Figure 7.1. Intracranial pressure monitoring placement.
Modalities include: 1) fluid-coupled monitoring; 2) fiberoptic monitoring which utilizes a reflected laser beam; and 3) piezoelectric crystal transducers which, when compressed, produce a voltage proportional to the degree of compression.
Fibre optic and piezoelectric monitors do not require other external transducers and are calibrated or “zeroed” prior to intracranial placement. These monitors are placed into the brain parenchyma and have the advantage that changes in the head of bed do not require re-levelling of a transducer. However, there may be some drift in the system over time, which causes inaccurate readings, and these monitors cannot be recalibrated. If inaccuracy due to drift is suspected, the intraparenchymal component of the monitor must be replaced with the attendant costs and potential complications of reinsertion. These monitors also have no ability to drain CSF.
The zero point for the fibre optic or electronic monitor is at the tip of the catheter. If the monitor is placed deeply compared to a more superficial placement, the measured ICP may be higher by several mmHg due to the hydrostatic weight of the brain above the monitor tip.
A fluid-coupled ventricular monitor is positioned with its tip in the frontal horn of a lateral ventricle. It is connected to an external strain gauge transducer and can be recalibrated and used to drain CSF, which will help with the treatment of intracranial hypertension. Both reliable and accurate, this technique is traditionally considered the gold standard for ICP monitoring. The transducer is calibrated to the level of the foramen of Monro. The tragus, which lies just anterior to the external auditory meatus, serves as the external anatomical landmark for the foramen of Monro. One disadvantage of a fluid-coupled monitor is potential obstruction by brain tissue, blood or bone especially during placement. Other drawbacks are that air within the system will dampen the waveform and artificially lower the pressure, and changes in head position require re-zeroing the transducer. When the ventricles are small and significantly compressed, placement of an intraventricular catheter may be difficult. Fluid-coupling also increases the risk of infection. Some studies have shown that the risk of infection increases with longer duration of catheter placement; however, other studies have reported that infectious risk is highest within the first 10 days and decreases thereafter. This would suggest that the infection is introduced at the time of catheter insertion. Periodic flushing with antimicrobials or routine replacement do not appear to reduce the risk of infection. The use of prophylactic antibiotics is often limited to periprocedural administration just prior to placement, since antimicrobial treatment for the duration the catheter is in place likely increases the risk of resistant organisms and may not reduce the risk of catheter-related ventriculitis. Removing the catheter as soon as possible and minimizing the frequency of opening and accessing the system may reduce the risk of infection. Subcutaneous tunnelling of the catheter away from the insertion site may also lower the risk of infection. Newer antibiotic-impregnated catheters are available.
A subarachnoid screw or bolt is a fluid-coupled metal tube secured in the skull through a burr hole and is connected to an external pressure transducer. These do not effectively drain the CSF and are less accurate than a ventricular monitor, since at higher pressures the screw becomes obstructed with brain tissue.
Subdural monitors can be placed during surgery but are not typically used as they can become obstructed and are considered less accurate.
Epidural catheters are also less accurate due to compartmentalization by the dura from the intraparenchymal space. Because there is a theoretically lower risk of hemorrhage during placement compared to an intraparenchymal monitor, epidural catheters were historically used in patients with coagulopathy who required urgent monitoring (e.g., hepatic encephalopathy and cerebral edema). But because of their inaccuracy, they are not commonly used today.
The semi-rigid dura mater creates compartments within the cranium. The falx cerebri, which is a sagittal fold of the dura mater, splits the supratentorium into two halves. The tentorium cerebelli is a fold of the dura mater that separates the occipital lobes from the cerebellum. The free edges of the tentorium cerebri bilaterally leave an opening that is traversed by the midbrain. Understanding the semi-rigid nature of the dura creating these compartments is relevant during ICP monitoring, since pressure differentials occur in different compartments. A parenchymal monitor in a frontal lobe may not detect elevation of pressure on the contralateral side or infratentorially in the cerebellum. Similarly, a fluid-coupled catheter placed into a lateral ventricle and passed too deeply past the third ventricle may not detect intracranial hypertension occurring in the supratentorium.
Lumbar drains cannot reliably measure intracranial pressure in herniation, when the intracranial and intraspinal spaces become further compartmentalized. In addition, placement of a lumbar catheter can precipitate cerebral herniation when ICP is high and removing CSF from below can create a pressure gradient with a downward shift of the brain.
7.3 The ICP Waveform
Cardiac and respiratory activity creates the pulsatile components of the ICP waveform. The normal ICP waveform has three major components: the percussion wave (P1); the tidal wave (P2); and the dicrotic wave (P3) (Figure 7.2). The percussion wave is the most constant in amplitude and results from systolic pulsation in the large intracranial arteries. The second or tidal wave (P2) is a result of the elastance of the brain. P2 and the dicrotic wave (P3) are separated by the dicrotic notch, which corresponds to the dicrotic notch of the arterial pressure waveform due to closure of the aortic valve during diastole. The dicrotic wave is considered venous in origin. Respiratory contribution to ICP has a tidal variation that is dependent on changes in cerebral venous outflow due to intrapleural pressures that result from changes in intrathoracic and intraabdominal pressure during the ventilatory cycle.
Figure 7.2. Normal ICP waveform (in mmHg). P1 corresponds to the percussion wave, P2 the tidal wave, and P3 the dicrotic wave.
The morphology and pulse pressure of the ICP waveform can be indicative of pathological states. As noted above, elastance describes the change in pressure per unit change in volume. Elastance is dependent on the capacity to displace CSF or intravascular blood when one component of the intracranial compartment increases. Since the ICP waveform results from a bolus of blood from the heart into the intracranial vault, the waveform reflects the elastance. When the elastance increases, the pulse amplitude of the ICP waveform also increases (Figure 7.3).
Figure 7.3. Volume to pressure relationship curve. At low elastance, a change in volume, as depicted by ΔV, increases the intracranial pressure (ICP) by ΔP1; however, at higher elastance when there is less ability for the brain to displace other intracranial components, the same ΔV results in a much greater change in pressure: ΔP2>>ΔP1. Of note is that the pulse pressure of the waveform is much greater and the waveform morphology changes such that the percussion and tidal wave become confluent.
Attempts have been made to predict impending intracranial hypertension when the pressure level is still normal. Increasing elastance is indicative of impending intracranial hypertension. Techniques to detect this include: 1) measurement of the pressure response to the infusion or withdrawal of fluid; 2) ICP pulse amplitude analysis; and 3) ICP waveform analysis.
There are various methods for the instillation or withdrawal of fluid or CSF to evaluate the pressure response. Marmarou proposed the “pressure-volume index” (PVI), wherein a standard amount of fluid is instilled to determine the volume of fluid needed to raise the opening pressure 10 times. In this calculation, the mathematical log of the ICP is linearly related to the volume with a slope equal to the PVI. A decrease in the PVI indicates increasing elastance and correlates with the development of intracranial hypertension and poorer outcome. Miller induced a “volume-pressure response” by rapidly infusing a set amount of fluid into the CSF space, from which he observed that a greater than 3 mmHg rise in pressure for each millilitre infused would predict increasing elastance and worsening ICP. The obvious complications associated with this technique are infection and potential precipitation of sustained ICP elevation, also known as plateau waves, described below.
Another method to detect impending intracranial hypertension that does not require infusion of fluid into the CSF is analysis of the pulse amplitude and variance in ICP. Increasing pulse amplitude and increased ICP variance can be caused by worsening elastance or cerebral vasodilation. It has been proposed that a cardiac to respiratory wave amplitude ratio >2 indicates cerebral vasodilation, while a lower ratio is indicative of increasing elastance.
Finally, when ICP is elevated, the waveform morphology changes further. P2, the tidal wave, becomes more prominent, increases in amplitude, and merges with P1, which results in a widening and rounding of the waveform (Figure 7.4).
But even with the use of these various techniques, the clinical relevance of determining increasing elastance is unclear, since it is uncertain which therapies should be initiated, and how aggressive they should be, to prevent worsening of ICP.
Figure 7.4. Elevated intracranial pressure wave (in mmHg). Note the change in morphology from the normal pressure wave seen in Figure 2. The tidal wave, P2, increases in amplitude and becomes confluent with P1, the percussion wave. The pulse pressure, or the difference between the lowest and highest amplitudes of the waveform, is also larger.
7.4 Elevated Intracranial Pressure and Pressure Waves
Lundberg observed the ICP waveforms of 143 patients with intracranial tumours, spontaneous hemorrhages or head trauma and described three patterns of ICP waveforms as a function of pressure and time (Figure 7.5).
Figure 7.5. Lundberg waveforms.
The Lundberg A wave, also called a plateau wave, persists for 5 to 20 minutes at amplitudes of 50 to 100 mmHg. Lundberg B waves are moderately elevated to about 50 mmHg and persist only over 30 seconds to a few minutes. Lundberg C waves occur approximately 6 to 10 times a minute and have amplitudes of 20 mmHg.
Lundberg hypothesized that the A wave is a result of cerebral autoregulation and an increase in CBV due to vasodilation. Elevation in ICP results in decreased cerebral perfusion pressure. Cerebral vessels dilate to maintain cerebral blood flow constant. This results in prolonged elevation of ICP and the plateau wave. This prolonged elevation may be aborted by draining the CSF or other rapid maneuvers to lower ICP. When elastance is high, hypercarbia or other maneuvers that cause cerebral vasodilation can produce plateau waves. The plateau wave may eventually resolve spontaneously, as the prolonged elevation of ICP results in cerebral ischemia which, in turn, produces an adrenergic discharge, an increase in mean arterial pressure (MAP), and restoration of CPP. When the CPP is adequate, the cerebral vessels constrict and CBV and ICP both decrease. Since ischemia is often associated with Lundberg A waves, they may be associated with permanent cerebral injury. Cerebral ischemia may result in more edema and increased elastance. Plateau waves may be a sign of impending herniation.
Lundberg B waves have no significant clinical effects, but they are considered a sign of cerebral dysfunction. They are seen in comatose patients with Cheyne-Stokes respirations. Variation in CBF occurs in synchrony with B waves. In animal studies, B waves are associated with oscillations in the diameter of the pial vessels and CBV but not with blood pressure or paCO2.
Lundberg C waves correlate with Traube-Hering waves, which are rhythmic oscillations in blood pressure that occur every 6 to 10 times per minute as a result of fluctuations in vasomotor tone and they are independent of the respiratory cycle. They are seen in normal individuals and are not pathological.
7.5 Cerebral Perfusion Pressure
Cerebral perfusion pressure (CPP) can be calculated with placement of an ICP monitor. CPP is the difference between MAP and ICP. When measuring systemic MAP by an invasive technique, the fluid-coupled transducer is zeroed at the phlebostatic axis in the midaxillary line; however, when measuring MAP to determine the CPP, the invasive arterial line transducer, similarly to the fluid-coupled ICP monitor, is calibrated to the tragus, which corresponds to the foramen of Monro.
Normal CPP is between 70 and 100 mmHg. A CPP of 50-60 may be adequate, but ischemia ensues at a CPP <40 mmHg.
When the central venous pressure (CVP) is elevated, venous outflow from the brain is impeded. The resultant increase in CBV elevates ICP. When the CVP exceeds the ICP, the CPP is instead determined by calculating the difference between the MAP and the CVP.
Positive end-expiratory pressure (PEEP) during mechanical ventilation can affect ICP but it is less frequently seen that one might expect. For PEEP to be clinically relevant to ICP, it must increase the intrapleural pressure. This occurs in patients with compliant lungs but poor chest wall compliance. Studies suggest that ICP is affected by PEEP in situations where PEEP exceeds 20 cmH20 and when the chest wall is stiff. In this situation, the right atrial pressure and the superior vena cava pressure both increase and venous outflow from the brain is reduced. In conditions where the mean airway pressure is elevated with increased intrapleural pressure, ICP may be affected in the same manner.
Intraabdominal hypertension and the abdominal compartment syndrome seen in multitrauma or patients with severe ileus can also increase ICP by increasing intrapleural pressure. This pressure is transmitted across the walls of the thoracic vasculature. The CVP rises and again impedes cerebral venous outflow, impeding venous return from the brain and increasing ICP. Elevated intraabdominal pressure also reduces venous return to the heart and can decrease systemic blood pressure, thereby also causing a decrease in CPP.
7.6 Cerebral Autoregulation
In steady-state conditions, cerebral blood vessels dilate or constrict to maintain cerebral blood flow constant. When cerebral autoregulation is intact, vasoconstriction ensues with rising MAP to maintain CBF at approximately 50 mg/100g/min. When the blood pressure decreases, vasodilation will maintain CBF at the same level. In normotensive individuals, autoregulation typically occurs between a MAP of 50-150 mmHg (Figure 7.6).
Figure 7.6. Normal autoregulation curve.
The relevance of this for ICP occurs at times of low compliance or high elastance, when increases in MAP result in vasoconstriction which decreases both CBF and CBV, thereby lowering ICP. Conversely, low blood pressures cause autoregulatory cerebral vasodilation and increased ICP (Figure 7.7).
Figure 7.7. Disrupted autoregulation. Autoregulatory curve in areas of brain injury, such as the core of an ischemic stroke or an area of cerebral contusion in traumatic brain injury, where the cerebral vasculature is disrupted.
In the pathological state, autoregulation is disrupted and CBF cannot be maintained constant. In this situation, decreases in blood pressure directly result in decreased CBF and ischemia. Increases in MAP will increase both CBF and CBV and can result in elevation of ICP. Increases in MAP will also raise the risk of cerebral edema and hemorrhage.
7.7 Indications for ICP Monitoring
Since secondary brain injury such as ischemia can occur in patients with intracranial hypertension, traumatic brain injury (TBI) guidelines recommend the placement of a monitor when the Glasgow Coma Scale score is ≤8 in a patient with an abnormal computed tomography (CT) scan or even when the scan is normal but the patient is aged over 40 years, has unilateral or bilateral motor posturing or systolic blood pressure <90 mmHg. Normal ICP is <10 mmHg. In the Traumatic Coma Data Bank, 72% of patients with severe TBI had an ICP >20 mmHg. Since numerous studies have shown worsened outcome with ICP above 20-25 mmHg, the published guidelines use this as the threshold to treat.
There are no randomized clinical trials to create a strong level of evidence supporting the use of ICP monitoring in other disease states such as ischemic and hemorrhagic stroke (ICH). However, ICP monitoring is considered an option in the 2007 American Heart Association/American Stroke Association guidelines for the management of spontaneous ICH in adults. There are some data to support the use of monitors in hepatic encephalopathy stage IV patients. A contraindication to placement of an ICP monitor is a bleeding diathesis or coagulopathy.
7.8 Conclusions
Intracranial pressure monitoring may be helpful in managing patients with suspected intracranial hypertension. Treating elevated ICP and maintaining adequate CPP may prevent secondary brain injury due to ischemia. An ICP monitor can detect elevations in cerebral pressure before a clinically significant herniation syndrome occurs. Familiarity with the types of monitors available and recognition of waveforms and their patterns can help the clinician to troubleshoot the technical problems that occur during monitoring and to detect worsening of edema.
References
1. Brain Trauma Foundation, American Association of Neurological Surgeons, Congress of Neurological Surgeons. Guidelines for the management of severe traumatic brain injury. Indications for intracranial pressure monitoring. J Neurotrauma 2007; 24(Suppl 1): S37-S44
2. Broderick J, Connolly S, Feldmann E, et al. Guidelines for the management of spontaneous intracerebral hemorrhage in adults: 2007 update. Stroke 2007; 38: 2001-23
3. De Iaet I, CiterioG, Malbrain ML. The influence of intraabdominal hypertension on the central nervous system: current insights and clinical recommendations, is it all in the head? Acta Clin Belg Suppl 2007; 89-97
4. DeWitt DS, Prough DS. Traumatic cerebral vascular injury: the effects of concussive brain injury on the cerebral vasculature. J Neurotraum 2003; 20: 795-825
5. Friedman WA, Vries JK. Percutaneous tunnel ventriculostomy: Summary of 100 procedures. J Neurosurg 1980; 53: 662-5
6. Holloway KL, Barnes T, Choi S, et al. Ventriculostomy infections: the effect of monitoring duration and catheter exchange in 584 patients. J Neurosurg 1996; 85: 419-24
7. Langfitt Tw, Weinstein JD, Kassell NF, et al. Transmission of increased intracranial pressure. I. Within the craniospinal axis. J Neurosurg 1964; 21: 989-97
8. Lundberg N. Continuous recording and control of ventricular fluid pressure in neurosurgical practice. Acta Psychiatr Neurol Scan 1960; 36 (Suppl 49): 1-193
9. Marmarou A, Anderson RL, Ward JD, et al. NINDS Traumatic Coma Data Bank: intracranial pressure monitoring methodology. J Neurosurg 1991; 75: S21-S27
10. Marmarou A, Maset AL, Ward JD, et al. Contribution of CSF and vascular factors to elevation of ICP in severely head-injured patients. J Neurosurg 1987; 66: 883-90
11. Mayhall CG, Archer NH, Lamb VA, et al. Ventriculostomy-related infections: a prospective epidemiologic study. N Engl J Med 1984; 310: 553-9
12. Miller JD, Garibi J, Pickard JD. Induced changes of cerebrospinal fluid volume. Arch Neurol 1973; 28: 265-9
13. Narayan RK, Kishore PRS, Becker DP, et al. Intracranial pressure: to monitor or not to monitor? A review of our experience with severe head injury. J Neurosurg 1982; 56: 650-9
14. Shapiro S, Bowman R, Callahan J, et al. The fiberoptic intraparenchymal cerebral pressure monitor in 244 patients. Surg Neurol 1996; 45: 278-82
15. Yablon JS, Lantner HJ, McCormack TM, et al. Clinical experience with a fiberoptic intracranial pressure monitor. J Clin Monit 1993; 9: 171-5
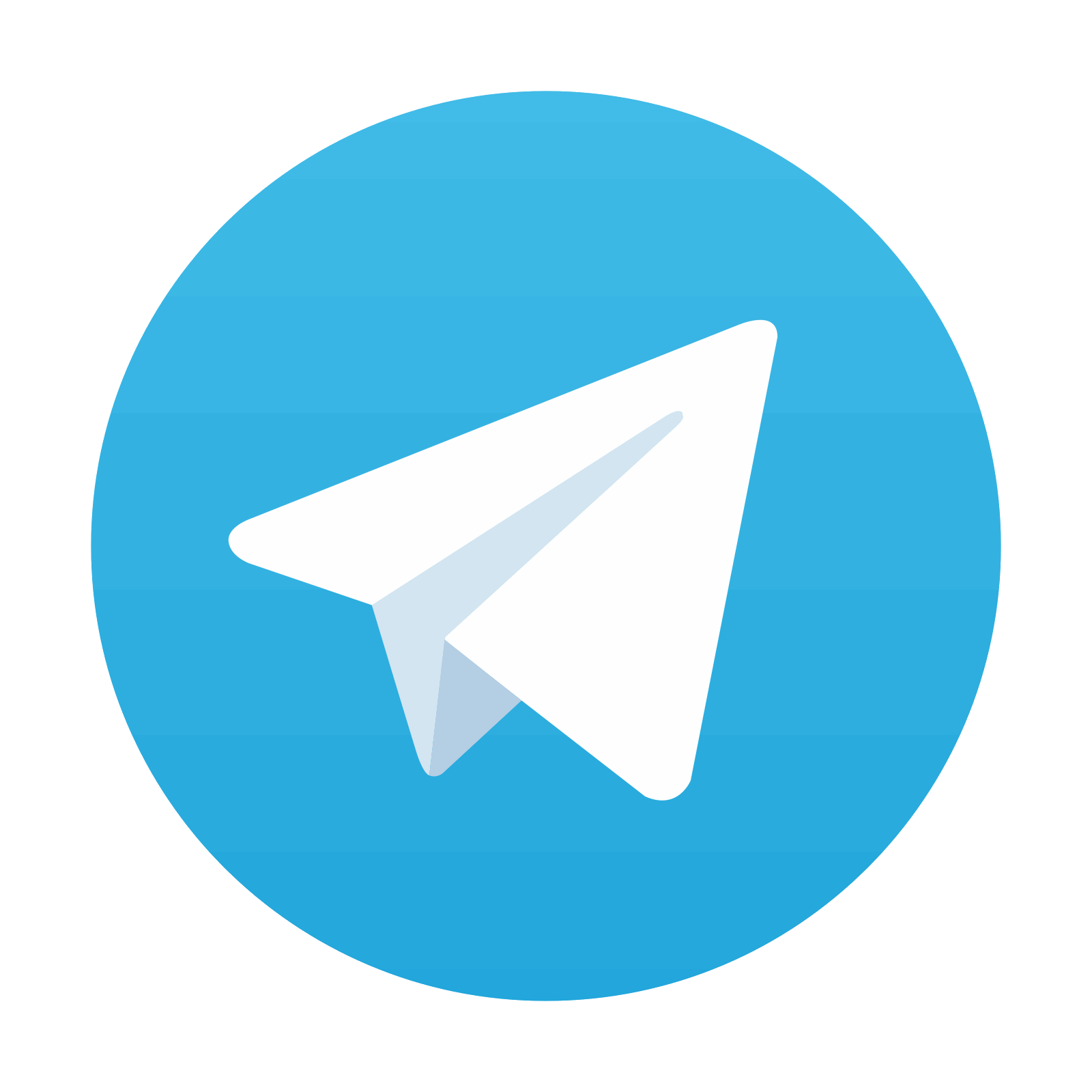
Stay updated, free articles. Join our Telegram channel

Full access? Get Clinical Tree
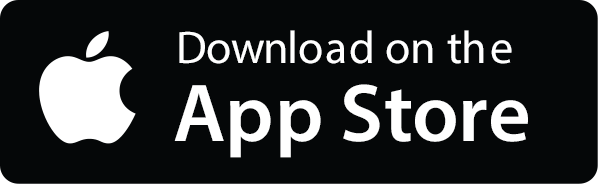
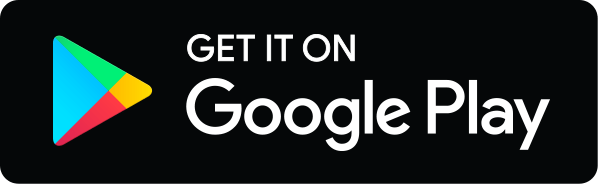