CHAPTER 269 Intraoperative Monitoring of the Spinal Cord and Nerve Roots
Intraoperative neurophysiologic monitoring is an important technique that is essential for improvement of safety in modern complex spine surgery.1–3 In its ideal form, a properly engineered intraoperative monitoring environment provides real-time feedback information to the surgical team about the functional status of the spinal cord and nerve roots under surgical manipulation so that preventive or corrective actions can be taken to avoid irreversible injuries.4–8 To gain universal acceptance and facilitate utilization, an ideal monitoring environment should include multiple properly designed human-machine interfaces, some of which involve biomedical engineering. It is also imperative that an optimal team of neurophysiologists and neurosurgeons be assembled to properly and efficiently use these technologies. Intraoperative monitoring modalities should have high sensitivity, high specificity, low invasiveness, and ease of use. Although numerous intraoperative monitoring techniques have been investigated since the days of Penfield and Boldrey,9 no single monitoring method has been able to satisfy these requirements in clinical spine surgery practice. However, general consensus regarding the use of intraoperative neurophysiologic monitoring is emerging as the evidence for monitoring continues to build,10 thereby encouraging optimism for the development of an ideal intraoperative monitoring modality.
Somatosensory Evoked Potentials
Monitoring of dorsal column integrity with SSEPs is the most commonly used technique in spine surgery.11 Early monitoring solely with SSEPs resulted in false-negative results in multiple reports and thus necessitated the development of multimodality monitoring.12–14 Nevertheless, SSEPs remain a standard modality with demonstrated improved neurological outcomes in corrective procedures for spinal deformity,4,6,7 and it serves as a building block for more complex monitoring arrangements. Multiple reports of the use of SSEPs in spine surgery, ranging from the early 1980s to current prospective studies involving larger patient populations, have demonstrated an improvement in outcome.15–17
In SSEP monitoring, stimulation electrodes excite controlled repetitive action potentials propagating from peripheral nerves to dorsal roots, the dorsomedial tracts of the spinal cord, and eventually the contralateral sensory cortex, along which multiple recording sites may be anatomically accessible. Typically, platinum subdermal needle electrodes are used for both purposes. Stimulation sites for the upper extremity include the median and ulnar nerves. For the lower extremity, the posterior tibial nerve is a standard location. Bipolar stimulation (requiring both cathode and anode placement) is typically used. Electrode impedances are key parameters to ensure contact and reduce artifacts. Impedance values below 1 to 5 kΩ are clinically used thresholds for interelectrode or individual electrode measurements, respectively. Typical stimulation waveforms are 250-µsec-duration square-wave pulse trains at 4.7 Hz, with stimulation amplitudes ranging from 20 to 40 mA, depending on patient variables. Recording electrode positioning follows set standards, such as the international 10-20 system (Fig. 269-1).18 For example, Cv2-Fpz for the N31 subcortical response at the cervicomedullary junction and Cpz-Fpz for P37/N45 responses at the cerebral sensory cortex are commonly used. Other recording sites such as Erb’s point are also used. It is worth noting that after neural stimulation of the lower extremities (e.g., at the posterior tibial nerve), SSEP monitoring also reflects the physiologic integrity of the spinocerebellar tracts, which involve the dorsal nucleus of Clarke’s column between T1 and L2.
Subcortical signals have lower signal amplitude and a reduced signal-to-noise ratio within a given amplifier gain and averaging setting but are more resistant to changes in depth of anesthesia. Cortical signals have larger amplitude and a higher signal-to-noise ratio and therefore tend to be more reliable electrically but are sensitive to anesthetic-induced changes. This tradeoff is illustrated in the improvement in reliability, approaching 93% to 98% in selected studies,4,19–26 when using multiple cortical and subcortical recording sites. Recent advances in digitization equipment, filtering algorithms, and signal processing have resulted in the continued reduction of system complexity, which may potentially translate into a reduction in cost or an increase in the number of concurrently monitored channels.
Motor Evoked Potentials
Transcranial electrical or magnetic stimulation of the cerebral motor cortex causes muscle activation, which can be detected peripherally to provide additional monitoring coverage specifically designed to detect motor deficits.27,29 This is particularly important with intramedullary resections and in procedures with a risk for vascular compromise of the anterior spinal artery and its associated vascular territory, which can affect motor function. Initial work28,29 paved the way for current single-pulse stimulation techniques, in which corticospinal tract recordings of direct waves (D waves) are detected, and multipulse stimulation techniques, in which short pulse trains are used to elicit CMAPs.30
Typically, in patients under general anesthesia, MEP monitoring involves an electrode montage over the scalp based on the international 10-20 electroencephalography system. The standard setup uses C3-4 for upper limb MEPs and C1-2 for the lower limbs, with selection of corkscrew, needle, or cup electrodes being based on the clinical scenario and the patient’s age. Skull thickness, electrode type and impedance, and the need for depth of stimulation determine the required stimulation amplitude. Typically, selective stimulation is preferred, and therefore superficial coverage of a small cortical area is used to provide activation of a single limb. With few exceptions, D waves directly generated by the electrical activation of axons of cortical motor neurons are recorded in patients under general anesthesia. This is due, in part, to the fact that vertically oriented excitatory chains of neurons terminating on the cortical motor neuron are blocked by anesthetics, thus abolishing indirect activation of the motor neuron and the associated indirect (I) waves.1 For D-wave recording, one or more sterile intraoperative epidural electrodes are required and placed caudally and cranially relative to the surgical lesion. Signal amplification typically requires a gain setting of 10,000× and bandpass filtering between 1.5 and 1700 Hz. Only a few traces are required for averaging, and the stimulation can be repeated at 0.5 to 2 Hz to provide real-time feedback.1 A reduction in D-wave amplitude of greater than 50% has been shown to correlate with new postoperative motor deficits.31 Myogenic MEPs use a short (5 to 7) pulse train with a 4-msec interpulse interval at the transcranial electrodes. Recordings of the resultant CMAPs at sites such as the thenar muscles or tibialis anterior are amplified at 10,000× and bandpass-filtered between 1.5 and 853 Hz. Again, multitrace signal averaging is not frequently required, and real-time feedback is feasible.
Multipulse stimulation and CMAP detection provide the benefit of monitoring the corticospinal tract, in addition to the distal muscle functional units, including the neuromuscular junction. Although the muscle units provide a physiologic nonlinear amplifier for the MEPs, which improves the electrical signal-to-noise ratio, the nonlinear relationship and preoperative muscle atrophy may cloud the interpretation. Thus, myogenic MEPs are typically interpreted as an all-or-none phenomenon with regard to preoperative clinical assessment and electrophysiologic findings. When MEP amplitude is lost, immediate intraoperative intervention can result in complete reversal, which can be sensitive and specific in predicting new postsurgical motor deficits.32 In conditions in which reliable transcranially stimulated MEPs are difficult to obtain, neurogenic MEPs, obtained through percutaneous stimulation, have been investigated.33,34 When combined with SSEP monitoring of the dorsal column, MEPs provide additional coverage for the ventral corticospinal tract and improve the efficacy of intraoperative monitoring (Fig. 269-2). The intermittent nature of MEP monitoring requires the surgeon to actively request interval measurements. These measurements are generally first taken as a baseline before surgery and then at surgical opening, during exposures approaching the spinal cord, on manipulation of the spinal cord, during different phases of intramedullary resection, and at closure. Close interaction between the neurophysiologist and the surgical team also allows periodic pauses intraoperatively to ensure that reliable recordings are being obtained throughout the procedure.
Electromyography
Typically, free-running or spontaneous EMG activities can be recorded with paired stainless steel needle electrodes insulated to within 5 mm of the tip and transdermally inserted into the target muscle. For example, lumbosacral surgeries may use bilateral quadriceps femoris, tibialis anterior, and gastrocnemius recordings to achieve myotomal coverage from L2 to S2. Electrode impedance below 5 kΩ and interelectrode impedance below 1 kΩ ensure electrical contact. EMG recordings are obtained with a 200-µV/division gain, bandpass filtering between 10 and 5000 Hz, and analysis time of 1.5 to 2.0 seconds. When the nerve or nerve root is compressed, irritated, or injured, spontaneous firing occurs and activation of the corresponding motor unit is monitored by EMG, with different manifestations noted that may correlate with increasing degrees of injury. Morphologic descriptions such as spikes, bursts, train activity, and neurotonic discharges correspond to individual discharges, brief bundles of discharges, persistent regularly repeated discharge patterns, and persistent prolonged bursting, respectively. Intraoperative neuromonitoring units typically contain loudspeakers that convert the electrical activity into audio feedback to the surgical team in real time. A single burst of activity synchronized to surgical manipulations is useful for functional identification and correlation of specific roots and typically does not result in adverse outcomes. Sustained activity (>2 seconds) prompts the electrophysiologist to alert the surgeon to potential neurological deficits. The overall level of EMG activity may also correlate with outcome; however, further research is required to provide definitive sensitivity and specificity. It is also possible that during EMG-monitored procedures, false-positive results may be the direct result of intraoperative monitoring, with excessive EMG activity usually resulting in alteration or cessation of surgical manipulation and irritation of the nerve roots in question. Figure 269-3 shows typical intraoperative free-running EMG monitoring.
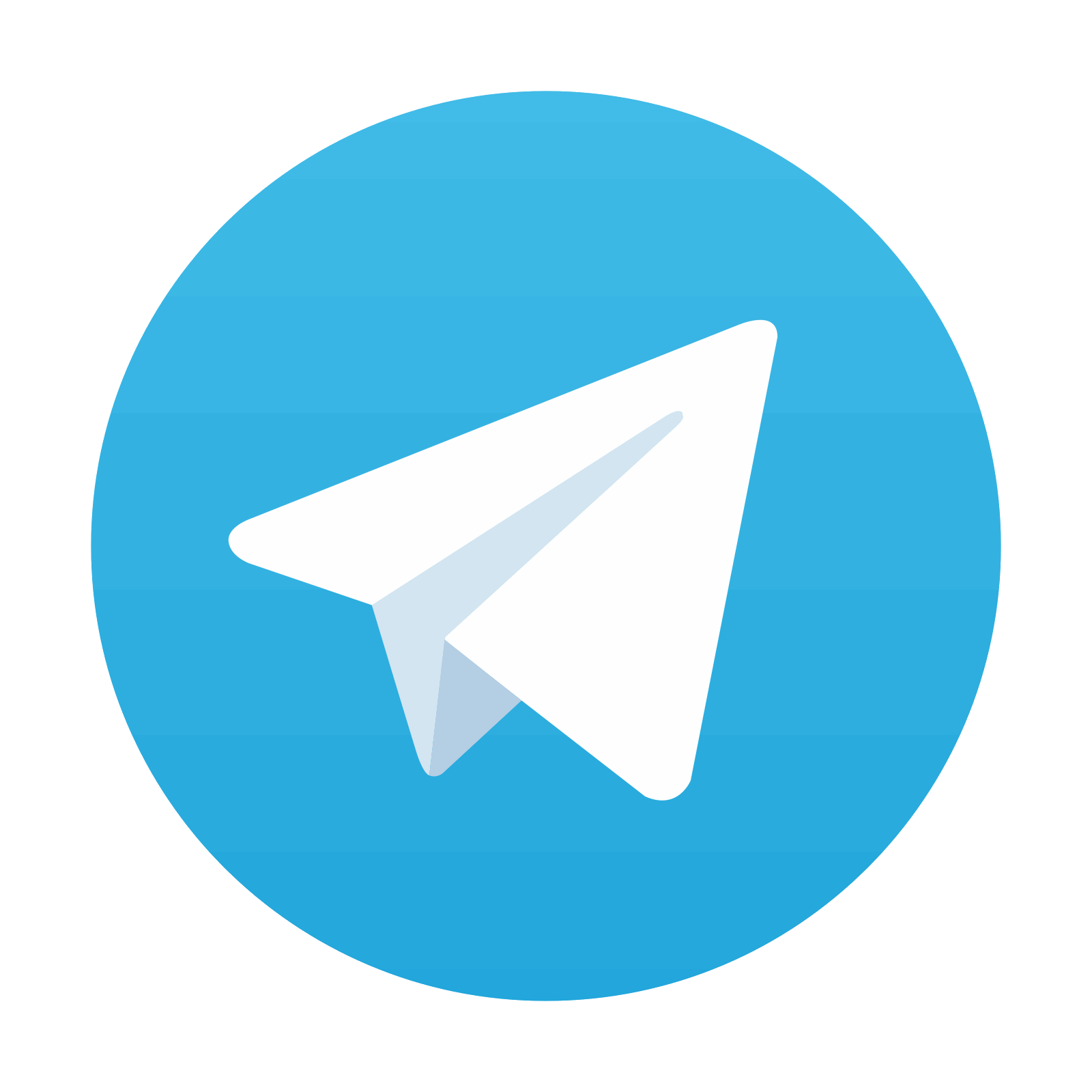
Stay updated, free articles. Join our Telegram channel

Full access? Get Clinical Tree
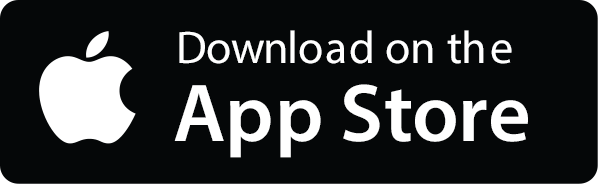
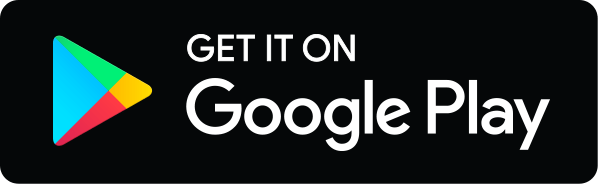