Study reference/principal investigator
Study title
Route
Cell type/source
Recipient species
Therapeutic window
Dose
Outcome measures
Follow-up/outcome measurements
Results summary
[10]
Treatment of stroke in rat with intracarotid administration of marrow stromal cells
IA
MSC/allogenic
Rat
1 day after ischemia
2 × 106
Neurological severity scores, Adhesive-removal test
At 1, 7, and 14 days after MCA
Homing of MSCs to ipsilateral MCA territory and functional improvement
[11
Intracarotid transplantation of bone marrow stromal cells increases axon-myelin remodeling after stroke
IA
Rat MSC/allogenic
Rat
After 24 h
2 x 106
Infarct volume, mNSS, adhesive removal
1, 7, 14, 21 and 28 days after MCA
Functional neurological improvement with treatment. Behavioral benefit is partly related to axon and myelin remodeling in the brain
[12]
Bone marrow stromal cells upregulate expression of bone morphogenetic proteins 2 and 4, gap junction protein connexin-43 and synaptophysin after stroke in rats
IA
Rat MSC/allogenic
Rat
At 24 h after MCAo
2 x 106
Infarct volume, mNSS, adhesive removal
Baseline before MCA, one day after MCAo, imme-diately prior to BMSC administration and at 7, 14, 21, and at 28 days after MCAo
Significantly improved functional neurological recovery in rats at 14 days, 21 and 28 days after MCAo, without any significant decrease in lesion volume
[13]
Brain protection using autologous bone marrow cell, metalloproteinase inhibitors, and metabolic treatment in cerebral ischemia
IA
Rat MSC/autologous
Rat
6 h after MCA
2 x 107
Infarct volume, rotarod
Rotarod at 2 hr after MCAo to 14 days
Combined treatment of BMCs with TIMPs and metabolic supplementation enhances neurogenesis and angiogenesis
[14]
One-year follow-up after bone marrow stromal cell treatment in middle-aged female rats with stroke
IA
Rat MSC/allogenic
Rat
At 1 day after stroke
2 x 106
Infarct volume, mNSS, adhesive removal
1 day, 2 weeks, 4 weeks, and then monthly
Improved neurological outcomes from 2 weeks through 1 year after BMSC injection
[15]
Intra-arterial transplantation of bone marrow mononuclear cells immediately after reperfusion decreases brain injury after focal ischemia in rats
IA/IV
BM-MNCs/autologous
Rat
Immediately after reperfusion(90 min)
1 × 107
Infarct Volume, Motor function
24 h or 7 days after reperfusion
IA superior to IV
Infarct volume decreased in the IA group, but not in the IV group
[16]
Intra-arterially delivered human umbilical cord blood-derived mesenchymal stem cells in canine cerebral ischemia
IA
MSC/Xenogenic
Canine
1 day after ischemia
1×106
Neurological evaluation, MR imaging
1,7, 10, and 22 days until 4 weeks
Improved infarction volume (MRI) at one week and early recovery from neurological deficits
[17]
Functional recovery after hematic administration of allogenic mesenchymal stem cells in acute ischemic stroke in rats
IA/IV
Rat MSC/allogenic
Rat
30 min after CC reperfusion
2 x 106
Infarct volume, MRI analysis of migration and implantation of stem cells
24 h and 14 days
IA equal to IV route in improving neurological recovery, and decreasing cerebral damage
[18]
In vivo MR imaging of intra-arterially delivered magnetically labeled mesenchymal stem cells in a canine stroke model
IA
MSC/autologous
Canine
At 1 week
3 × 106
MR imaging
In-vivo MRI images before cell grafting, one and 24 h after transplantation and weekly thereafter until 4 weeks
In vivo MR imaging is useful for tracking IA delivered MSCs after superparamagnetic iron oxide labeling
[19]
Intra-arterial cell transplantation provides timing-dependent cell distribution and functional recovery after stroke
IA
Human MSCs/xenogenic
Rat
At 1, 4, or 7 days after MCA
1 x 106
Behaviour test, histology
0, 1, 4, 7, 14, and 21 days after stroke
Improved motor function at day 1 and 4 but not day 7
[20]
Intracarotid transplantation of autologous adipose-derived mesenchymal stem cells significantly improves neurological deficits in rats after MCA
IA
Adipose-derived MSCs/autologous
Rat
At day 3 after MCA
2 x 106
Behaviour test, adhesive-removal test
1, 7, 14, 21 and 28 days after MCAo
No significant difference in infarct volume. Improved neurological and behavior scores 14 to day 28 after MCAo in treatment group
[21]
Intra-arterial delivery of human bone marrow mesenchymal stem cells is a safe and effective way to treat cerebral ischemia in rats
IA / IV
Human BM-derived MSCs/xenogenic
Rat
At 24 h
3 × 106
Functional outcome, LDF, SPECT, PET
1, 3, 7, 14, and 28 days after transplantation
IA route promoted angiogenesis and improved functional recovery compared to IV
[56]
Human bone marrow mesenchymal stem/stromal cells produce efficient localization in the brain and enhanced angiogenesis after intra-arterial delivery in rats with cerebral ischemia, but this is not translated to behavioral recovery
IA
Human BM-derived MSCs/xenogenic
Rat
At day 2 or 7
1 × 106
Cylinder test, sticky label test
At 6, 21 and 42 days
No improvement in functional recovery despite effective initial homing to the ischemic hemisphere and enhanced angiogenesis
[22]
Efficacy and dose-dependent safety of intra-arterial delivery of mesenchymal stem cells in a rodent stroke model
IA
MSCs
Rat
1 and 24 h after reperfusion/MCA model
5 x 104 to 1 x 106
Infarct size and neurological evaluation
1 day through 28 days
Improved infarct size and neurological scores. Optimal dose calculated at 1 x 105
Types of Stem Cells
Stem cells can be obtained from myriad sources. They can differentiate into diverse cell populations. Pluripotent stem cells, such as embryonic stem cells, are more potent with the ability to generate multiple cell types compared to multipotent stem cells that are derived from human tissue and differentiate into only mature cells [23]. However, the pluripotent stem cells have the drawback of tumorigenicity and forming potential teratomas [24]. Stem cells are also produced endogenously in response to tissue injury and can migrate to the ischemic lesions. Preclinical studies by various groups have shown that stem cells can act through multiple mechanisms including but not limited to neuroprotection, neurogenesis, angiogenesis, synaptogenesis, immunomodulation, release of growth, and trophic factors [25, 26].
Leong et al. in an extensive review of preclinical studies using stem cells in rodent models of ischemic stroke (most commonly used species) noted that among different types of stem cells, mesenchymal stem cells (MSCs, also called mesenchymal stem cell) and neuronal stem/progenitor cells reported most significant improvements in functional outcomes [27]. A recent meta-analysis of 46 preclinical studies of MSCs in ischemic stroke by Vu et al. suggested very promising results [28]. In their meta-analysis, they concluded that MSCs showed improved outcomes across various animal species, time of delivery, degree of immunogenicity, total dose/concentration, and in the presence of other comorbidities. Intracerebral (ICR) administration route was better than IA which in turn was better than IV route. ICR administration, however, is an invasive procedure compared to the alternative IA and IV modes of delivery. In our latest preclinical studies, we have shown the efficacy of IA delivery of MSCs and its superiority over the IV route in a rodent model of ischemic stroke [22]. The pros and cons of all routes of administration of stem cells are discussed in subsequent subsections. The meta-analysis by Vu et al. also suggested that MSCs used in primates showed most improvement in effect sizes compared to rodent and murine models [28]. The easy procurability and harvesting of MSCs, and improvement in outcomes after administration in various species and animal models of ischemic stroke, have been supported by various groups [29, 30]. MSCs can be obtained with relative ease; their procurement is not hampered by ethical considerations, and thus they have several advantages over other cell types.
There are various sources for MSCs, including but not limited to bone marrow (BM), umbilical cord blood cells (UCBCs), stem cells/progenitor cells derived from adipose tissue, stem cells derived from placenta [31], and human adult dental pulp [32]. UCBCs are pluripotent and are a source for MSCs. They are considered to have high regenerative potential and their naive immunologic phenotype makes them preferable for transplantation. Human adult dental pulp stem cells (DPSCs) that are derived from molar teeth are multipotent and have the capacity to differentiate into neurons. A preclinical study showed improved neurological outcomes with DPSCs in stroke animal model [32]. Olfactory ensheathing cells and endogenous stem cells from the subventricular zone (SVZ) and hippocampus are other potential therapies shown in preclinical studies for ischemic stroke [27, 33, 34].
Signaling Mechanisms
Complex signaling cascades and processes like vascular rolling, adhesion, endothelial transmigration, and migration through the extracellular space to the injury site are needed to recruit stem cells to the area of brain ischemia [35, 36]. Myriad chemical messengers are implicated in these processes. The cytokine stroma-derived factor (SDF)-1 from vascular endothelium and activated platelets acts on CXCR4 chemokine receptor on MSCs and influences the MSC mobilization and homing [37–41]. SDF-1 is regulated partially by the transcription modulator, hypoxia-inducible factor (HIF-1α) [42], matrix metalloproteinases (MMP), and nitric oxide (NO) [40, 41, 43–45]. Other chemokines like monocyte chemoattractant protein-1 (MCP-1) are also reported to have a role in the recruitment of MSCs [38]. Growth factors such as vascular endothelial growth factor (VEGF) and granulocyte colony-stimulating factor (G-CSF) are implicated in BM stem cell mobilization. G-CSF also promotes mobilization of hematopoietic stem cells (HSCs) and endothelial progenitor cells (EPCs) by decreasing SDF-1 expression in BM and CXCR4 expression on HSC [46–50].
Preclinical studies in stroke models suggest that MSCs themselves do not differentiate into neurons in vivo, however, promote differentiation of endogenous neural progenitor cells [27]. MSCs can secrete neurotrophic factors such as brain-derived neurotrophic factor (BDNF), basic fibroblast growth factor (bFGF), angiopoietin-2, transforming growth factor (TGF-B), and insulin-like growth factor (IGF-1). These factors promote the remodeling of the neurons, glia, and neurovascular cells, and enhance axonal sprouting and synaptogenesis [11]. MSCs also decrease neuronal apoptosis and activation of astrocytes to minimize scar formation, and promote angiogenesis by secreting VEGF, bFGF, nerve growth factor (NGF), and other angiogenic factors [13, 51]. Furthermore, MSCs also influence immunomodulation via TGF-B, NO and prostaglandin pathways, JAK-STAT signaling pathways and release of interleukin-6 [51]. Thus, stem cells could be considered to have pleiotropic effects by modulating numerous targets through multiple signaling cascades.
Radioimaging studies can be very helpful in tracking and localization of tracer-labeled stem cells which in turn can help in understanding homing mechanisms. Preclinical studies on rodent models [52, 53] as well as canine model [18] showed that bioluminescence imaging and superparamagnetic iron oxide labeling helped to track and localize IA-delivered stem cells to the ischemic areas after stroke. Other groups [16] also demonstrated the feasibility of magnetic resonance imaging (MRI) in canine model of ischemic stroke.
Therapeutic Window for Stem Cell Therapy in Ischemic Stroke
Therapies provided in the early hours after ischemic stroke are aimed at reducing the injury/limiting the infarct size. On the other hand, therapies started weeks to months after stroke are aimed at promoting remodeling and repair. MSCs and other stem cells may have potential for wide-ranging therapeutic time windows [27, 32, 54]. A compromised BBB after ischemic stroke may allow better delivery of the MSCs to the target ischemic lesion. On the other hand, the BBB may be intact up to 24 h after stroke and may deter the engraftment of target tissue. Similarly, in the chronic phase, remnant glial scar tissue is likely to obstruct the passage of stem cells [27].
We showed that the therapeutic window lasts at least 24 h in our preclinical studies using a rodent model of ischemic stroke [22] (Fig. 6.1). One benefit of early infusion of stem cells is that the inflammatory chemokines that are released after ischemic stroke can help in attracting the stem cells. Some researchers suggested that the effect of MSCs may be reduced if provided 7 days after stroke [55]. However, there are other preclinical IA studies that have shown that stem cell treatment showed significant enhancement of functional recovery when given up to 7 days after ischemic stroke [19] and increased angiogenesis [56]. It is understandable that preclinical studies mostly focused on early therapeutic window in order to demonstrate better outcomes. Nonetheless, the preclinical studies demonstrate a wide therapeutic window for stem cells compared to existing standard of care therapies. On the other hand, some preclinical studies have unequivocally demonstrated that the beneficial effects of stem cells extend for a long duration from 4 months [32] up to a year [14] from the time of administration after ischemic stroke. This is very encouraging for clinical trials, if these time frames in rodent models were converted to human years.
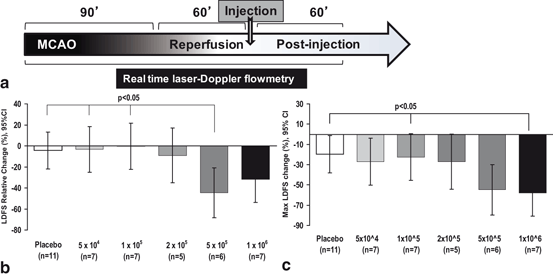
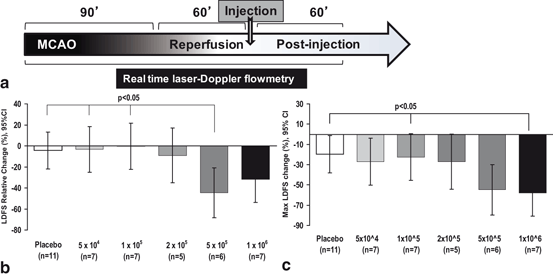
Fig. 6.1
Lower doses of IC MSCs mitigate adverse effect IC injection on MCA blood flow. (a) Experimental timeline showing rMCAo for 90 minutes followed by withdrawal of the suture to allow reperfusion. At 60 minutes of reperfusion, IC MSC or vehicle only injection was given, followed by LDF monitoring for 60 min. (b) Comparison of relative LDFS worsening from baseline to final recording, among de-escalating dose groups (c) The 1 x 105 dose and placebo has significantly less maximum LDF worsening as compared to the 1 x 106 dose. The comparisons in (b) and (c) were done using general linear modeling (GLM) to compare mean differences among groups. LDFS = Laser Doppler Flow Signal. IC=intracarotid. (Yavagal et al., 2014)
Advantages and Disadvantages of Intra-arterial Route
Preclinical studies point out the superiority of IA and IV routes of administration over direct implantation with ICR and intracerebroventricular (ICV) modes of delivery. Firstly, IA and IV routes are less invasive than ICR and ICV routes. IA may be considered more invasive than IV, however compared to all other routes (ICR, ICV, IV, intrathecal), the IA route should be able to deliver significantly increased number of cells with more uniform cell distribution in the target ischemic brain tissue [11, 52]. The verdict is unclear with many preclinical studies showing diverse results.
Many groups have also shown that IA and IV are equally effective for improving neurological recovery, decreasing cerebral damage, and promoting protection mechanisms in ischemic stroke [57–59]. Vasconcelos-dos-Santos et al. used bone marrow mononuclear cells (BM-MNCs) via IA and IV routes, and Zhang et al. used human umbilical tissue-derived cells via IA, IV, ICR, ICV, and intrathecal routes. Both groups showed improved outcomes with all routes of delivery of stem cells. This was echoed by Savitz et al. who used autologous BM-MNCs and showed no difference in outcomes between IA and IV routes [58]. These studies suggest that IA delivery of BM-MNCs may not be superior to their IV delivery in ischemic stroke. Also, Gutierrez-Fernandez et al. used allogenic MSCs in a permanent middle cerebral artery occlusion (MCAo) rat model and showed no difference in ICR and IV routes of administration on the functional outcomes [17]. The permanent occlusion of the internal carotid artery (ICA)-MCA on the side of IA administration would block direct delivery of MSCs to the infarct area and may be the crucial reason for the absence of superior of IA over IV route in efficacy in this study. On the other hand, several preclinical direct comparison studies between IV and IA routes have shown that IA delivery provides higher concentration of cells to the target tissue than IV delivery [15, 21, 53, 60, 61]. BM-MNCs when delivered via IA route showed better results than IV route on the size of infarct volume, target cell delivery, and motor function after ischemic stroke [21, 61]. Other showed this in ischemic stroke using human MSCs in rodent model [60] and mouse neural stem cells in a mouse model [53]. Guzman et al. have shown using bioluminescence imaging that IA injection results in significantly higher and more sustained cell delivery to the brain [53]. Du et al. [21] in a direct comparison study showed greater functional recovery and increased angiogenesis with IA route compared to IV route using human BM-MSCs in a rat ischemic model. Lundberg et al. proved this concept in the traumatic brain injury (TBI) model. They showed in a rat TBI model, using various cell populations, human MSCs, human neural progenitor cells, and rat neural progenitor cells, that targeted IA route is more effective than IV administration [61]. The superiority of IA route over IV route may partly be due to circumvention of cell sequestration in the peripheral filtering organs such as lung capillaries encountered with the IV route [62]. We feel that there are multiple critical factors that can influence superiority of IA route over IV route including but not limited to homing of stem cells in response to chemoattractants, cell migration, level of arteriosclerosis and resulting stenosis, surviving arterial arborization, and disruption of BBB after stroke.
IA delivery of cells, however, does pose certain safety hurdles that need to be addressed fully prior to clinical translation. Microvascular occlusions leading to decreased cerebral blood perfusion have been reported in preclinical studies with IA stem cell delivery. Chua et al. reported that micro-occlusions can be prevented by preserving anterograde flow [63]. Whether related to microvascular occlusions or not, IA delivery of neural progenitor cells and MSCs has been reported to worsen ischemia and increased mortality in some preclinical studies [64–66]. We investigated this issue in our preclinical studies using multiple concentrations of MSCs delivered via IA route [22]. Dose de-escalation studies with real-time monitoring of MCA flow showed that de-escalation of IA MSC cells doses to 1 × 105 MSCs mitigated the decrease in MCA Doppler flow signal that was seen at higher doses (Figs. 6.2 and 6.3). This IA maximum tolerated dose (MTD) of 1 × 105 MSCs given at 24 h post rMCAO also resulted in significantly superior attenuation of neurological deficits and reduction in infarct size after ischemic stroke as compared to IA vehicle and IV MSC groups (Figs. 6.1 and 6.4). Moreover, delivering MSCs using IA route, we also showed that the therapeutic window extended to 24 h. In fact, MSCs delivered at 24 h after ischemic stroke showed greater beneficial effects as compared to MSCs administered at 1 h. The findings were very promising because the benefits of arterial delivery of MSCs translated into long-term neurological improvement as well as reduction in infarct size (Figs. 6.1 and 6.4). Considering all published preclinical studies till date, the IA route of stem cells delivery remains promising with a number of clinically attractive advantages over other modes of delivery.
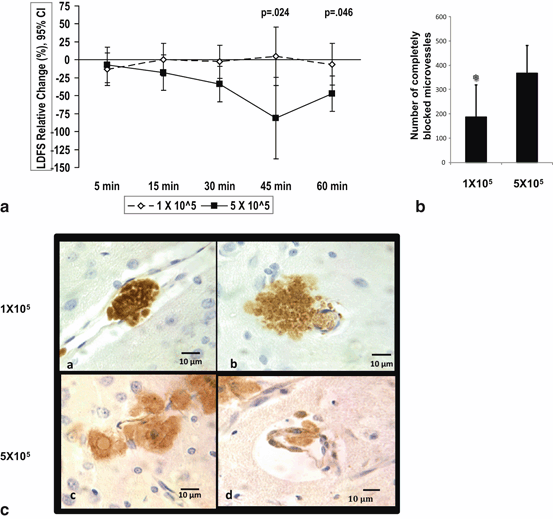
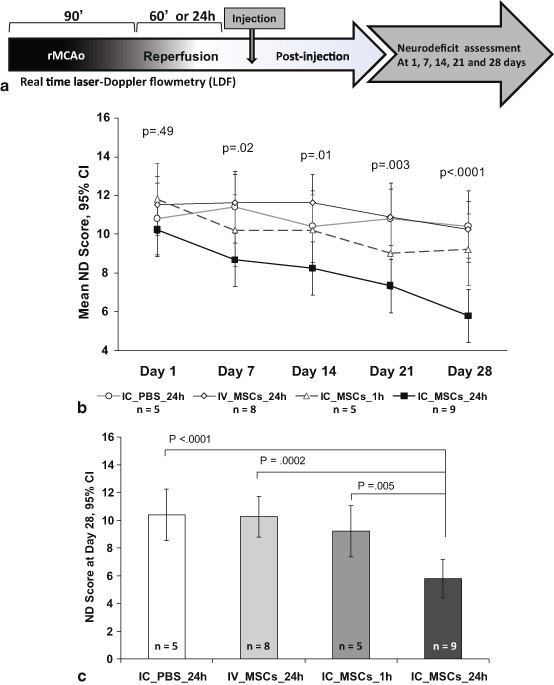
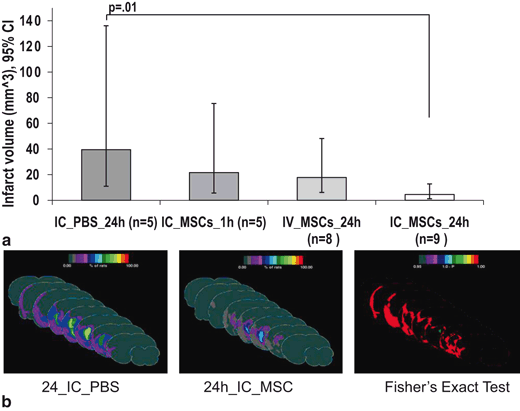
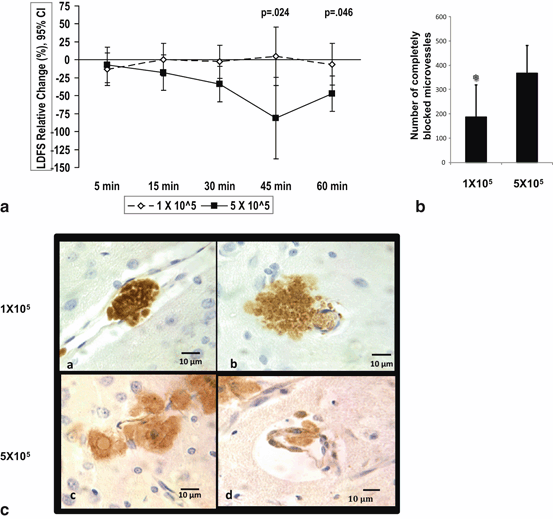
Fig. 6.2
Comparison of 1 x 105 to 5 x 105 dose-groups for LDFS change over time and microvascular occlusion. (a) IC MSC dose of 1 x 105 has a transient and less MCA LDF worsening during 60 minutes post injection as compared to 5 x 105 dose using mixed model. LDFS = Laser Doppler Flow Signal. (b) On comparing the total number of microvessels with complete occlusion among the two dose groups, there were a significantly lower number of complete occlusions in the lower dose group. mean ± SD, * P< 0.05, ANOVA. (c) Representative brain sections from IC MSC 1 x 105 dose group showing GFP + MSCs identified by 3, 3”-diaminobenzidine (DAB), showing localized complete filling of microvessels at 3-5 days post-injection, as well as MSCs just outside the vessel wall in brain parenchyma and high power field of representative brain sections from IC 5 x 105 dose group showing single MSC partly inside and partly outside microvessel wall IC=intracarotid. (Yavagal et al., 2014)
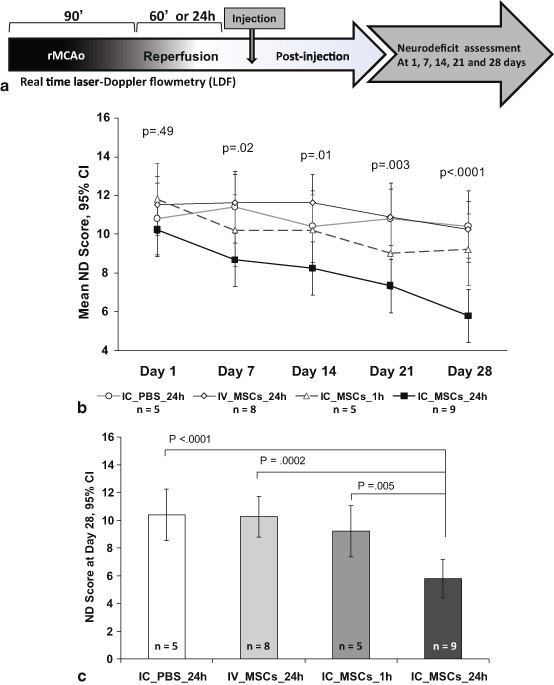
Fig. 6.3
Functional neurologic outcomes are superior in the group treated with 1 x 105 IC MSCs at 24 hours (24h IC MSC). (a) Experimental timeline of the efficacy study showing 90 min rMCAo followed by 24 hour reperfusion except in group receiving IC MSCs at 1 hour reperfusion followed by ND score assessment at 1,7,14,21 & 28 days. (b) On day 1 post rMCAo, the ND scores were not significantly different among groups. The ND score of the 1 x 105 group progressively decreased over time and at 28 days was significantly lower than the other groups. (c) The day 28 ND score was significantly lower in the 24h IC MSC group as compared to all the remaining groups. ND = Neurodeficit. IC=intracarotid. (Yavagal et al., 2014)
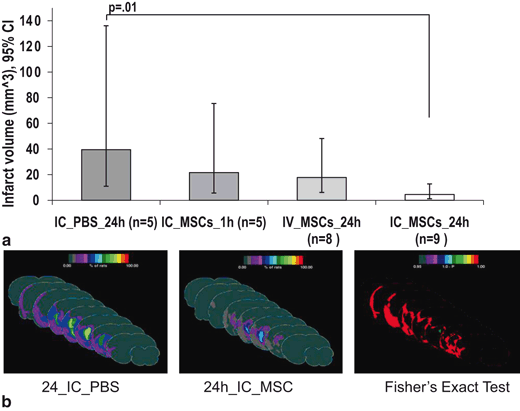
Fig. 6.4
Comparison of infarct volume among treatment groups. (a) Geometric mean infarct volumes are compared among groups after log transformation to achieve normal distribution. Only the 24h IC MSC group shows significantly reduced infarct volume as compared to the 24h IC PBS. (b) Frequency infarct map statistically comparing the location of the mean infarct volume in the 24h IC MSC and 24h IC PBS groups using color- coded representation of the percent of rats showing infarction in each brain region using “Fisher’s exact test” with a color coded representation of the “p-value”, the color bar in “1-p” format. Displays are in “Coronal presentation”; middle sections are selected. The 24h IC MSC group shows a much reduced infarction frequency, particularly surrounding the core as quantized by the Fisher test. IC=intracarotid. (Yavagal et al., 2014)
Clinical Studies and Trials of Intra-arterial Delivery of Stem Cell Therapies in Ischemic Stroke
There has been a surge of early clinical trials of stem cells in acute ischemic stroke and interest has spiked across the globe in the last 5 years [34, 67]. It is of paramount importance to first establish clinical safety during the development of novel therapies. Rapid strides have been made over the past decade in establishing the clinical safety of stem cells in ischemic stroke patients. Kondziolka et al. first demonstrated the feasibility and safety of stem cells in ischemic stroke [68]. They directly implanted stem cells derived from a human teratocarcinoma cell line (NT2N, Layton BioScience Inc, Sunnyvale, CA) via stereotactic ICR delivery in ischemic stroke patients. In this phase I, non-randomized, observer-blinded study involving 12 patients with basal ganglia strokes and motor deficits, they showed that ICR transplantation of stem cells (2–6 × 106) was feasible. All patients were immunosuppressed with cyclosporine A. Two isolated events of a generalized seizure in one patient and a distant brain stem stroke in another patient were thought to be unrelated to the stem cell injections. The 6- and 12-month follow-up positron emission tomography (PET) scans showed improved fluorodeoxyglucose uptake at the implant site in seven and three patients, respectively [68, 69]. Postmortem analysis on one patient who died of myocardial infarction 27 months after transplantation showed the presence of neurons derived from the transplanted cells at the implantation site [70]. Kondziolka et al. followed this study with a phase II, randomized study in nine patients with ischemic and hemorrhagic stroke each [71]. They treated seven patients in each group and four patients served as controls. They reported improved “Action Research Arm Test” gross hand-movement scores compared with the control and baseline values.
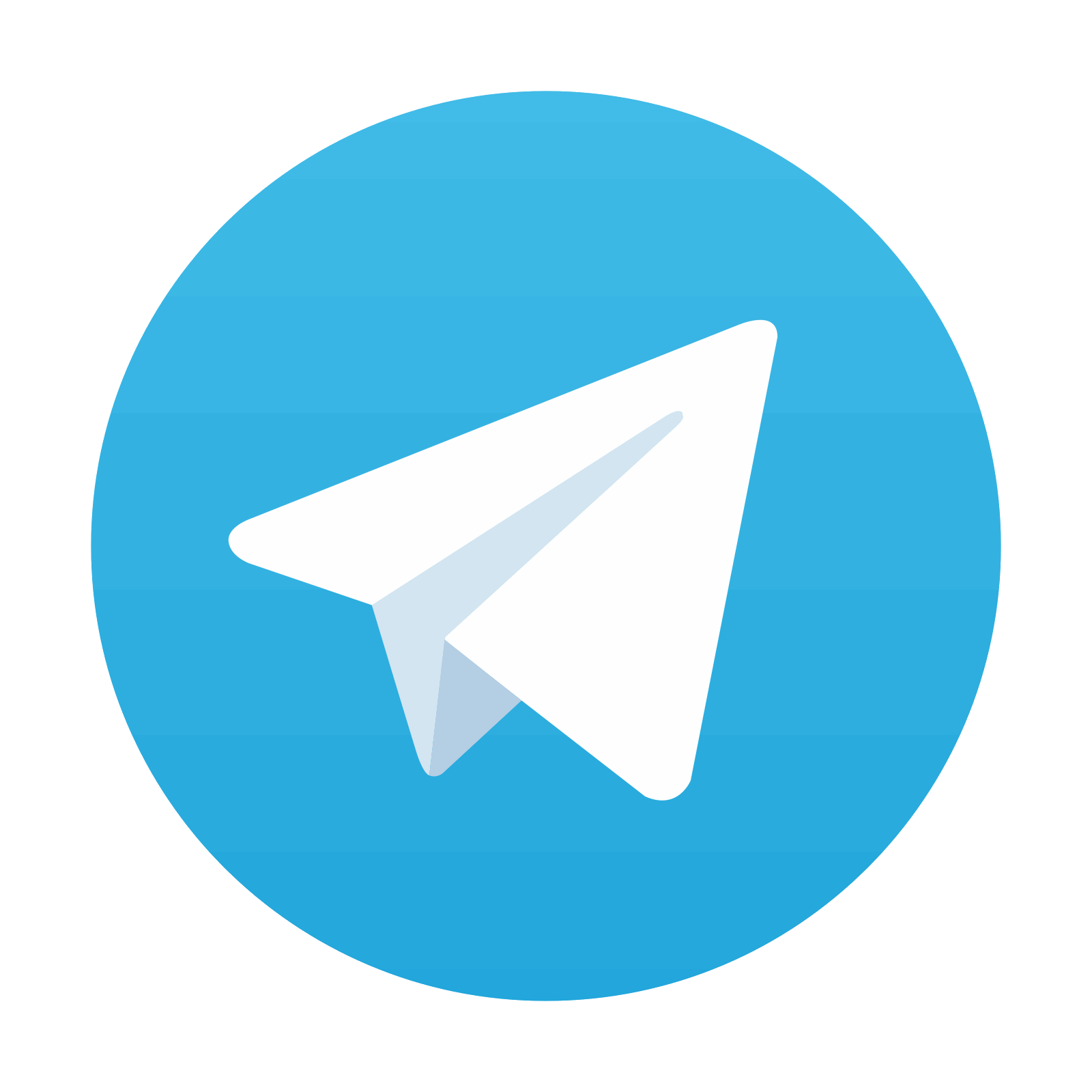
Stay updated, free articles. Join our Telegram channel

Full access? Get Clinical Tree
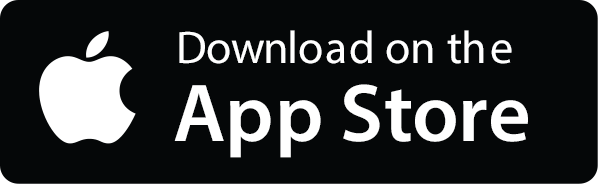
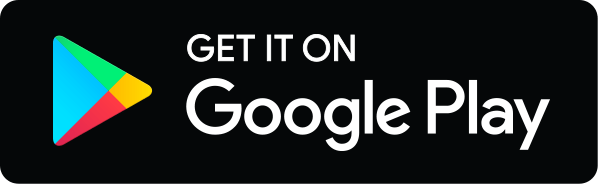