Morbidity and mortality of patients with aneurysmal subarachnoid hemorrhage (aSAH) is significantly related to the development of chronic cerebral vasospasm. Despite extensive clinical and experimental research, the pathophysiology of the events that result in delayed arterial spasm is not fully understood. A review of the published literature on cerebral vasospasm that included but was not limited to all PubMed citations from 1951 to the present was performed. The findings suggest that leukocyte-endothelial cell interactions play a significant role in the pathophysiology of cerebral vasospasm and explain the clinical variability and time course of the disease. Experimental therapeutic targeting of the inflammatory response when timed correctly can prevent vasospasm, and supplementation of endothelial relaxation by nitric oxide-related therapies and other approaches could result in reversal of the arterial narrowing and improved outcomes in patients with aSAH.
Aneurysmal subarachnoid hemorrhage (aSAH) remains a leading cause of morbidity and mortality in patients who survive the initial ictus, primarily as a result of the development of delayed or chronic vasospasm. Vasospasm after aSAH in humans is a biphasic phenomenon in which an acute phase that typically occurs 3 to 4 hours after an aSAH and generally resolves rapidly, is followed by a chronic phase that occurs 3 to 14 days later. This chronic or delayed phase is characterized by sustained arterial narrowing that can lead to permanent deficits and death in 20% to 40% of patients. Cerebral vasospasm has also been observed in other conditions, including traumatic brain injury, after craniotomies, and in meningitis. Interactions between leukocytes and endothelial cells are fundamental factors in the inflammatory response to injury, and seem to be critical components in the pathophysiology of posthemorrhagic cerebral vasospasm. This review summarizes the growing body of evidence that supports the prominent role of inflammation in this condition, and discusses its potential implications in the development of diagnostic and therapeutic strategies for this condition.
The inflammatory hypothesis of vasospasm after subarachnoid hemorrhage
During SAH, blood deposition into the subarachnoid space results in release of free hemoglobin (Hgb), which is extremely toxic. To counteract free Hgb toxicity, the immune system stimulates rapid expression of specific cell adhesion molecules (CAMs) on the luminal surface of the endothelial cells. This allows macrophages and neutrophils to bind to the endothelial cells and enter the subarachnoid space, where they phagocytose extravasated red blood cells (RBCs) and remove free Hgb. The binding and clearance of extracorpuscular Hgb relies on the identification of Hgb only when it is conjugated with haptoglobin (Hp), a serum protein that binds to free Hgb with high affinity.
After RBC phagocytosis and Hgb clearance, however, macrophages and neutrophils remain trapped in the subarachnoid space because of the absence of lymphatics in the central nervous system (CNS) and impaired cerebrospinal fluid (CSF) flow caused by the SAH, and within 2 to 4 days after their entry into the subarachnoid space, macrophages and neutrophils die and degranulate. This results in a release of intracellular endothelins (ET) and oxygen-free radicals into the interstitial and subarachnoid spaces that ultimately cause an inflammation-induced arteriopathy and arterial vasoconstriction. Arterial narrowing, however, is only 1 manifestation of the inflammatory response that follows SAH; the clinical deterioration of patients with arterial narrowing by imaging or ultrasonography is the result of a more complex and robust inflammatory response that results in meningitis and cerebritis. This hypothesis explains why the meningitic syndrome and arterial spasm seen after aSAH, which results in delayed ischemic deficits and stroke, is also present in other pathophysiologic entities such as bacterial meningitis and traumatic brain injury (TBI), among others. This hypothesis is illustrated in Fig. 1 .

Hematological components of vasospasm
Hgb and Hp
Hgb is the iron-carrying oxygen transport metalloprotein that constitutes most of the structure of the RBCs. Although intracorpuscular Hgb is usually degraded by macrophages in the reticuloendothelial system, extracorpuscular Hgb is a proinflammatory molecule that requires complex interactions for proper recycling. Free Hgb also reduces vasodilation induced by nitric oxide (NO), and the synergistic proinflammatory and vasospastic effects of extravascular Hgb seem to be critical in the development of vasospasm.
Hepatocytes synthesize large quantities of Hp in serum. This protein couples with Hb through a stable, high affinity bond and ameliorates the toxicity of free extracorpuscular Hgb. Although in humans the Hp gene has 2 alleles, designated Hp 1 and Hp 2, other mammalian species have only a single Hp1 allele. The dimeric protein coded by Hp 1-1 more efficiently binds and promotes the clearance of Hgb molecules when compared with the cyclical Hp 2-2 protein, and seems to have superior antiinflammatory, immunomodulatory, antioxidant, and vasodilatory effects in vitro and in animal studies. Hp 2-2, however, provides protection against some infectious disease, a characteristic that has promoted its dissemination in the human gene pool.
The Inflammatory Response
Inflammation constitutes a biphasic response with an acute and a chronic period. Although the acute period constitutes a short event that occurs immediately after the initial injury and uses polymorphonuclear neutrophils, macrophages, and monocytes as its primary effector cells, the chronic period occurs in a delayed manner in days or weeks, and has lymphocytes and plasma cells as the main effector cells. Acute inflammation results from the combination of a vascular response that includes arteriolar relaxation (increasing blood flow) and local endothelial cell contraction (increasing vascular permeability) and a cellular response that involves neutrophils, macrophages, and monocytes, which migrate to the inflamed site and phagocytose the particles that stimulated the inflammatory response. These inflammatory effector cells eventually degranulate, and release enzymes and other toxic intermediates into the extravascular space that precede and subsequently promote chronic inflammation. Continuous proinflammatory stimulation primarily mediated by lymphocytes and monocytes/macrophages that invade the affected tissue and release cytokines (eg, interferon-γ), reactive oxygen species, and hydrolytic enzymes result in chronic inflammation. This chronic component develops in days or weeks, and can persist for many months or years.
Hematological components of vasospasm
Hgb and Hp
Hgb is the iron-carrying oxygen transport metalloprotein that constitutes most of the structure of the RBCs. Although intracorpuscular Hgb is usually degraded by macrophages in the reticuloendothelial system, extracorpuscular Hgb is a proinflammatory molecule that requires complex interactions for proper recycling. Free Hgb also reduces vasodilation induced by nitric oxide (NO), and the synergistic proinflammatory and vasospastic effects of extravascular Hgb seem to be critical in the development of vasospasm.
Hepatocytes synthesize large quantities of Hp in serum. This protein couples with Hb through a stable, high affinity bond and ameliorates the toxicity of free extracorpuscular Hgb. Although in humans the Hp gene has 2 alleles, designated Hp 1 and Hp 2, other mammalian species have only a single Hp1 allele. The dimeric protein coded by Hp 1-1 more efficiently binds and promotes the clearance of Hgb molecules when compared with the cyclical Hp 2-2 protein, and seems to have superior antiinflammatory, immunomodulatory, antioxidant, and vasodilatory effects in vitro and in animal studies. Hp 2-2, however, provides protection against some infectious disease, a characteristic that has promoted its dissemination in the human gene pool.
The Inflammatory Response
Inflammation constitutes a biphasic response with an acute and a chronic period. Although the acute period constitutes a short event that occurs immediately after the initial injury and uses polymorphonuclear neutrophils, macrophages, and monocytes as its primary effector cells, the chronic period occurs in a delayed manner in days or weeks, and has lymphocytes and plasma cells as the main effector cells. Acute inflammation results from the combination of a vascular response that includes arteriolar relaxation (increasing blood flow) and local endothelial cell contraction (increasing vascular permeability) and a cellular response that involves neutrophils, macrophages, and monocytes, which migrate to the inflamed site and phagocytose the particles that stimulated the inflammatory response. These inflammatory effector cells eventually degranulate, and release enzymes and other toxic intermediates into the extravascular space that precede and subsequently promote chronic inflammation. Continuous proinflammatory stimulation primarily mediated by lymphocytes and monocytes/macrophages that invade the affected tissue and release cytokines (eg, interferon-γ), reactive oxygen species, and hydrolytic enzymes result in chronic inflammation. This chronic component develops in days or weeks, and can persist for many months or years.
Inflammation and chronic vasospasm
Although Ecker and Riemenschneider first described angiographic cerebral vasospasm in 6 patients with aSAH in 1951, William Gull had already reported a case consistent with vasospasm in England in 1859. Cerebral arterial vasospasm was experimentally recreated in laboratory models in the early twentieth century, and the clinicopathologic correlation of cerebral infarction in the presence of a patent cerebral vasculature was provided by Robertson in 1949, who studied a series of patients with ruptured aneurysms and concomitant cerebral infarction, and hypothesized that the infarctions resulted from transient spasm of the supplying arteries and not from mechanical compression from the aneurysms. The first correlation of angiographic vasospasm and focal neurologic deficits, however, was not reported until Fisher and colleagues’ study in 1977. Despite increasing clinical and experimental evidence, the pathophysiology of vasospasm continues to be elusive and modest therapeutic progress has been made to date.
Clinical Correlates of Inflammation and Vasospasm
Hyperthermia
Hyperthermia or fever was the first clinical sign that indicated a subjacent inflammatory response in these patients, and its appearance correlated with the onset of chronic vasospasm. These observations were later confirmed by Weir and colleagues, who found that of all patients with aSAH who developed clinical vasospasm, 60% had a temperature greater than 37.5°C in the 6 days before vasospasm onset, which represented nearly twice the incidence of patients with lower temperatures. These findings were replicated in other studies that also correlated persistent fever after aSAH with less favorable outcomes.
Leukocytosis
Increased white blood cell (WBC) counts have been shown to correlate with an increased risk of clinically significant vasospasm and worst outcomes. Although the impact of leukocytosis on clinical outcomes was first reported in 1974 by Neil-Dwyer and Cruikshank, a link between increased WBCs and vasospasm was not confirmed until 1987, when Spallone and colleagues correlated leukocytosis with the development of ischemia after aSAH. Detailed chronologic correlation between leukocytosis and the time course of chronic vasospasm was later provided by Niikawa and colleagues, and an independent association between peak leukocyte counts and the development of cerebral vasospasm was then reported in a multivariate analysis by McGirt and colleagues.
Serologic markers of inflammation in vasospasm
Immune complexes are seen in patients with posthemorrhagic vasospasm, along with activated complement cascade proteins, and C-reactive protein (CRP). In fact, Rothoerl and colleagues have shown that CRP is significantly increased in patients who developed symptomatic vasospasm and that it correlates with the worst neurologic outcomes.
Histopathologic changes in vasospastic cerebral vessels
Evidence of a significant inflammatory arteriopathy has been described on histopathologic examination of cerebral arteries from patients with clinical and angiographic vasospasm and is consistently replicated in multiple experimental models of the disease. For instance, increased endothelial penetration of monocytes within arteries in proximity to ruptured-aneurysm sites, macrophage invasion of the tunica media and adventitia of vessels in angiographic vasospasm, and positive immunofluorescence for IgM and C3 in the endothelium of spastic arteries in patients with aSAH have been reported.
Experimental Evidence of Inflammation in Vasospasm
Induction of vasospasm with proinflammatory agents
A clear pathophysiologic link between inflammation and cerebral vasospasm has been difficult to demonstrate despite numerous clinical studies that correlated a robust inflammatory response with the progression of vasospasm. To elucidate the nature and causality of this relationship, researchers have replicated arterial vasospasm in the absence of blood products and other conditions associated with SAH by administering several proinflammatory agents in experimental models. Vasospasm has been successfully induced by injected latex and dextran beads into the cistern magna of dogs, administration of polystyrene latex beads and talc (crystallized hydrous magnesium sulfate), and locally delivered lipopolysaccharide (LPS) into the subarachnoid space of rabbits. Controlled release of LPS in particular resulted in chronic vasospasm in a dose-dependent fashion, which replicated the basilar artery vasospasm induced by SAH in the same model. These studies demonstrated that significant arterial vasospasm could be induced despite the absence of RBCs or Hgb in the subarachnoid space and provide further confirmation of the role of inflammation in the development of chronic vasospasm.
Prevention of vasospasm with immunosuppressive or antiinflammatory agents
Immunosuppressive or antiinflammatory agents have been postulated as potential treatments for chronic cerebral vasospasm in various animal models and in a few human clinical trials with varied results. Among the proposed agents, corticosteroids, cyclosporine, tacrolimus (FK-506), and nonsteroidal antiinflammatory drugs (NSAIDs) have been most extensively studied.
Corticosteroids are steroidal hormones with antiinflammatory and immunosuppressive properties that primarily affect lymphocyte proliferation and function, and tend to suppress chronic rather than acute inflammation. Experimental administration of high dose methylprednisolone decreased cerebral vasospasm, ameliorated arterial wall abnormalities, and suppressed prostaglandin E2 synthesis in animal models. Human clinical studies by Chyatte and colleagues in 21 patients at high risk for vasospasm by clinical criteria showed that methylprednisolone therapy improved neurologic outcomes, decreased mortality, and reduced delayed cerebral ischemia. A multicenter study by Hashi and colleagues then followed that included 52 centers with 140 enrolled patients and evaluated the effects of hydrocortisone administration after vasospasm onset. Results showed improved mental status, speech, and motor function in hydrocortisone treated patients 1 month after treatment.
Cyclosporine causes T-cell dysfunction by inhibiting interleukin-2 (IL-2) transcription, and its use in animal models of experimental SAH has produced conflicting results. Clinical studies with cyclosporine have also had mixed results. Although in a study by Manno and colleagues cyclosporine failed to prevent chronic vasospasm in patients with Fisher grade 3 SAH, a study by Ryba and colleagues showed that a combination of cyclosporine with nimodipine significantly improved outcomes in patients who underwent early clipping (<72 hours) after a SAH.
In addition to their antipyretic and analgesic effects, NSAIDs also have potent antiinflammatory properties, mediated in part by a nonselective inhibition of cyclooxygenase expression, which reduces prostaglandin synthesis. Furthermore, certain NSAIDs such as ibuprofen have been shown to prevent leukocyte migration into the periadventitial space by inhibition of endothelial intercellular adhesion molecule 1 (ICAM1; CD54) expression. White and colleagues have also shown that intravenous NSAID administration in a canine model of SAH significantly reduced the severity of vasospasm. In this study, however, NSAIDs were injected 30 minutes before and 3 hours after induction of SAH, and despite their positive findings these time points would limit therapeutic replication in human trials.
Decreased levels of activated complement proteins in serum have been shown to ameliorate cerebral vasospasm in experimental models and in human subjects. Nafamostat mesilate is a serine protease inhibitor that prevents complement activation and experimentally reduced angiographic vasospasm in rabbits with hemorrhagic and latex bead-induced vasospasm. These findings were replicated in a small clinical trial in which Nafamostat reduced the incidence of vasospasm alone and in combination with a thromboxane synthetase inhibitor.
Current Molecular Evidence of Inflammation in Vasospasm
CAMs and leukocyte migration
The development of monoclonal antibodies led to the discovery of cell-adhesion molecules (CAMs), which facilitated a detailed understanding of leukocyte-endothelial cell interactions during inflammation. The 3 classes of CAMs that regulate leukocyte-endothelial cell interactions are selectins, integrins, and immunoglobulin superfamily proteins. Identification of the location and variable expression of CAMs elucidated and clarified the complex process that results in leukocyte adhesion, diapedesis, and migration, which is now known to involve 3 primary steps: selectin-facilitated rolling, chemokine-induced activation, and integrin-dependent arrest. The initial tethering of leukocytes to the vessel walls results from the interaction between sialylated carbohydrates on the leukocyte membrane and endothelial selectins. This interaction is followed by binding of leukocyte receptors to chemoattractants released from the injured tissue and integrin activation. Integrins in turn bind to immunoglobulin superfamily members expressed on the endothelium, which increases leukocyte adhesiveness and causes rolling leukocytes to arrest. Arrested leukocytes then diapedese and migrate to sites of inflammation.
Selectins
Selectin expression facilitates the formation of adhesions between leukocytes and endothelial cells and reflects an evolving inflammatory response. E-selectin has been found to be elevated in SAH patients, with higher concentrations seen in patients who develop moderate or severe vasospasm. Although P-selectin levels appeared to be higher in patients with low-grade SAH who developed ischemia, L-selectins were higher in patients who did not develop delayed cerebral ischemia. Selectin inhibition in a mouse model of SAH resulted in improved lumen patency and decreased peripheral WBC counts when compared with SAH controls.
Integrins
The main integrins involved in leukocyte adhesion and migration are LFA-1 and Mac-1. The authors have analyzed the effects of systemically administered anti-LFA-1 and Mac-1 monoclonal antibodies on morphometric arterial vasospasm in rats, rabbits, and monkeys after experimental SAH and found a significant decrease in posthemorrhagic vasospasm in all models, which correlated with fewer periadventitial infiltration of neutrophils and macrophages. Intracisternal monoclonal antibody administration in a rabbit model by Bavbek and colleagues produced similar results.
Statins are 3-hydroxy-3-methylglutaryl coenzyme A reductase inhibitors clinically used as cholesterol-reducing agents. Their ability to reduce the expression of proinflammatory cytokines and inhibit leukocyte integrins confers them potent antiinflammatory activity also. In a randomized controlled trial by Tseng and colleagues, patients with aSAH (n = 80) were randomized to receive either oral pravastatin or placebo within 72 hours of their initial hemorrhage. Patients treated with pravastatin had a 32% reduction in vasospasm incidence, vasospasm-related neurologic deficits decreased by 83%, and mortality decreased by 75% when compared with patients treated with placebo. A subsequent study by the same group found that pravastatin also improved neurologic outcomes at 6 months. In addition, a case-control series by Parra and colleagues showed decreased incidences of clinical vasospasm and improved 14-day functional outcomes in patients receiving statins before developing aSAH compared with patients who did not use statins. Kramer and colleagues and McGirt and colleagues, however, in recent retrospective studies did not find significant differences in the severity of angiographic or clinical vasospasm, or in the neurologic outcomes of patients receiving statins after aSAH.
Immunoglobulin superfamily proteins
Immunoglobulin superfamily proteins, such as ICAM1, have been found to be upregulated in patients who develop clinical vasospasm and increased expression correlates with poor neurologic outcomes following aSAH. The authors have shown that anti-ICAM1 monoclonal antibodies can decrease the extent of femoral artery vasospasm and inhibit periadventitial infiltration of macrophages and neutrophils in a rat model. This antibody produced a similar effect in basilar artery vasospasm and inflammatory cell infiltration as an anti-LFA-1 monoclonal antibody in animal models.
The use of drugs like statins and ibuprofen, which downregulate immunoglobulin superfamily expression, also decreases vasospasm in experimental studies. Several clinical studies have shown that statins decrease serum ICAM1 levels in hypercholesterolemic patients, and may contribute to the beneficial effects of statins in reducing the incidence of vasospasm in clinical trials. Ibuprofen is another antiinflammatory with anti-ICAM1 and antivascular cell adhesion molecule 1 (VCAM1) activity. Local sustained delivery of ibuprofen via controlled-release polymers significantly inhibited femoral artery vasospasm and decreased the number of periadventitial monocytes and macrophages when administered at 0 and 6 hours after hemorrhage in a rat model. These results were replicated in rabbit and monkey SAH models. Chyatte and colleagues also demonstrated that ibuprofen prevented ultrastructural changes in the cerebral vessel walls of dogs after blood injection. Clinical use of ibuprofen, however, is limited because its efficacy at preventing vasospasm has been shown only if it is administered within 6 hours of hemorrhage.
The critical role of leukocytes and inflammation in the pathophysiology of chronic vasospasm is widely supported by the experimental findings described earlier and by the efficacy of monoclonal antibodies against integrins and immunoglobulin superfamily CAMs in animal models. In the rabbit and primate SAH models, treatment with monoclonal antibodies against CAMs prevents leukocyte migration and vasospasm despite the presence of RBCs and Hgb in the subarachnoid space, which shows that chronic vasospasm does not occur in the absence of leukocytes or attenuated inflammation.
Other proinflammatory proteins
Cytokines and other proinflammatory proteins such as c-Jun N-terminal kinase (JNK) and poly (ADP-ribose) polymerase (PARP) have also been implicated in vasospasm. The main proinflammatory cytokines that have been shown to be elevated in patients with vasospasm include IL-1, IL-6, IL-8, and tumor necrosis factor α. Administration of drugs that inhibit cytokine production has resulted in attenuation of vasospasm in animal models. JNK is a mitogen-activated kinase involved in the inflammatory response. The use of a JNK inhibitor has been found to decrease angiographic vasospasm, improve neurologic function, reduce leukocyte infiltration, and decrease IL-6 production following blood injection in a canine model. PARP is a nuclear enzyme that regulates CAM expression and neutrophil recruitment during inflammation. In a rabbit model of SAH Satoh and colleagues showed that PARP activation occurred in the smooth muscles and adventitia of blood-exposed vessels, and that a PARP inhibitor decreased the severity of vasospasm.
NO Depletion and ET Elevation
Endothelium-derived relaxing factor or NO is synthesized in the blood vessel wall in response to shear stress or metabolic dysfunction, and results in significant arterial vasodilation. After hemorrhage, however, free Hgb disrupts several components of NO-mediated vasodilation. Besides inflammation, NO dysfunction is believed to play a contributory role in the development of posthemorrhagic vasospasm and has been a target in several experimental studies. Following aSAH, CSF levels of nitrites, a major source of endogenous NO, have been found to be significantly decreased in patients who develop vasospasm. The authors have shown that intrathecal NO supplementation via controlled-released polymers prevented vasospasm in rat and rabbit models of SAH, and that delayed polymer implantation 24 or 48 hours after SAH also ameliorated vasospasm. Several studies have also shown that selective intracerebral NO injection, intraventricular NO injection, and systemic nitrite infusions improved the severity or decreased the incidence of vasospasm experimental and clinical studies.
ET are powerful vasoconstrictors commonly expressed by vascular endothelial cells. Although several studies have documented significant intrathecal ET-1 level increases in aSAH patients that develop vasospasm, others have not. Anti-ET-1 monoclonal antibodies, anti-ET receptors antibodies, and ET activation enzyme inhibitors have been shown to decrease vasospasm in some, but not all studies ; therefore, additional studies are needed to clarify its role in the pathophysiology of chronic vasospasm.
Nonhemorrhagic vasospasm
Cerebral vasospasm seems to develop in other pathologic conditions that affect the CNS in the absence of aSAH, such as TBI, infectious meningitis, and after craniotomies. Inflammation also seems to contribute significantly to the development of vasospasm in these other conditions.
Reported incidences of vasospasm in patients after TBI have ranged from 25% to 40%, regardless of intracranial penetration. The incidence and the pathophysiology of post-TBI vasospasm closely resemble those of posthemorrhagic vasospasm. Post-TBI vasospasm is also biphasic; it has an acute and a chronic period, and its time course parallels posthemorrhagic vasospasm. Despite the limited experimental and clinical studies on post-TBI, these observations suggest that trauma or trauma-related hemorrhage triggers a perivascular inflammatory response that results in cerebral vasospasm.
In addition to posttraumatic vasospasm, it has been reported that vasospasm can develop in patients who have undergone craniotomies for nonvascular causes. Bejjani and colleagues reported a case of a 6-year-old girl who underwent a craniotomy for an intracranial schwannoma who later developed angiographically confirmed vasospasm. El Hendawy and colleagues reported 14 cases of vasospasm following craniotomies for intraaxial and extraaxial brain tumors, including gliomas and meningiomas. As with posttraumatic vasospasm, it is believed that the same mechanisms underlying post-aSAH vasospasm may explain postcraniotomy vasospasm.
Several cases of meningitis-associated cerebral vasospasm have been reported. The authors have shown that meningitis-associated vasospasm follows a time course similar to aSAH-associated vasospasm, and that its pathophysiology could also be explained by the inflammatory hypothesis. Following bacterial meningeal colonization and infection, endothelial activation signaling is triggered, leukocyte infiltration into the subarachnoid space occurs, and cytokines and other proinflammatory agents are released, which upregulate CAM expression, and enhance the inflammatory response that results in cerebral vasospasm.
Future directions
Despite numerous studies with promising experimental therapies for aSAH-induced vasospasm, hypertensive-hypervolemic-hemodilutional (“triple H”) therapy still remains as the mainstay of clinical vasospasm treatment. Large prospective controlled trials, however, have failed to show that prophylactic triple H therapy significantly reduces the incidence of clinical or angiographic vasospasm or that it improves neurologic outcomes. Additional treatments including transluminal balloon angioplasty, lumbar drainage of CSF, and intracisternal thrombolysis have been used as salvage therapies but they typically result in minimal benefits and increased complications. Pharmacologic therapies involving systemic calcium channel blockers (nimodipine), a nonglucocorticoid free radical scavenger (tirilazad mesilate), and intraarterial have suffered from these same limitations. Arterial narrowing was prevented effectively by nicardipine and clazosentan in clinical trials, but neurologic outcomes remained unchanged.
Limited progress has been made in the development of techniques to identify prospectively aSAH patients at risk for chronic cerebral vasospasm that would enable early and selective application of targeted therapies to prevent or ameliorate the inflammatory response and restore NO-mediated vasodilation. Among the potential molecular markers for predicting which patients will develop clinical vasospasm following aSAH, the Hp genotype has gained recent interest. Of all aSAH patients, 30% develop symptomatic vasospasm, 50% develop asymptomatic angiographic vasospasm, and 20% do not show signs of angiographic or clinical vasospasm. This distribution follows the prevalence of Hp genotypes in humans, and the Hp 2-2 genotype in particular seems to be present in 30% of humans, which correlates with the incidence of clinical vasospasm in aSAH patients. The authors have genetically engineered mice to express the Hp 2-2 genotype, and showed that Hp 2-2 mice developed more severe morphometric and clinical vasospasm after experimental SAH than wild-type Hp 1-1 mice. Vasospasm in these animals correlated with increased periadventitial neutrophils and macrophages, which strongly suggests a relationship between the Hp 2-2 genotype, inflammation, and cerebral vasospasm. Further studies are needed to clarify the relationship between the Hp genotype and inflammation, and only prospective clinical studies will define the effect of an Hp genotype in the development of chronic cerebral vasospasm.
Based on the substantial evidence on the contribution of inflammation to the pathophysiology of vasospasm presented, a hypothesis that links the various pathophysiologic events described in this review and elsewhere in the literature with the development of chronic vasospasm has been formulated: after aSAH, erythrocyte extravasation into the subarachnoid space induces endothelial upregulation of CAMs, primarily of ICAM1, which can also be upregulated by bacterial meningitis and traumatic SAH. ICAM1 upregulation enables endothelial cells to bind to LFA-1 or Mac-1 proteins on the leukocyte surface and mediate transendothelial leukocyte migration into the periadventitial space. Once in the subarachnoid space, extravasated leukocytes phagocytose subarachnoid erythrocytes in SAH or bacteria in bacterial meningitis. The absence of a lymphatic intrathecal system prevents leukocyte recirculation and trapped leukocytes die and degranulate in the subarachnoid space 2 to 4 days after the triggering event, which corresponds to the onset and time course of chronic vasospasm in humans. Leukocyte degranulation results in ET and oxygen free radicals release, and NO dysfunction. Although these molecular mechanisms are strongly amplified in Hp 2-2, they are moderately present in Hp 2-1 patients and lead to clinical vasospasm in Hp 2-2 patients, angiographic vasospasm in Hp 2-1 patients, and do not result in vasospasm in Hp 1-1 patients. Validation of this hypothesis will require extensive future testing in experimental models and clinical settings.
Summary
Delayed or chronic cerebral vasospasm results in major morbidity and mortality for patients after aSAH. Despite extensive clinical and experimental analysis of this phenomenon its pathophysiology remains poorly understood and the biologic and genetic principles behind the variability in the development of clinical vasospasm have not been elucidated. The cumulative evidence presented strongly supports the role that inflammation and leukocyte-endothelial cell interactions play in the pathophysiology of vasospasm, but translation of these findings into clinically effective therapies will require further molecular and genetic understanding of this inflammatory arteriopathy.
References
- 1. Kassell N.F., Torner J.C., Haley E.C., et al: The International Cooperative Study on the timing of aneurysm surgery. Part 1: overall management results. J Neurosurg 1990; 73: pp. 18
- 2. Kassell N.F., Torner J.C., Jane J.A., et al: The International Cooperative Study on the timing of aneurysm surgery. Part 2: surgical results. J Neurosurg 1990; 73: pp. 37
- 3. Solenski N.J., Haley E.C., Kassell N.F., et al: Medical complications of aneurysmal subarachnoid hemorrhage: a report of the multicenter, cooperative aneurysm study. Participants of the Multicenter Cooperative Aneurysm Study. Crit Care Med 1995; 23: pp. 1007
- 4. Suarez J.I., Tarr R.W., and Selman W.R.: Aneurysmal subarachnoid hemorrhage. N Engl J Med 2006; 354: pp. 387
- 5. Weir B., Grace M., Hansen J., et al: Time course of vasospasm in man. J Neurosurg 1978; 48: pp. 173
- 6. Haley E.C., Kassell N.F., Torner J.C., et al: A randomized trial of two doses of nicardipine in aneurysmal subarachnoid hemorrhage. A report of the Cooperative Aneurysm Study. J Neurosurg 1994; 80: pp. 788
- 7. Armin S.S., Colohan A.R., and Zhang J.H.: Traumatic subarachnoid hemorrhage: our current understanding and its evolution over the past half century. Neurol Res 2006; 28: pp. 445
- 8. Hamer J., and Krastel A.: Cerebral vasospasm after brain injury. Neurochirurgia (Stuttg) 1976; 19: pp. 185
- 9. Martin N.A., Doberstein C., Alexander M., et al: Posttraumatic cerebral arterial spasm. J Neurotrauma 1995; 12: pp. 897
- 10. Martin N.A., Doberstein C., Zane C., et al: Posttraumatic cerebral arterial spasm: transcranial Doppler ultrasound, cerebral blood flow, and angiographic findings. J Neurosurg 1992; 77: pp. 575
- 11. Pasqualin A., Vivenza C., Rosta L., et al: Cerebral vasospasm after head injury. Neurosurgery 1984; 15: pp. 855
- 12. Sander D., and Klingelhofer J.: Cerebral vasospasm following post-traumatic subarachnoid hemorrhage evaluated by transcranial Doppler ultrasonography. J Neurol Sci 1993; 119: pp. 1
- 13. Soustiel J.F., and Shik V.: Posttraumatic basilar artery vasospasm. Surg Neurol 2004; 62: pp. 201
- 14. Taneda M., Kataoka K., Akai F., et al: Traumatic subarachnoid hemorrhage as a predictable indicator of delayed ischemic symptoms. J Neurosurg 1996; 84: pp. 762
- 15. Zubkov A.Y., Lewis A.I., Raila F.A., et al: Risk factors for the development of post-traumatic cerebral vasospasm. Surg Neurol 2000; 53: pp. 126
- 16. Bejjani G.K., Duong D.H., Kalamarides M., et al: Cerebral vasospasm after tumor resection. A case report. Neurochirurgie 1997; 43: pp. 164
- 17. el Hendawy M., Wronski J., Juniewicz H., et al: Cerebral vasospasm detection by TCD after supratentorial brain tumours surgery. Neurol Neurochir Pol 2000; 34: pp. 114
- 18. Yamashima T., Kida S., and Yamamoto S.: An electron microscopic study of cerebral vasospasm with resultant myonecrosis in cases of subarachnoid haemorrhage, meningitis and trans-sylvian surgery. J Neurol 1986; 233: pp. 348
- 19. Chaichana K., Riley L.H., and Tamargo R.J.: Delayed cerebral vasospasm secondary to bacterial meningitis after lumbosacral spinal surgery: case report. Neurosurgery 2007; 60: pp. E206
- 20. Ferris E.J., Rudikoff J.C., and Shapiro J.H.: Cerebral angiography of bacterial infection. Radiology 1968; 90: pp. 727
- 21. Pfister H.W., Borasio G.D., Dirnagl U., et al: Cerebrovascular complications of bacterial meningitis in adults. Neurology 1992; 42: pp. 1497
- 22. Ries S., Schminke U., Fassbender K., et al: Cerebrovascular involvement in the acute phase of bacterial meningitis. J Neurol 1997; 244: pp. 51
- 23. Yamashima T., Kashihara K., Ikeda K., et al: Three phases of cerebral arteriopathy in meningitis: vasospasm and vasodilatation followed by organic stenosis. Neurosurgery 1985; 16: pp. 546
- 24. Gallia G.L., and Tamargo R.J.: Leukocyte-endothelial cell interactions in chronic vasospasm after subarachnoid hemorrhage. Neurol Res 2006; 28: pp. 750
- 25. Ascenzi P., Bocedi A., Visca P., et al: Hemoglobin and heme scavenging. IUBMB Life 2005; 57: pp. 749
- 26. Claassen J., Bernardini G.L., Kreiter K., et al: Effect of cisternal and ventricular blood on risk of delayed cerebral ischemia after subarachnoid hemorrhage: the Fisher scale revisited. Stroke 2001; 32: pp. 2012
- 27. Dietrich H.H., and Dacey R.G.: Molecular keys to the problems of cerebral vasospasm. Neurosurgery 2000; 46: pp. 517
- 28. Fisher C.M., Kistler J.P., and Davis J.M.: Relation of cerebral vasospasm to subarachnoid hemorrhage visualized by computerized tomographic scanning. Neurosurgery 1980; 6: pp. 1
- 29. Hijdra A., van Gijn J., Nagelkerke N.J., et al: Prediction of delayed cerebral ischemia, rebleeding, and outcome after aneurysmal subarachnoid hemorrhage. Stroke 1988; 19: pp. 1250
- 30. Nishizawa S., and Laher I.: Signaling mechanisms in cerebral vasospasm. Trends Cardiovasc Med 2005; 15: pp. 24
- 31. Qureshi A.I., Sung G.Y., Razumovsky A.Y., et al: Early identification of patients at risk for symptomatic vasospasm after aneurysmal subarachnoid hemorrhage. Crit Care Med 2000; 28: pp. 984
- 32. Doering T.J., Brix J., Schneider B., et al: Cerebral hemodynamics and cerebral metabolism during cold and warm stress. Am J Phys Med Rehabil 1996; 75: pp. 408
- 33. Rother R.P., Bell L., Hillmen P., et al: The clinical sequelae of intravascular hemolysis and extracellular plasma hemoglobin: a novel mechanism of human disease. JAMA 2005; 293: pp. 1653
- 34. Hooper D.C., Steer C.J., Dinarello C.A., et al: Haptoglobin and albumin synthesis in isolated rat hepatocytes. Response to potential mediators of the acute-phase reaction. Biochim Biophys Acta 1981; 653: pp. 118
- 35. Giblett E.R.: The haptoglobins of human serum. J Forensic Sci 1963; 8: pp. 446
- 36. McCormick D.J., and Atassi M.Z.: Hemoglobin binding with haptoglobin: delineation of the haptoglobin binding site on the alpha-chain of human hemoglobin. J Protein Chem 1990; 9: pp. 735
- 37. Wejman J.C., Hovsepian D., Wall J.S., et al: Structure and assembly of haptoglobin polymers by electron microscopy. J Mol Biol 1984; 174: pp. 343
- 38. Wejman J.C., Hovsepian D., Wall J.S., et al: Structure of haptoglobin and the haptoglobin-hemoglobin complex by electron microscopy. J Mol Biol 1984; 174: pp. 319
- 39. Bowman B.H., and Kurosky A.: Haptoglobin: the evolutionary product of duplication, unequal crossing over, and point mutation. Adv Hum Genet 1982; 12: pp. 189
- 40. Langlois M.R., and Delanghe J.R.: Biological and clinical significance of haptoglobin polymorphism in humans. Clin Chem 1996; 42: pp. 1589
- 41. Smithies O.: Zone electrophoresis in starch gels: group variations in the serum proteins of normal human adults. Biochem J 1955; 61: pp. 629
- 42. Javid J.: The effect of haptoglobin polymer size on hemoglobin binding capacity. Vox Sang 1965; 10: pp. 320
- 43. Asleh R., Guetta J., Kalet-Litman S., et al: Haptoglobin genotype- and diabetes-dependent differences in iron-mediated oxidative stress in vitro and in vivo. Circ Res 2005; 96: pp. 435
- 44. Blum S., Asaf R., Guetta J., et al: Haptoglobin genotype determines myocardial infarct size in diabetic mice. J Am Coll Cardiol 2007; 49: pp. 82
- 45. Lange V.: Anthropol Anz 1992; 50: pp. 281
- 46. Melamed-Frank M., Lache O., Enav B.I., et al: Structure-function analysis of the antioxidant properties of haptoglobin. Blood 2001; 98: pp. 3693
- 47. Serhan C.N.: Resolution phase of inflammation: novel endogenous anti-inflammatory and proresolving lipid mediators and pathways. Annu Rev Immunol 2007; 25: pp. 101
- 48. Serhan C.N., and Savill J.: Resolution of inflammation: the beginning programs the end. Nat Immunol 2005; 6: pp. 1191
- 49. Filer A., Raza K., Salmon M., et al: Targeting stromal cells in chronic inflammation. Discov Med 2007; 7: pp. 20
- 50. O’Shea J.J., and Murray P.J.: Cytokine signaling modules in inflammatory responses. Immunity 2008; 28: pp. 477
- 51. Springer T.A.: Traffic signals for lymphocyte recirculation and leukocyte emigration: the multistep paradigm. Cell 1994; 76: pp. 301
- 52. Ecker A., and Riemenschneider P.A.: Arteriographic demonstration of spasm of the intracranial arteries, with special reference to saccular arterial aneurysms. J Neurosurg 1951; 8: pp. 660
- 53. Gull W.M.: Cases of aneurism of the cerebral vessels. Guys Hosp Rep 1859; 5: pp. 281
- 54. Florey H.: Microscopical observations on the circulation of blood in the cerebral cortex. Brain 1925; 48: pp. 43
- 55. Jackson I.J.: Aseptic hemogenic meningitis. An experimental study of aseptic meningeal reactions due to blood and its breakdown products. Arch Neurol Psychiatry 1949; 62: pp. 572
- 56. Robertson E.G.: Cerebral lesions due to intracranial aneurysms. Brain 1949; 72: pp. 150
- 57. Chou W.H., Choi D.S., Zhang H., et al: Neutrophil protein kinase Cdelta as a mediator of stroke-reperfusion injury. J Clin Invest 2004; 114: pp. 49
- 58. Fisher C.M., Roberson G.H., and Ojemann R.G.: Cerebral vasospasm with ruptured saccular aneurysm–the clinical manifestations. Neurosurgery 1977; 1: pp. 245
- 59. Henker R., and Carlson K.K.: Fever: applying research to bedside practice. AACN Adv Crit Care 2007; 18: pp. 76
- 60. Rousseaux P., Scherpereel B., Bernard M.H., et al: Fever and cerebral vasospasm in ruptured intracranial aneurysms. Surg Neurol 1980; 14: pp. 459
- 61. Weir B., Disney L., Grace M., et al: Daily trends in white blood cell count and temperature after subarachnoid hemorrhage from aneurysm. Neurosurgery 1989; 25: pp. 161
- 62. Oliveira-Filho J., Ezzeddine M.A., Segal A.Z., et al: Fever in subarachnoid hemorrhage: relationship to vasospasm and outcome. Neurology 2001; 56: pp. 1299
- 63. Maiuri F., Gallicchio B., Donati P., et al: The blood leukocyte count and its prognostic significance in subarachnoid hemorrhage. J Neurosurg Sci 1987; 31: pp. 45
- 64. McGirt M.J., Mavropoulos J.C., McGirt L.Y., et al: Leukocytosis as an independent risk factor for cerebral vasospasm following aneurysmal subarachnoid hemorrhage. J Neurosurg 2003; 98: pp. 1222
- 65. Neil-Dwyer G., and Cruickshank J.: The blood leucocyte count and its prognostic significance in subarachnoid haemorrhage. Brain 1974; 97: pp. 79
- 66. Niikawa S., Hara S., Ohe N., et al: Correlation between blood parameters and symptomatic vasospasm in subarachnoid hemorrhage patients. Neurol Med Chir (Tokyo) 1997; 37: pp. 881
- 67. Spallone A., Acqui M., Pastore F.S., et al: Relationship between leukocytosis and ischemic complications following aneurysmal subarachnoid hemorrhage. Surg Neurol 1987; 27: pp. 253
- 68. Ostergaard J.R., Kristensen B.O., Svehag S.E., et al: Immune complexes and complement activation following rupture of intracranial saccular aneurysms. J Neurosurg 1987; 66: pp. 891
- 69. Pellettieri L., Carlson C.A., and Lindholm L.: Is the vasospasm following subarachnoidal hemorrhage an immunoreactive disease? Experientia 1981; 37: pp. 1170
- 70. Pellettieri L., Nilsson B., Carlsson C.A., et al: Serum immunocomplexes in patients with subarachnoid hemorrhage. Neurosurgery 1986; 19: pp. 767
- 71. Kasuya H., and Shimizu T.: Activated complement components C3a and C4a in cerebrospinal fluid and plasma following subarachnoid hemorrhage. J Neurosurg 1989; 71: pp. 741
- 72. Rothoerl R.D., Axmann C., Pina A.L., et al: Possible role of the C-reactive protein and white blood cell count in the pathogenesis of cerebral vasospasm following aneurysmal subarachnoid hemorrhage. J Neurosurg Anesthesiol 2006; 18: pp. 68
- 73. Crompton M.R.: The pathogenesis of cerebral infarction following the rupture of cerebral berry aneurysms. Brain 1964; 87: pp. 491
- 74. Hoshi T., Shimizu T., Kito K., et al: Neurol Med Chir (Tokyo) 1984; 24: pp. 647
- 75. Hughes J.T., and Schianchi P.M.: Cerebral artery spasm. A histological study at necropsy of the blood vessels in cases of subarachnoid hemorrhage. J Neurosurg 1978; 48: pp. 515
- 76. Ryba M., Jarzabek-Chorzelska M., Chorzelski T., et al: Is vascular angiopathy following intracranial aneurysm rupture immunologically mediated? Acta Neurochir (Wien) 1992; 117: pp. 34
- 77. Shimizu T., Kito K., Hoshi T., et al: Neurol Med Chir (Tokyo) 1982; 22: pp. 613
- 78. Walton J.N.: The prognosis and management of subarachnoid haemorrhage. Can Med Assoc J 1955; 72: pp. 165
- 79. Mori T., Nagata K., Ishida T., et al: Sequential morphological changes of the constrictive basilar artery in a canine model of experimental cerebral vasospasm by talc injection. J Vet Med Sci 1994; 56: pp. 535
- 80. Nagata K., Sasaki T., Mori T., et al: Cisternal talc injection in dog can induce delayed and prolonged arterial constriction resembling cerebral vasospasm morphologically and pharmacologically. Surg Neurol 1996; 45: pp. 442
- 81. Peterson J.W., Kwun B.D., Hackett J.D., et al: The role of inflammation in experimental cerebral vasospasm. J Neurosurg 1990; 72: pp. 767
- 82. Recinos P.F., Pradilla G., Thai Q.A., et al: Controlled release of lipopolysaccharide in the subarachnoid space of rabbits induces chronic vasospasm in the absence of blood. Surg Neurol 2006; 66: pp. 463
- 83. Yanamoto H., Kikuchi H., Okamoto S., et al: Cerebral vasospasm caused by cisternal injection of polystyrene latex beads in rabbits is inhibited by a serine protease inhibitor. Surg Neurol 1994; 42: pp. 374
- 84. Chyatte D.: Prevention of chronic cerebral vasospasm in dogs with ibuprofen and high-dose methylprednisolone. Stroke 1989; 20: pp. 1021
- 85. Chyatte D., Rusch N., and Sundt T.M.: Prevention of chronic experimental cerebral vasospasm with ibuprofen and high-dose methylprednisolone. J Neurosurg 1983; 59: pp. 925
- 86. Chyatte D., and Sundt T.M.: Response of chronic experimental cerebral vasospasm to methylprednisolone and dexamethasone. J Neurosurg 1984; 60: pp. 923
- 87. Handa Y., Hayashi M., Takeuchi H., et al: Effect of cyclosporine on the development of cerebral vasospasm in a primate model. Neurosurgery 1991; 28: pp. 380
- 88. Mori T., Nagata K., Ishida T., et al: FK-506: a new immunosuppressive agent, failed to reduce cerebral vasospasm after experimental subarachnoid hemorrhage. J Vet Med Sci 1993; 55: pp. 581
- 89. Nagata K., Sasaki T., Iwama J., et al: Failure of FK-506, a new immunosuppressant, to prevent cerebral vasospasm in a canine two-hemorrhage model. J Neurosurg 1993; 79: pp. 710
- 90. Nishizawa S., Peterson J.W., Shimoyama I., et al: Therapeutic effect of a new immunosuppressant, FK-506, on vasospasm after subarachnoid hemorrhage. Neurosurgery 1993; 32: pp. 986
- 91. Peterson J.W., Nishizawa S., Hackett J.D., et al: Cyclosporine A reduces cerebral vasospasm after subarachnoid hemorrhage in dogs. Stroke 1990; 21: pp. 133
- 92. White R.P., and Robertson J.T.: Comparison of piroxicam, meclofenamate, ibuprofen, aspirin, and prostacyclin efficacy in a chronic model of cerebral vasospasm. Neurosurgery 1983; 12: pp. 40
- 93. Chyatte D., Fode N.C., Nichols D.A., et al: Preliminary report: effects of high dose methylprednisolone on delayed cerebral ischemia in patients at high risk for vasospasm after aneurysmal subarachnoid hemorrhage. Neurosurgery 1987; 21: pp. 157
- 94. Hashi K., Takakura K., Sano K., et al: No To Shinkei 1988; 40: pp. 373
- 95. Manno E.M., Gress D.R., Ogilvy C.S., et al: The safety and efficacy of cyclosporine A in the prevention of vasospasm in patients with Fisher grade 3 subarachnoid hemorrhages: a pilot study. Neurosurgery 1997; 40: pp. 289
- 96. Ryba M., Pastuszko M., Iwanska K., et al: Cyclosporine A prevents neurological deterioration of patients with SAH–a preliminary report. Acta Neurochir (Wien) 1991; 112: pp. 25
- 97. Ryba M., Pastuszko M., Dziewiecki C., et al: A strategy for analyzing multiple parameters with application to aneurysmal SAH patients all of them clipped but treated with and without cyclosporine. Acta Neurochir (Wien) 1993; 122: pp. 194
- 98. Pozzesi N., Gizzi S., Gori F., et al: IL-2 induces and altered CD4/CD8 ratio of splenic T lymphocytes from transgenic mice overexpressing the glucocorticoid-induced protein GILZ. J Chemother 2007; 19: pp. 562
- 99. Elenkov I.J.: Glucocorticoids and the Th1/Th2 balance. Ann N Y Acad Sci 2004; 1024: pp. 138
- 100. Pritchard D.I.: Sourcing a chemical succession for cyclosporin from parasites and human pathogens. Drug Discov Today 2005; 10: pp. 688
- 101. Ryba M., Grieb P., Bidzinski J., et al: Cyclosporine A for the prevention of neurological deficit following subarachnoid hemorrhage. Stroke 1991; 22: pp. 531
- 102. Green G.A.: Understanding NSAIDs: from aspirin to COX-2. Clin Cornerstone 2001; 3: pp. 50
- 103. Kapiotis S., Sengoelge G., Sperr W.R., et al: Ibuprofen inhibits pyrogen-dependent expression of VCAM-1 and ICAM-1 on human endothelial cells. Life Sci 1996; 58: pp. 2167
- 104. Pradilla G., Thai Q.A., Legnani F.G., et al: Local delivery of ibuprofen via controlled-release polymers prevents angiographic vasospasm in a monkey model of subarachnoid hemorrhage. Neurosurgery 2005; 57: pp. 184
- 105. Thai Q.A., Oshiro E.M., and Tamargo R.J.: Inhibition of experimental vasospasm in rats with the periadventitial administration of ibuprofen using controlled-release polymers. Stroke 1999; 30: pp. 140
- 106. German J.W., Gross C.E., Giclas P., et al: Systemic complement depletion inhibits experimental cerebral vasospasm. Neurosurgery 1996; 39: pp. 141
- 107. Yanamoto H., Kikuchi H., Okamoto S., et al: Preventive effect of synthetic serine protease inhibitor, FUT-175, on cerebral vasospasm in rabbits. Neurosurgery 1992; 30: pp. 351
- 108. Kaminogo M., Yonekura M., Onizuka M., et al: Combination of serine protease inhibitor FUT-175 and thromboxane synthetase inhibitor OKY-046 decreases cerebral vasospasm in patients with subarachnoid hemorrhage. Neurol Med Chir (Tokyo) 1998; 38: pp. 704
- 109. Yanamoto H., Kikuchi H., Sato M., et al: Therapeutic trial of cerebral vasospasm with the serine protease inhibitor, FUT-175, administered in the acute stage after subarachnoid hemorrhage. Neurosurgery 1992; 30: pp. 358
- 110. Kansas G.S.: Selectins and their ligands: current concepts and controversies. Blood 1996; 88: pp. 3259
- 111. Campbell J.J., Qin S., Bacon K.B., et al: Biology of chemokine and classical chemoattractant receptors: differential requirements for adhesion-triggering versus chemotactic responses in lymphoid cells. J Cell Biol 1996; 134: pp. 255
- 112. Chan J.R., Hyduk S.J., and Cybulsky Ml: Chemoattractants induce a rapid and transient upregulation of monocyte alpha4 integrin affinity for vascular cell adhesion molecule 1 which mediates arrest: an early step in the process of emigration. J Exp Med 2001; 193: pp. 1149
- 113. Schenkel A.R., Mamdouh Z., and Muller W.A.: Locomotion of monocytes on endothelium is a critical step during extravasation. Nat Immunol 2004; 5: pp. 393
- 114. Lasky L.A.: Selectins: interpreters of cell-specific carbohydrate information during inflammation. Science 1992; 258: pp. 964
- 115. Rosen S.D.: Cell surface lectins in the immune system. Semin Immunol 1993; 5: pp. 237
- 116. Polin R.S., Bavbek M., Shaffrey M.E., et al: Detection of soluble E-selectin, ICAM-1, VCAM-1, and L-selectin in the cerebrospinal fluid of patients after subarachnoid hemorrhage. J Neurosurg 1998; 89: pp. 559
- 117. Nissen J.J., Mantle D., Blackburn A., et al: The selectin superfamily: the role of selectin adhesion molecules in delayed cerebral ischaemia after aneurysmal subarachnoid haemorrhage. Acta Neurochir Suppl 2000; 76: pp. 55
- 118. Nissen J.J., Mantle D., Gregson B., et al: Serum concentration of adhesion molecules in patients with delayed ischaemic neurological deficit after aneurysmal subarachnoid haemorrhage: the immunoglobulin and selectin superfamilies. J Neurol Neurosurg Psychiatry 2001; 71: pp. 329
- 119. Lin C.L., Dumont A.S., Calisaneller T., et al: Monoclonal antibody against E selectin attenuates subarachnoid hemorrhage-induced cerebral vasospasm. Surg Neurol 2005; 64: pp. 201
- 120. de Fougerolles A.R., Stacker S.A., Schwarting R., et al: Characterization of ICAM-2 and evidence for a third counter-receptor for LFA-1. J Exp Med 1991; 174: pp. 253
- 121. Springer T.A.: Adhesion receptors of the immune system. Nature 1990; 346: pp. 425
- 122. Clatterbuck R.E., Oshiro E.M., Hoffman P.A., et al: Inhibition of vasospasm with lymphocyte function-associated antigen-1 monoclonal antibody in a femoral artery model in rats. J Neurosurg 2002; 97: pp. 676
- 123. Pradilla G., Wang P.P., Legnani F.G., et al: Prevention of vasospasm by anti-CD11/CD18 monoclonal antibody therapy following subarachnoid hemorrhage in rabbits. J Neurosurg 2004; 101: pp. 88
- 124. Clatterbuck R.E., Gailloud P., Ogata L., et al: Prevention of cerebral vasospasm by a humanized anti-CD11/CD18 monoclonal antibody administered after experimental subarachnoid hemorrhage in nonhuman primates. J Neurosurg 2003; 99: pp. 376
- 125. Bavbek M., Polin R., Kwan A.L., et al: Monoclonal antibodies against ICAM-1 and CD18 attenuate cerebral vasospasm after experimental subarachnoid hemorrhage in rabbits. Stroke 1998; 29: pp. 1930
- 126. Ascer E., Bertolami M.C., Venturinelli M.L., et al: Atorvastatin reduces proinflammatory markers in hypercholesterolemic patients. Atherosclerosis 2004; 177: pp. 161
- 127. Chello M., Carassiti M., Agro F., et al: Simvastatin blunts the increase of circulating adhesion molecules after coronary artery bypass surgery with cardiopulmonary bypass. J Cardiothorac Vasc Anesth 2004; 18: pp. 605
- 128. Tseng M.Y., Czosnyka M., Richards H., et al: Effects of acute treatment with pravastatin on cerebral vasospasm, autoregulation, and delayed ischemic deficits after aneurysmal subarachnoid hemorrhage: a phase II randomized placebo-controlled trial. Stroke 2005; 36: pp. 1627
- 129. Tseng M.Y., Hutchinson P.J., Czosnyka M., et al: Effects of acute pravastatin treatment on intensity of rescue therapy, length of inpatient stay, and 6-month outcome in patients after aneurysmal subarachnoid hemorrhage. Stroke 2007; 38: pp. 1545
- 130. Parra A., Kreiter K.T., Williams S., et al: Effect of prior statin use on functional outcome and delayed vasospasm after acute aneurysmal subarachnoid hemorrhage: a matched controlled cohort study. Neurosurgery 2005; 56: pp. 476
- 131. Kramer A.H., Gurka M.J., Nathan B., et al: Statin use was not associated with less vasospasm or improved outcome after subarachnoid hemorrhage. Neurosurgery 2008; 62: pp. 422
- 132. McGirt M.J., Garces Ambrossi G.L., Huang J., et al: Simvastatin for the prevention of symptomatic cerebral vasospasm following aneurysmal subarachnoid hemorrhage: a single-institution prospective cohort study. J Neurosurg 2009; 110: pp. 968
- 133. Cheong J.H., Kim J.M., Bak K.H., et al: Correlation between cerebral vasospasm after subarachnoid hemorrhage and intracellular adhesion molecule-1 levels in serum and cerebrospinal fluid. J Korean Med Sci 2005; 38: pp. 1
- 134. Mack W.J., Mocco J., Hoh D.J., et al: Outcome prediction with serum intercellular adhesion molecule-1 levels after aneurysmal subarachnoid hemorrhage. J Neurosurg 2002; 96: pp. 71
- 135. Mocco J., Mack W.J., Kim G.H., et al: Rise in serum soluble intercellular adhesion molecule-1 levels with vasospasm following aneurysmal subarachnoid hemorrhage. J Neurosurg 2002; 97: pp. 537
- 136. Oshiro E.M., Hoffman P.A., Dietsch G.N., et al: Inhibition of experimental vasospasm with anti-intercellular adhesion molecule-1 monoclonal antibody in rats. Stroke 1997; 28: pp. 2031
- 137. Doo Y.C., Han S.J., Han S.W., et al: Effect of preexisting statin use on expression of C-reactive protein, adhesion molecules, interleukin-6, and antioxidized low-density lipoprotein antibody in patients with unstable angina undergoing coronary stenting. Clin Cardiol 2005; 28: pp. 72
- 138. Koh K.K., Son J.W., Ahn J.Y., et al: Vascular effects of diet and statin in hypercholesterolemic patients. Int J Cardiol 2004; 95: pp. 185
- 139. Rezaie-Majd A., Prager G.W., Bucek R.A., et al: Simvastatin reduces the expression of adhesion molecules in circulating monocytes from hypercholesterolemic patients. Arterioscler Thromb Vasc Biol 2003; 23: pp. 397
- 140. Lynch J.R., Wang H., McGirt M.J., et al: Simvastatin reduces vasospasm after aneurysmal subarachnoid hemorrhage: results of a pilot randomized clinical trial. Stroke 2005; 36: pp. 2024
- 141. Hofbauer R., Frass M., Gmeiner B., et al: Rapid, fluorescence-based assay for microtiter plates to test drug influences on neutrophil transmigration through endothelial cell monolayers. Life Sci 1999; 65: pp. 2453
- 142. Frazier J.L., Pradilla G., Wang P.P., et al: Inhibition of cerebral vasospasm by intracranial delivery of ibuprofen from a controlled-release polymer in a rabbit model of subarachnoid hemorrhage. J Neurosurg 2004; 101: pp. 93
- 143. Aihara Y., Kasuya H., Onda H., et al: Quantitative analysis of gene expressions related to inflammation in canine spastic artery after subarachnoid hemorrhage. Stroke 2001; 32: pp. 212
- 144. Fassbender K., Hodapp B., Rossol S., et al: Inflammatory cytokines in subarachnoid haemorrhage: association with abnormal blood flow velocities in basal cerebral arteries. J Neurol Neurosurg Psychiatry 2001; 70: pp. 534
- 145. Gaetani P., Tartara F., Pignatti P., et al: Cisternal CSF levels of cytokines after subarachnoid hemorrhage. Neurol Res 1998; 20: pp. 337
- 146. Hendryk S., Jarzab B., and Josko J.: Increase of the IL-1 beta and IL-6 levels in CSF in patients with vasospasm following aneurysmal SAH. Neuro Endocrinol Lett 2004; 25: pp. 141
- 147. Nam D.H., Kim J.S., Hong S.C., et al: Expression of interleukin-1 beta in lipopolysaccharide stimulated monocytes derived from patients with aneurysmal subarachnoid hemorrhage is correlated with cerebral vasospasm. Neurosci Lett 2001; 312: pp. 41
- 148. Schoch B., Regel J.P., Wichert M., et al: Analysis of intrathecal interleukin-6 as a potential predictive factor for vasospasm in subarachnoid hemorrhage. Neurosurgery 2007; 60: pp. 828
- 149. Wang Y., Zhong M., Tan X.X., et al: Expression change of interleukin-8 gene in rabbit basilar artery after subarachnoid hemorrhage. Neurosci Bull 2007; 23: pp. 151
- 150. Bowman G., Bonneau R.H., Chinchilli V.M., et al: A novel inhibitor of inflammatory cytokine production (CNI-1493) reduces rodent post-hemorrhagic vasospasm. Neurocrit Care 2006; 5: pp. 222
- 151. Bowman G., Dixit S., Bonneau R.H., et al: Neutralizing antibody against interleukin-6 attenuates posthemorrhagic vasospasm in the rat femoral artery model. Neurosurgery 2004; 54: pp. 719
- 152. Manning A.M., and Davis R.J.: Targeting JNK for therapeutic benefit: from junk to gold? Nat Rev Drug Discov 2003; 2: pp. 554
- 153. Yatsushige H., Yamaguchi M., Zhou C., et al: Role of c-Jun N-terminal kinase in cerebral vasospasm after experimental subarachnoid hemorrhage. Stroke 2005; 36: pp. 1538
- 154. Cuzzocrea S.: Shock, inflammation and PARP. Pharmacol Res 2005; 52: pp. 72
- 155. Satoh M., Date I., Nakajima M., et al: Inhibition of poly(ADP-ribose) polymerase attenuates cerebral vasospasm after subarachnoid hemorrhage in rabbits. Stroke 2001; 32: pp. 225
- 156. Pluta R.M.: Dysfunction of nitric oxide synthases as a cause and therapeutic target in delayed cerebral vasospasm after SAH. Neurol Res 2006; 28: pp. 730
- 157. Cosby K., Partovi K.S., Crawford J.H., et al: Nitrite reduction to nitric oxide by deoxyhemoglobin vasodilates the human circulation. Nat Med 2003; 9: pp. 1498
- 158. Weyerbrock A., Walbridge S., Pluta R.M., et al: Selective opening of the blood-tumor barrier by a nitric oxide donor and long-term survival in rats with C6 gliomas. J Neurosurg 2003; 99: pp. 728
- 159. Pluta R.M.: Delayed cerebral vasospasm and nitric oxide: review, new hypothesis, and proposed treatment. Pharmacol Ther 2005; 105: pp. 23
- 160. Pluta R.M., Dejam A., Grimes G., et al: Nitrite infusions to prevent delayed cerebral vasospasm in a primate model of subarachnoid hemorrhage. JAMA 2005; 293: pp. 1477
- 161. Gabikian P., Clatterbuck R.E., Eberhart C.G., et al: Prevention of experimental cerebral vasospasm by intracranial delivery of a nitric oxide donor from a controlled-release polymer: toxicity and efficacy studies in rabbits and rats. Stroke 2002; 33: pp. 2681
- 162. Pradilla G., Thai Q.A., Legnani F.G., et al: Delayed intracranial delivery of a nitric oxide donor from a controlled-release polymer prevents experimental cerebral vasospasm in rabbits. Neurosurgery 2004; 55: pp. 1393
- 163. Pluta R.M., Oldfield E.H., and Boock R.J.: Reversal and prevention of cerebral vasospasm by intracarotid infusions of nitric oxide donors in a primate model of subarachnoid hemorrhage. J Neurosurg 1997; 87: pp. 746
- 164. Egemen N., Turker R.K., Sanlidilek U., et al: The effect of intrathecal sodium nitroprusside on severe chronic vasospasm. Neurol Res 1993; 15: pp. 310
- 165. Inoue A., Yanagisawa M., Kimura S., et al: The human endothelin family: three structurally and pharmacologically distinct isopeptides predicted by three separate genes. Proc Natl Acad Sci U S A 1989; 86: pp. 2863
- 166. Sakurai T., Yanagisawa M., Inoue A., et al: cDNA cloning, sequence analysis and tissue distribution of rat preproendothelin-1 mRNA. Biochem Biophys Res Commun 1991; 175: pp. 44
- 167. Mascia L., Fedorko L., Stewart D.J., et al: Temporal relationship between endothelin-1 concentrations and cerebral vasospasm in patients with aneurysmal subarachnoid hemorrhage. Stroke 2001; 32: pp. 1185
- 168. Seifert V., Loffler B.M., Zimmermann M., et al: Endothelin concentrations in patients with aneurysmal subarachnoid hemorrhage. Correlation with cerebral vasospasm, delayed ischemic neurological deficits, and volume of hematoma. J Neurosurg 1995; 82: pp. 55
- 169. Hamann G., Isenberg E., Strittmatter M., et al: Absence of elevation of big endothelin in subarachnoid hemorrhage. Stroke 1993; 24: pp. 383
- 170. Yamaura I., Tani E., Maeda Y., et al: Endothelin-1 of canine basilar artery in vasospasm. J Neurosurg 1992; 76: pp. 99
- 171. Clozel M., Breu V., Burri K., et al: Pathophysiological role of endothelin revealed by the first orally active endothelin receptor antagonist. Nature 1993; 365: pp. 759
- 172. Zuccarello M., Boccaletti R., Romano A., et al: Endothelin B receptor antagonists attenuate subarachnoid hemorrhage-induced cerebral vasospasm. Stroke 1998; 29: pp. 1924
- 173. Matsumura Y., Ikegawa R., Suzuki Y., et al: Phosphoramidon prevents cerebral vasospasm following subarachnoid hemorrhage in dogs: the relationship to endothelin-1 levels in the cerebrospinal fluid. Life Sci 1991; 49: pp. 841
- 174. Cosentino F., McMahon E.G., Carter J.S., et al: Effect of endothelinA-receptor antagonist BQ-123 and phosphoramidon on cerebral vasospasm. J Cardiovasc Pharmacol 1993; 8: pp. S332
- 175. Kordestani R.K., Counelis G.J., McBride D.Q., et al: Cerebral arterial spasm after penetrating craniocerebral gunshot wounds: transcranial Doppler and cerebral blood flow findings. Neurosurgery 1997; 41: pp. 351
- 176. Oertel M., Boscardin W.J., Obrist W.D., et al: Posttraumatic vasospasm: the epidemiology, severity, and time course of an underestimated phenomenon: a prospective study performed in 299 patients. J Neurosurg 2005; 103: pp. 812
- 177. Dulkerian S.J., Kilpatrick L., Costarino A.T., et al: Cytokine elevations in infants with bacterial and aseptic meningitis. J Pediatr 1995; 126: pp. 872
- 178. Fassbender K., Ries S., Schminke U., et al: Inflammatory cytokines in CSF in bacterial meningitis: association with altered blood flow velocities in basal cerebral arteries. J Neurol Neurosurg Psychiatry 1996; 61: pp. 57
- 179. Fassbender K., Schminke U., Ries S., et al: Endothelial-derived adhesion molecules in bacterial meningitis: association to cytokine release and intrathecal leukocyte-recruitment. J Neuroimmunol 1997; 74: pp. 130
- 180. Mukai A.O., Krebs V.L., Bertoli C.J., et al: TNF-alpha and IL-6 in the diagnosis of bacterial and aseptic meningitis in children. Pediatr Neurol 2006; 34: pp. 25
- 181. Sethi S., Sharma P., and Dikshit M.: Nitric oxide- and oxygen-derived free radical generation from control and lipopolysaccharide-treated rat polymorphonuclear leukocyte. Nitric Oxide 2001; 5: pp. 482
- 182. van Deuren M., van der Ven-Jongekrijg J., Bartelink A.K., et al: Correlation between proinflammatory cytokines and antiinflammatory mediators and the severity of disease in meningococcal infections. J Infect Dis 1995; 172: pp. 433
- 183. Pigott R., Dillon L.P., Hemingway I.H., et al: Soluble forms of E-selectin, ICAM-1 and VCAM-1 are present in the supernatants of cytokine activated cultured endothelial cells. Biochem Biophys Res Commun 1992; 187: pp. 584
- 184. Egge A., Waterloo K., Sjoholm H., et al: Prophylactic hyperdynamic postoperative fluid therapy after aneurysmal subarachnoid hemorrhage: a clinical, prospective, randomized, controlled study. Neurosurgery 2001; 49: pp. 593
- 185. Feigin V.L., Rinkel G.J., Algra A., et al: Calcium antagonists in patients with aneurysmal subarachnoid hemorrhage: a systematic review. Neurology 1998; 50: pp. 876
- 186. Suarez J.I., Shannon L., Zaidat O.O., et al: Effect of human albumin administration on clinical outcome and hospital cost in patients with subarachnoid hemorrhage. J Neurosurg 2004; 100: pp. 585
- 187. Keyrouz S.G., and Diringer M.N.: Clinical review: prevention and therapy of vasospasm in subarachnoid hemorrhage. Crit Care 2007; 11: pp. 220
- 188. Rinkel G.J., Feigin V.L., Algra A., et al: Circulatory volume expansion therapy for aneurysmal subarachnoid haemorrhage. Cochrane Database Syst Rev 2004; undefined:
- 189. Hoh B.L., and Ogilvy C.S.: Endovascular treatment of cerebral vasospasm: transluminal balloon angioplasty, intra-arterial papaverine, and intra-arterial nicardipine. Neurosurg Clin N Am 2005; 16: pp. 501
- 190. Muizelaar J.P., Zwienenberg M., Rudisill N.A., et al: The prophylactic use of transluminal balloon angioplasty in patients with Fisher Grade 3 subarachnoid hemorrhage: a pilot study. J Neurosurg 1999; 91: pp. 51
- 191. Kinouchi H., Ogasawara K., Shimizu H., et al: Prevention of symptomatic vasospasm after aneurysmal subarachnoid hemorrhage by intraoperative cisternal fibrinolysis using tissue-type plasminogen activator combined with continuous cisternal drainage. Neurol Med Chir (Tokyo) 2004; 44: pp. 569
- 192. Klimo P., Kestle J.R., MacDonald J.D., et al: Marked reduction of cerebral vasospasm with lumbar drainage of cerebrospinal fluid after subarachnoid hemorrhage. J Neurosurg 2004; 100: pp. 215
- 193. Findlay J.M., Kassell N.F., Weir B.K., et al: A randomized trial of intraoperative, intracisternal tissue plasminogen activator for the prevention of vasospasm. Neurosurgery 1995; 37: pp. 168
- 194. Pickard J.D., Murray G.D., Illingworth R., et al: Effect of oral nimodipine on cerebral infarction and outcome after subarachnoid haemorrhage: British aneurysm nimodipine trial. BMJ 1989; 298: pp. 636
- 195. Haley E.C., Kassell N.F., Apperson-Hansen C., et al: A randomized, double-blind, vehicle-controlled trial of tirilazad mesylate in patients with aneurysmal subarachnoid hemorrhage: a cooperative study in North America. J Neurosurg 1997; 86: pp. 467
- 196. Kassell N.F., Haley E.C., Apperson-Hansen C., et al: Randomized, double-blind, vehicle-controlled trial of tirilazad mesylate in patients with aneurysmal subarachnoid hemorrhage: a cooperative study in Europe, Australia, and New Zealand. J Neurosurg 1996; 84: pp. 221
- 197. Polin R.S., Hansen C.A., German P., et al: Intra-arterially administered papaverine for the treatment of symptomatic cerebral vasospasm. Neurosurgery 1998; 42: pp. 1256
- 198. Vajkoczy P., Horn P., Bauhuf C., et al: Effect of intra-arterial papaverine on regional cerebral blood flow in hemodynamically relevant cerebral vasospasm. Stroke 2001; 32: pp. 498
- 199. Kasuya H., Onda H., Sasahara A., et al: Application of nicardipine prolonged-release implants: analysis of 97 consecutive patients with acute subarachnoid hemorrhage. Neurosurgery 2005; 56: pp. 895
- 200. Vajkoczy P., Meyer B., Weidauer S., et al: Clazosentan (AXV-034343), a selective endothelin A receptor antagonist, in the prevention of cerebral vasospasm following severe aneurysmal subarachnoid hemorrhage: results of a randomized, double-blind, placebo-controlled, multicenter phase IIa study. J Neurosurg 2005; 103: pp. 9
- 201. Chaichana K.L., Levy A.P., Miller-Lotan R., et al: Haptoglobin 2-2 genotype determines chronic vasospasm after experimental subarachnoid hemorrhage. Stroke 2007; 38: pp. 3266
- 202. Dorsch N.W.: Cerebral arterial spasm–a clinical review. Br J Neurosurg 1995; 9: pp. 403
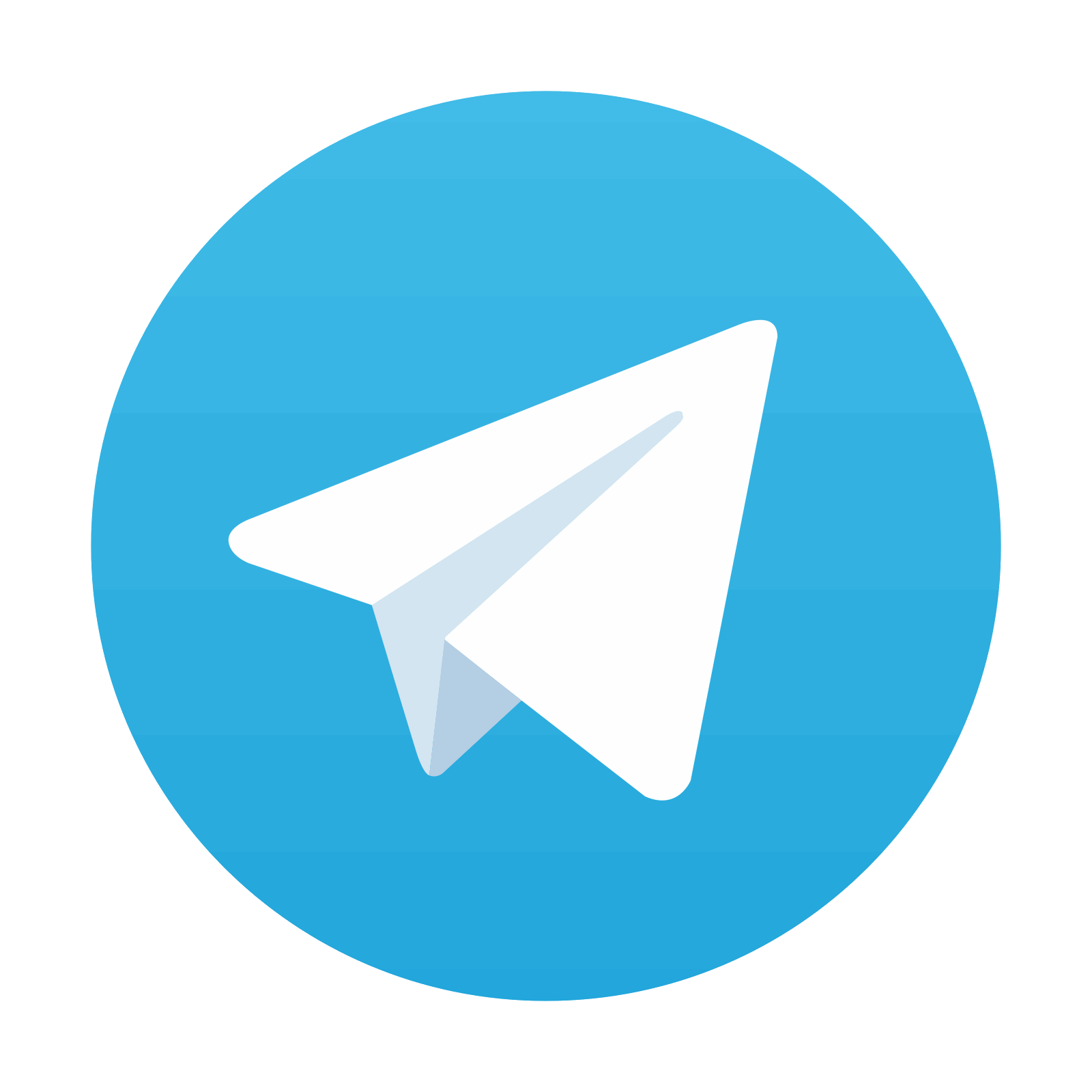
Stay updated, free articles. Join our Telegram channel

Full access? Get Clinical Tree
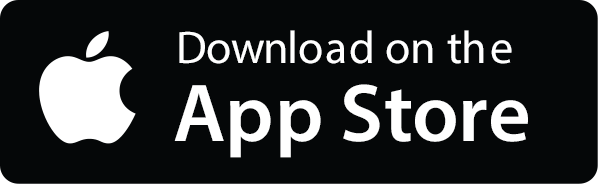
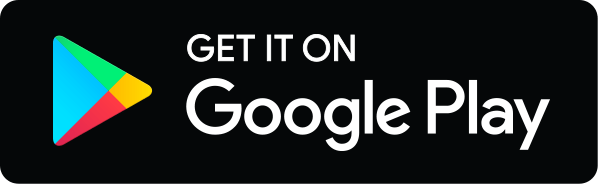