulent infection in this potential space. Box 6-1 lists the causes of subdural empyema. Among the several possible mechanisms by which a subdural empyema is thought to form are (1) a distended arachnoid villus could rupture into the subdural space and infect it; (2) phlebitic bridging veins (secondary to meningitis) may infect the subdural space; (3) the subdural space may be infected by direct hematogenous dissemination; and (4) direct extension may occur through a necrotic arachnoid membrane from the subarachnoid space or from extracranial infections.
Features of subdural empyema are those of extracerebral collections over the convexities and within the interhemispheric fissure, which on magnetic resonance (MR) display isointensity on T1WI and high signal on T2WI/FLAIR, and on CT show an isodense to low density extra-axial mass (Fig. 6-3). Empyema may be distinguished from subdural effusion on DWI if restricted diffusion is identified in the former; that is, empyemas are hyperintense on DWI (low apparent diffusion coefficient [ADC] value), whereas sterile effusions are low intensity on DWI and have ADCs similar to those of CSF. There may be effacement of the cortical sulci and compression of the ventricular system. A rim of enhancement may be observed. This enhancement occurs from granulation tissue that has formed over time in reaction to the adjacent infection. Coronal MR is a useful aid in confirming the exact location of the collection.
Leptomeningitis
The pathologic process of meningitis (leptomeningitis) involves inflammatory infiltration of the pia mater and arachnoid mater (Fig. 6-4). Leptomeningeal inflammation most often occurs after direct hematogenous dissemination from a distant infectious focus. Pathogens also gain access by passing through regions that may not have a normal blood-brain barrier, such as the choroid plexus or circumventricular organs. Direct extension from sinusitis, orbital cellulitis, mastoiditis, or otitis media is also possible, as is infection after brain surgery. After septicemia, bacteria may lodge in venous sinuses and precipitate inflammatory changes, which in turn can interfere with CSF drainage, leading to hydrocephalus. With stagnation of CSF flow, bacteria are offered the opportunity to invade the meninges and indulge themselves. Early in the course of infection, congestion and hyperemia of the pia and arachnoid mater are present. Later, an exudate covers the brain, especially in the dependent sulci and basal cisterns. The leptomeninges become thickened. Clinical features are related to patient age (Box 6-2). Infants and particularly neonates may have a perplexing clinical picture, lacking physical signs that directly demonstrate meningeal irritation.
Shortly after the onset of meningitis, it is not uncommon to visualize marked enhancement of the leptomeninges, better visualized on MR than on CT (much more common with bacterial lesions). Postgadolinium FLAIR images appear to be very sensitive for subarachnoid disease. Parenchymal abnormalities (uncommon) are primarily those of high signal on T2WI/FLAIR or of low density on CT. DWI is usually normal unless adjacent encephalitis develops. Vasculitis may ensue and involve either arteries or veins; hence, patterns of infarction associated with meningitis differ depending on the location, number, and type of vessels involved. It is more demarcated than cerebritis. Box 6-3 summarizes the spectrum of imaging abnormalities in purulent meningitis.
Many additional complications occur as a result of inflammation involving the meninges. These sequelae are better imaged and characterized than are the manifestations of the meningitis itself. Communicating hydrocephalus can occur as both an early and a late manifestation of leptomeningitis, often becoming symptomatic to the point of requiring ventricular shunting. The subacute imaging findings of complicated leptomeningeal infection are those of atrophy, encephalomalacia (infarction), focal abscess, subdural empyema formation, and basilar loculations of CSF (Box 6-4).
Sinusitis can serve as a nidus for leptomeningeal infection and can produce septic thrombosis of adjacent venous sinuses and pseudoaneurysm formation if the cavernous sinus is affected (Fig. 6-5). Labyrinthitis ossificans (see Chapter 12) may occur as a late finding secondary to infiltration of the cochlear channels by infected CSF.
Neonates represent a special case with respect to the cerebral sequelae of bacterial leptomeningitis. The most commonly encountered organism is gram-negative bacilli, followed by group B Streptococcus, Listeria monocytogenes, and others. The neonatal meningitides are believed to be acquired as a result of the delivery process, chorioamnionitis, immaturity, or iatrogenic problems (e.g., catheters, inhalation therapy equipment). The lack of a developed immune system at birth makes neonates susceptible to organisms that are normally not very virulent. These children frequently have severe parenchymal brain damage as a result of the infection that ultimately produces a multicystic-appearing brain, often with hydrocephalus. The late imaging findings are those of multifocal encephalomalacia leading to multiple distended intraventricular and paraventricular cysts (Fig. 6-6). In children (age 1 month to 15 years), Haemophilus influenzae is a common pathogen associated with upper respiratory infections and can produce a virulent meningitis with vascular infarction. Other bacteria in this group are Neisseria meningitidis and Streptococcus pneumoniae. In adults, S. pneumoniae and N. meningitidis are the most common bacterial organisms producing meningitis. H. influenzae characteristically produces a high rate of subdural effusions.
What should you look for before clearing a patient for LP? Be sure that you do not see cerebellar tonsils impacted in the foramen magnum, cerebellar herniation syndromes, obliterated or trapped fourth ventricles (see Chapter 8), cerebellar masses or strokes, transtentorial downward herniation, completely effaced basal cisterns/sulci, or significant subfalcine herniation. Obliteration of the superior cerebellar and quadrigeminal plate cisterns with sparing of the ambient cisterns is concerning. Better to err on suggesting use of clinical acumen in diagnosing meningitis than clearing a patient for LP and having them herniated and die from the “approved tap.”
Leptomeningitis versus Pachymeningitis (Fig. 6-7)
These entities may be legitimately separated by their enhancement pattern on MR. Leptomeningeal enhancement follows the gyri/sulci or involves the meninges around the basal cisterns (because the dura-arachnoid is widely separated from the pia-arachnoid here) (see Figs. 6-4 and 6-5A). Pachymeningeal enhancement is thick and linear/nodular, following the inner surface of the calvarium, falx, and tentorium and without extension into the sulci or involvement of the basal cisterns (see Fig. 6-7). An inflammatory process involving endothelial cells opens the tight junctions, allowing the pathogens to reach the leptomeninges and producing leptomeningitis. In carcinomatous meningitis, the tumor cells lack the properties that bacterial cell walls possess. They do not produce the same inflammatory process and the blood-brain barrier remains intact. The tumor cells can pass through the capillaries (no tight junctions) of the dura, resulting in dural inflammation. CSF cytology in these cases may be negative. As everyone is aware, the leptomeninges can be involved by tumor. In such cases, the CSF is positive and the tumor cells probably get there via the choroid plexus (no blood-brain barrier) or from extension of superficial parenchymal lesions. Box 6-5 provides a list of conditions that can produce pachymeningeal versus leptomeningeal enhancement.
Idiopathic Hypertrophic Cranial Pachymeningitis
This is a rare disorder characterized by severe headache, cranial nerve palsies, and ataxia. The peak age is in the sixth decade. The clinical course is chronic with some initial improvement with steroids. On T1WI the dura is obviously thickened, sometimes in a pseudotumor fashion, and avidly enhances, whereas on T2WI the dura is hypointense and there is no involvement of the brain (see Fig. 6-7). The differential diagnosis includes meningioma, sarcoid, tuberculosis, and syphilis. The syndrome is in the category of orbital pseudotumor, Tolosa-Hunt syndrome, and other fibrotic syndromes.
Pyogenic Brain Abscess
Cerebral abscess is most often the result of hematogenous dissemination from a primary infectious site. The various causes of cerebral abscess are listed in Box 6-6. The most frequent locations are the frontal and parietal lobes in the distribution of the middle cerebral artery. Intracranial abscess affects predominantly preadolescent and middle-age groups. In part, this is related to the incidence of congenital heart disease, IV drug abuse, acquired immune deficiency syndrome (AIDS), and tympanomastoid and paranasal sinus infections. In all series there is a preponderance of male patients over female. Abscesses may be unilocular or multilocular, solitary or multiple. A variety of bacterial organisms are commonly cultured from brain abscesses (Box 6-7). In addition, numerous other pathogens can infect the brain when the immune system is compromised.
The characteristics of cerebral abscess depend on the pathologic phase during which the abscess is being examined. In the cerebritis phase, CT demonstrates low-density abnormalities with mass effect. Patchy or gyriform enhancement is present. On MR, one may see low intensity on T1WI and high signal intensity on T2WI/FLAIR (Fig. 6-8), with a typical epicenter at the corticomedullary junction and patchy enhancement. In the late cerebritis phase, ring enhancement may be present. The presence of ring enhancement should not unequivocally imply capsular formation. It is important for the surgeon contemplating drainage to appreciate that a firm, discrete abscess may not be present despite ring enhancement.
After 2 to 3 weeks a mature abscess appears on T1WI as a round, well-demarcated low-intensity region with mass effect and peripheral low intensity (edema) beyond the margin of the lesion. On T2WI/FLAIR, high intensity is noted in the cavity and in the parenchyma surrounding the lesion (Fig. 6-9). Concentric bands of varying thickness on T2WI/FLAIR have been seen in abscesses. DWI is usually positive (Fig. 6-10).
On CT, the encapsulated intracerebral abscess shows a low-density center and low density surrounding the lesion (edema). Ring enhancement is virtually always present in pyogenic brain abscess. Thickness, irregularity, and nodularity of the enhancing ring should raise the suspicion that one is dealing with a tumor (most of them) or an unusual infection (e.g., fungus). However, many exceptions to this rule occur and nodular, irregular pyogenic abscesses are not that infrequent. Some of these represent subacute and chronic abscesses, whereas others are the result of adjacent daughter abscess formation. Multiple ring-enhancing lesions are more consistent with hematogenous dissemination of an infectious focus. Multiple rings in a single location can be seen with daughter abscesses but have also been noted with glioma (and other lesions). Box 6-8 is a partial differential diagnosis of the ring-enhancing lesion.
An uncommon observation on noncontrast CT is the presence of a complete ring (Fig. 6-11). The noncontrast ring is most often identified in metastases, less often in abscess or glioma, and hardly ever in hematoma or infarct. This finding could help in unknown cases of ring enhancement by narrowing the differential diagnosis.
Several reports in the literature discuss proton MRS (1HMRS) in pyogenic abscess. These include resonances from cytosolic amino acids (0.9 ppm), acetate (1.92 ppm), lactate (1.3 ppm), and alanine (1.5 ppm). It is believed that the presence of amino acid resonances may distinguish pyogenic abscess from necrotic brain tumors. See Box 6-9 for MRS helpful hints.
Ventriculitis (Ependymitis)
Ventriculitis can be seen as part of the spectrum of infection, including meningitis as a postoperative complication (particularly related to ventricular shunting) or as an isolated finding. Ventriculitis is more commonly seen in neonates with meningitis and is a feature of cytomegalovirus (CMV) infection as well as some peripartal anaerobic infections. Organisms are introduced into the ventricle as a result of bacteremia, from abscess, trauma, or instrumentation of the ventricle. The ventricles can be enlarged, and on T2WI/FLAIR high intensity can be observed surrounding the ventricles or even within the CSF on FLAIR. The key to the diagnosis is subependymal enhancement (Fig. 6-12). Choroid plexus enhancement has been reported. Periventricular calcification can be seen on CT after neonatal ventriculitis. Purulent ependymitis can be bright on DWI.
Choroid Plexitis
The normal choroid plexus is isodense on CT or isointense to brain on MR and enhances. Calcifications of the choroid plexus produce hypointensity on MR and high density on CT. Xanthogranulomatous change to the choroid plexus is bright on DWI and sometimes FLAIR but does not enhance much. Asymmetry of the lateral ventricle choroid plexus or bilateral symmetric enlargement of the choroid plexus should alert one to possible choroid plexitis. The differential diagnosis of choroid plexus disease is provided in Box 6-10.
Septic Embolus
The most frequent manifestation of infective endocarditis is stroke, with Staphylococcus aureus by far the most common organism. However, sepsis from any cause, including pulmonary arteriovenous malformations, pulmonary infection, intravenous drug abuse, infected catheters with cardiac septal defects, and occult infection may produce septic emboli to the brain. Septic emboli are associated with persistent mass effect, edema, and enhancement beyond a 6-week period. This should alert the radiologist to consider septic infarction with development of abscess formation in association with cerebral infarction. Rarely, bland infarction may serve as a nidus for subsequent bacterial colonization from bacteremia and abscess formation. Another diagnostic possibility would be tumor emboli mimicking a stroke. This usually results in hemorrhage or tumoral edema. Cardiac myxoma can embolize and produce acute stroke, and later the tumor grows into the vessel wall to produce aneurysmal dilatation. The vast majority of septic emboli are self-induced by drug users. Septic emboli result in brain abscess, mycotic aneurysm (these occur in distal vessels, usually the middle cerebral artery, and are less likely to hemorrhage), or obliterative vasculitis (Fig. 6-13). Mycotic aneurysm presents with intraparenchymal-dominating subarachnoid hemorrhage. DWI scans may be positive for both the stroke and the infection, but the size is smaller than typical strokes.
This section is not intended to be comprehensive but provides a sample of what you might encounter.
VIRAL (TABLE 6-1)
Herpes Simplex
Herpes simplex virus (HSV) is the most common cause of fatal endemic encephalitis in the United States. The survivors of infections with this virus have severe memory and personality problems. Early diagnosis and therapy with antiviral agents can favorably affect the outcome. Both the oral strain (type 1) and the genital strain (type 2) may produce encephalitis in human beings. Type 2 is responsible for infection in the neonatal period, presumably acquired either transplacentally or during birth from mothers with genital herpes. This strain may cause a variety of teratogenic problems, including intracranial calcifications, microcephaly, microphthalmia, and retinal dysplasia. It carries a high morbidity and mortality rate, with CNS infection either primary or part of a disseminated infection. Sequelae from neonatal herpes also include multicystic encephalomalacia, seizures, motor deficits, mental and motor retardation, and porencephaly. The features of intracranial neonatal herpes are different from those in adults and are summarized in Box 6-11. The early findings in neonatal herpes are subtle regions of low density on CT in various regions in the brain parenchyma, including gray matter and cerebellum. These regions enlarge rapidly, with meningeal and gyriform enhancement. Later gyri may demonstrate strikingly high density on noncontrast CT. Calcification can appear between 17 and 21 days after disease onset and can be variable in location. Thalamic hemorrhage has been observed. Atrophy is also seen early in this disease. The normal low intensity on T1WI of neonatal white matter and high intensity on T2WI/FLAIR limit the sensitivity of MR in this disease. Loss of gray-white contrast is an early abnormality that can be incorrectly interpreted as poor quality images. DWI may show gyriform high signal. The MR correlate of the high density in the cortex on CT is hypointensity on T2WI/FLAIR. The cause of these characteristic cortical changes is not understood, but increased cortical blood volume (with deoxyhemoglobin), calcification, laminar necrosis, and other associated paramagnetic ions may be possible causes. Cystic encephalomalacia is often the end game of neonatal herpes.
Table 6-1 Characteristic Targets of Viral Encephalitides
Virus | Favored Site of Involvement | Caveats |
---|---|---|
HSV-1 | Medial temporal lobe, insula, cingulum | May bleed, bilateral |
VZV | MCA infarcts | Vasculitis |
EBV | Brain stem and cerebellum | Mononucleosis symptoms, nodes, nasopharyngeal cancer |
EEE | Brain stem, deep gray matter | Whinny/deep gray matter involved in brain stem |
Japanese | Deep gray matter, brain stem | Giant panda sign |
HIV | White matter, atrophy | Opportunistic infections |
CMV | Periventricular, ependymitis | Congenital migrational anomalies |
JC virus | Subcortical white matter | Immunocompromised |
Nipah | Small-vessel infarcts in white matter | |
West Nile virus | Myelitis, central brain, and deep gray matter | |
St. Louis encephalitis | Substantia nigra | |
Rabies | Basal ganglia, brain stem | Tick bite |
Measles | Cortical edema, basal ganglia disease | Rash |
In type 1 herpes encephalitis, MR findings within the first 5 days of the disease show high intensity on T2WI/FLAIR and either positive or negative DWI in the temporal and inferior frontal lobes and progressive mass effect, including the cingulate region, whereas CT findings at this time may be subtle to nonexistent (Fig. 6-14). Negative diffusion images may indicate some possibility for reversibility. The earliest CT abnormalities are low-density areas in the temporal lobe and insular cortex. Hemorrhage may be identified. The areas of abnormality in the temporal lobe and insula abruptly end at the lateral putamen, which is characteristically spared. MR is frequently able to detect asymmetric bilateral temporal lobe involvement. This picture is virtually pathognomonic of HSV infection. Unlike other viral encephalitides (see later discussion), HSV type 2 rarely involves the basal ganglia. The full extent of parenchymal damage is difficult to assess during the first 10 days of the disease.
< div class='tao-gold-member'>
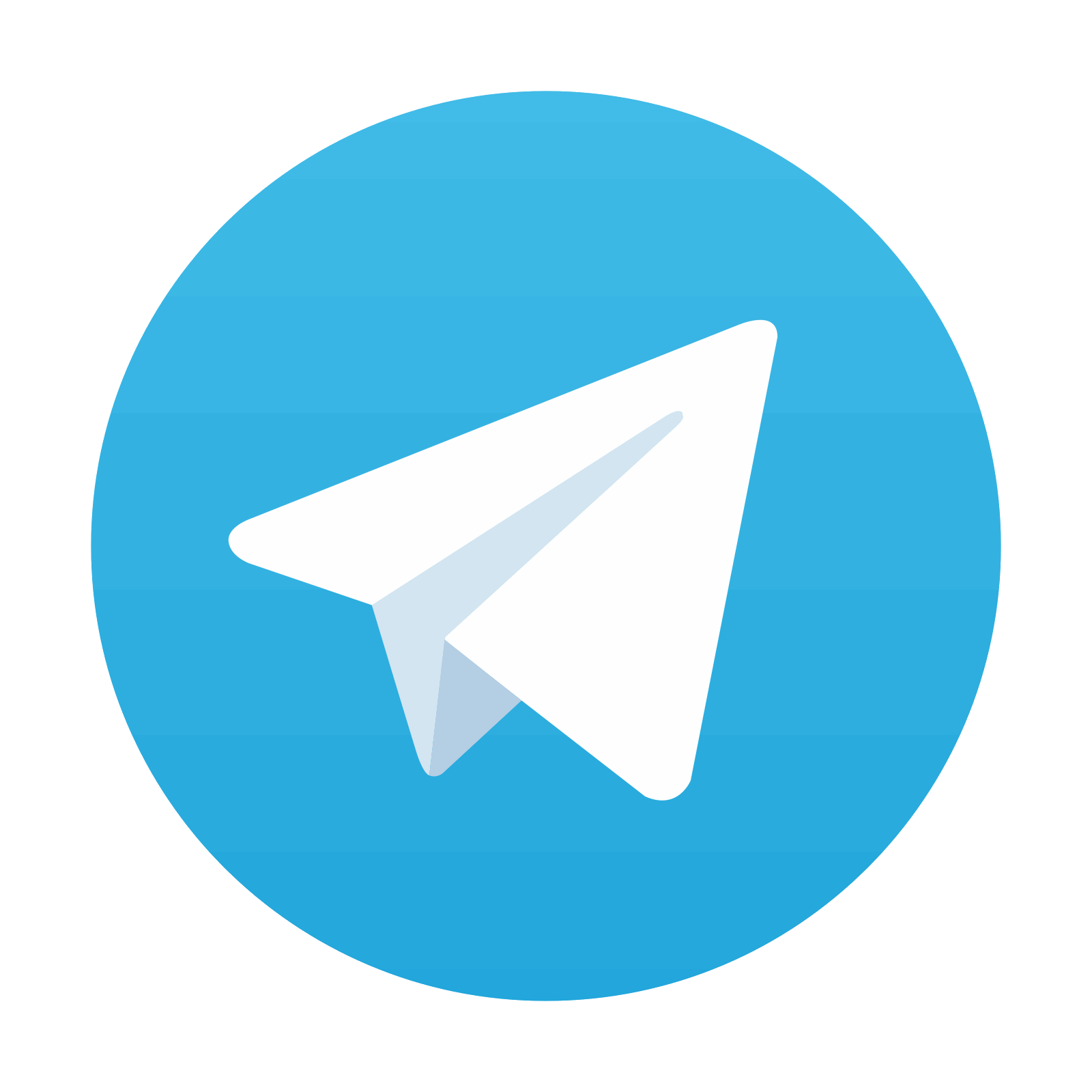
Stay updated, free articles. Join our Telegram channel

Full access? Get Clinical Tree
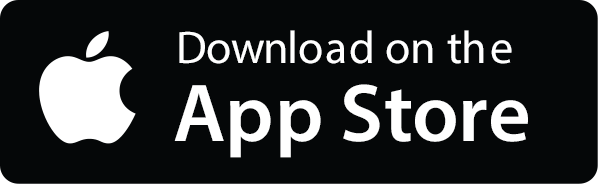
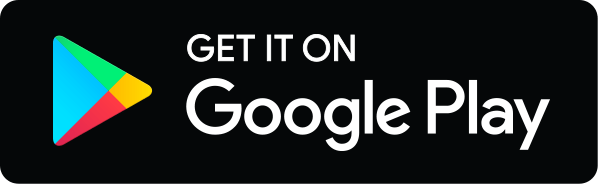