Fig. 13.1
Schematic classification of intraoperative neurophysiology techniques in the posterior fossa surgery. Left panel: neurophysiological mapping allows to identify the functional landmarks such as the nuclei of motor cranial nerves on the floor of the fourth ventricle. (a) A handheld monopolar probe is used to electrically stimulate the rhomboid fossa. (b) Compound muscle action potentials are recorded from the muscles innervated by motor cranial nerves. VII recording from the orbicularis oris for the facial nerve, IX/X recording from the posterior wall of the pharynx for the glossopharyngeal/vagus complex, XII recording from the tongue muscles for the hypoglossal nerve. Right panel: neurophysiological monitoring allows to keep under control the functional integrity of neural pathways (motor, sensory, auditory,…) throughout the surgery. See the text for further details on each monitoring technique. MEPs motor-evoked potentials, SEPs somatosensory-evoked potentials, BAERs brainstem auditory-evoked responses, CBT corticobulbar tract
Mapping techniques: these techniques allow the functional identification of neural structures that are ambiguous from a merely anatomical standpoint. The following mapping techniques are relevant to posterior fossa surgery:
1.
Identification of oculomotor nerves (III–(IV)–VI) through direct stimulation of the peripheral nerve
2.
Identification of cranial motor nerves (VII–IX/X, XI, XII) through direct stimulation of the peripheral nerve
3.
Identification of the oculomotor nerve nuclei at the level of the tectal plate
4.
Identification of the corticospinal tract (CT) at the level of the cerebral peduncle through direct stimulation
5.
Identification of safe entry zones to the brainstem through direct mapping of the floor of the fourth ventricle to localize cranial motor nerve nuclei.
Mapping techniques allow identifying neural structures at a specific point in time but do not provide any information on what happens to these structures between one mapping and the following one. So, for example, we can identify the facial nerve nuclei on the floor of the fourth ventricle while approaching an intra-axial pontine lesion. Once the nuclei have been localized, the ependyma is open and dissection starts to detach the tumor from the surrounding parenchyma. If hazardous coagulation, traction, or any other surgical maneuver at this time compromises the brainstem through either mechanical and/or vascular injury, mapping techniques cannot assist to recognize these events. To do so, monitoring techniques should be used.
Monitoring techniques: these are true evoked potentials and provide continuous feedback on the functional integrity of neural pathways. Unlikely from mapping techniques, monitoring techniques do not localize function but are the best way to provide “online” information on the well-being of different pathways. The following are used in posterior fossa surgery.
1.
Motor-evoked potential monitoring (MEP)
2.
Corticobulbar motor-evoked potential monitoring (CBT MEP)
3.
Free-running electromyography (EMG)
4.
Somatosensory-evoked potentials (SEPs)
5.
Brainstem auditory-evoked responses (BAERs)
13.2.1 Mapping Techniques
13.2.1.1 Identification of Oculomotor Nerves (III–(IV)–VI) Through Direct Stimulation of the Peripheral Nerve
Peripheral cranial motor nerves can be identified through direct stimulation. Either a handheld monopolar probe or a bipolar concentric probe can be used to deliver low-intensity stimuli directly to the nerve. The advantage of bipolar stimulation is a limited spreading of the current (rectangular pulses of 0.2 ms duration at 1–3 Hz and intensity up to 0.5–3 mA). This could be advantageous when the goal is to identify a peripheral nerve encased in or dislocated by a tumor, to reduce the risk of activation of nearby fibers.
Recordings for mapping techniques are obtained by placing needle electrodes in the muscles innervated by their respective cranial nerves. However, these needles may be a bit traumatic in children, especially for hypoglossal, laryngeal, and, even more, oculomotor muscles, given their small sizes. Wire Teflon-coated electrodes may be used instead. The placement of recording electrodes in extrinsic oculomotor muscles may require the assistance of an ophthalmologist to avoid misplacement of the electrodes and injury to the ocular bulb, and one pair of electrodes is inserted in the superior rectus and the lateral rectus muscles to monitor the III and VI cranial nerve, bilaterally, and in the superior oblique for the trochlear nerve. When tissue of ambiguous origin is encountered during surgery, the tip of the stimulator is placed on the tissue, and the oscilloscope displays the recording muscles to determine whether this is a neural tissue or not. Similarly, mapping can also be used to confirm electrophysiologically the visual identification of a nerve. Especially when working in narrow spaces where the concentration of neural structures is high (e.g., the cavernous sinus), it is of outmost importance to adjust the stimulus intensity so that a response is obtained from only one muscle at a time. If the intensity is too high, the current may spread and the localizing value of the mapping decreases. Compound muscle action potentials (CMAPs) from extraocular muscles are usually of low amplitude as their muscle units have a considerably smaller number of fibers innervated by one axon, as compared to peripheral skeletal muscle units. Latency of the response obviously depends on the point of stimulation along the peripheral nerve, but in general it ranges between 2 and 5 ms [64, 65].
Other authors [21] have proposed less invasive recording using electrooculographic monitoring, but this was a rather small series, and the specificity and sensitivity of this method are not well established yet.
Overall, the application of intraoperative mapping of peripheral oculomotor nerves remains anecdotal and likely of little relevance in pediatric neurosurgery. Nevertheless it could be indicated during surgery for lesions involving the cisternal, cavernous, or intraorbital segment of these nerves.
13.2.1.2 Identification of Cranial Motor Nerves (VII–IX/X, XI, XII) Through Direct Stimulation of the Peripheral Nerve
Motor cranial nerves V–XII can be mapped during surgery for skull base and cerebellopontine angle tumors. Stimulation parameters similar to those used for mapping the oculomotor nerves can be used, considering that when stimulating directly the nerve, 0.1–0.3 mA usually suffices to elicit a CMAP; higher intensities could be required to elicit a response from nerve fibers encased in tumoral tissue. For recording, needle or wire electrodes are inserted in the following muscles: the masseter (V), orbicularis oculi and oris (VII), posterior wall of the pharynx (IX/X complex), vocal cords (X), trapezius (XI), and tongue (XII) (Fig. 13.2).


Fig. 13.2
Identification of cranial motor nerves through direct stimulation of the peripheral nerve. (a) Direct stimulation of the right hypoglossal nerve (XII) at its exit zone from the brainstem (BS). (b) Screenshot of online mapping results. A large compound action muscle potential (CMAP) is consistently recorded from the right hypoglossal muscles (RH). A small CMAP is recorded also from the posterior wall of the pharynx muscles (RG), most likely due to some current spreading while using a monopolar stimulating probe. No responses are recorded from the right abductor pollicis brevis muscle (RA). Stimulation intensity was 0.2 mA and stimulus duration 0.5 ms
A typical example is the identification of the facial nerve in the cerebellopontine angle during surgery for vestibular schwannomas in children with NF2. Besides the identification of the nerve or its fascicles during the removal of the tumor, some authors suggest to repeat a proximal stimulation (close to the brainstem) at the end of surgery, before closure, to assess the functional integrity of the nerve. A low stimulating threshold should warrant a good clinical outcome of the facial nerve [33]. Yet, a recent publication by Sugruhe et al. [68] suggests that elevated stimulation threshold exceeding >0.05 mA is a highly specific (90 %), but little sensitive (29 %) finding.
The possibility to locate other cranial nerves and record the so-called CMAP peripherally has become a standard technique in skull base surgery. In the pediatric population, it could be valuable, for example, when dealing with ependymomas invading the cerebellopontine angle through the lateral recess, in order to identify and preserve the lower cranial nerve peripherally.
13.2.1.3 Identification of the Oculomotor Nerve Nuclei at the Level of the Tectal Plate
Besides the identification of peripheral motor cranial nerves in the posterior fossa through direct stimulation, the same technique can be used to identify relatively safe entry zones into the brainstem. Crowded by the cranial nerve nuclei and ascending, descending, and interconnecting fascicles, bundles, pathways, and reticular formation, the brainstem presents a highly complex structure both anatomically and functionally. This makes the brainstem a sort of neurological “minefield” such that surgical resection of brainstem tumors demands meticulous microsurgical technique due to the narrow routes leading to the lesion. If the tumor is exophyting outside the brainstem surface, its removal clearly begins at such outgrowth. In such cases, the tumor itself creates its own entry into the brainstem where it may be penetrated and eventually removed. However, tumors truly intrinsic with no surface component will require greater care and a full understanding of the local functional anatomy. This latter can be unpredictably abnormal due to tumoral mass effect and distortion of anatomical landmarks, to the point that ION may be invaluable to a reliable selection of safe entry zones.
The midbrain, which occupies the notch of the tentorium, consists of a dorsal part (the tectal plate), a large ventral portion (the tegmentum), and the cerebral peduncles. During dorsal approaches to treat intrinsic midbrain lesions, it is crucial to minimize injury to the oculomotor nerve nuclei and intramedullary tracts in order not to compromise the quality of life of these patients. This problem is not uncommon in the pediatric population that typically harbors a number of neoplastic lesions in this region. The great majority of midbrain gliomas are focal, benign astrocytomas. These tumors usually arise from either the tectal plate or tegmentum and may extend upward to the thalamus or downward to the pons, displacing but not infiltrating these structures [23, 39, 72]. Germinomas, teratomas, and primitive neuroectodermal tumors can also be found in this location.
Direct neurophysiological mapping of the tectal plate can therefore be used to identify safe entry zones to approach intrinsic midbrain lesions. Stimulation and recording techniques are the same used for mapping the peripheral oculomotor nerves, except for the stimulation intensity that at the level of the brainstem is usually kept very low starting at 0.05 mA and usually not exceeding 1–1.5 mA. Reports are anecdotal [18, 25, 65], but it appears that direct mapping is of little help to select the entry zone as it is almost impossible to obtain a positive response when stimulating directly the superior collicula (Fig. 13.3c). This is because the superficial layers of the colliculus connect to the visual system by projection to the thalamus and the lateral geniculate nuclei, while the nuclei of the oculomotor nerves are embedded deeper in the periaqueductal gray matter, too far to be activated by superficial stimulation. This is in agreement also with our experience. Once in the brainstem, however, from the cavity wall it is possible to use neurophysiological mapping to localize the oculomotor nuclei (Fig. 13.3d).




Fig. 13.3
Identification of the oculomotor nerve nuclei at the level of the tectal plate. (a) Sagittal (left), coronal (middle), and axial (right) magnetic resonance images of a midbrain cavernoma. (b) One pair of wire electrodes is inserted in the upper rectus and lateral rectus muscles, bilaterally, to record compound action muscle potentials (CMAPs) after stimulation of the III and VI cranial nerve nuclei, respectively. (c) Initially (time 12.33), direct stimulation of the superior colliculus (left panel) does not elicit any response from the oculomotor muscles innervated by the III and VI cranial nerves (right panel). (d) Later on (time 13.41), stimulation from inside the surgical cavity, during removal of the cavernoma, elicits a consistent response (arrow) from the left upper rectus muscles (L III), indicating stimulation of the nearby nuclei. R III right upper rectus muscle, L III left upper rectus muscle, R VI right lateral rectus muscle, L VI left lateral rectus muscle
Overall it could be concluded that the reliability of mapping the oculomotor nuclei and their tracts in the tegmentum is likely not as good as that achieved in direct mapping of other cranial nerves and of the floor of the fourth ventricle.
13.2.1.4 Identification of the Corticospinal Tract at the Level of the Cerebral Peduncle Through Direct Stimulation (Fig. 13.4)


Fig. 13.4
Identification of the corticospinal tract at the level of the cerebral peduncle. (a) Preoperative gadolinium-enhanced T1-weighted images of a pilocytic astrocytoma of the left cerebral peduncle. Coronal (left), sagittal (middle), and axial (right) view. (b) Schematic illustration of direct stimulation of the corticospinal tract at the level of the cerebral peduncle by using a monopolar handheld probe with a short train of stimuli (each stimulus 0.5 ms duration) at 1 Hz and current up to 2 mA (left panel). Intraoperative view of stimulation of the left cerebral peduncle in the patient depicted in (a). The tumor was approached through a left lateral supracerebellar infratentorial route (right panel). (c) Schematic illustration of the motor-evoked potential recorded from the abductor pollicis brevis (APB) muscle following direct stimulation of the cerebral peduncle (left panel). Mapping results in the same patients depicted in (a, b). A consistent response from the left ABP (LA) was recorded, while no responses were recorded in the left tibialis anterior muscle (LT) and in the right side muscles (RA and RT) (right panel). (d) The tumor was then removed entering the lateral midbrain posteriorly to the zone where the left APB response was elicited. Continuous transcranial MEP monitoring was performed during the surgery with no significant changes. The postoperative gadolinium-enhanced T1-weighted images documented a complete removal of the tumor, and the patient presented no additional motor deficits (Modified from Sala and Lanteri [61])
With lesions involving the anterolateral aspect of the midbrain, it is crucial to avoid injuring the CT. The lateral mesencephalic vein [55], which courses into the lateral mesencephalic sulcus, is a useful anatomical landmark because it usually delimits posteriorly the corticospinal tract. Therefore, the entry zone to the brainstem is posterior to this sulcus in order to avoid injury to the pyramidal tract in the peduncle. However, local anatomy can be distorted by the tumor so that only a functional identification of the motor tracts can allow a safe entry to the lateral midbrain.
To identify the CT we use a handheld monopolar stimulator (tip diameter 0.75 mm) as cathode, with a needle electrode inserted in nearby muscles as anode. The response is recorded as a CMAP from one or more muscles of contralateral limbs, after a train of four to five stimuli of 0.5 ms duration, at 1–2 Hz. We usually increase stimulation intensity up to 2 mA, starting from 0.5 mA. Other authors have successfully used higher intensities [70]. When a motor response is recorded, the probe is then moved in small increments of 1 mm in order to find the lowest threshold to elicit that response. In the case of cystic midbrain lesion (i.e., a pilocytic astrocytoma, common in this location), sometimes mapping of the CT is negative at the beginning of the procedure, but a positive response could be recorded when mapping from within the cystic cavity towards the anterolateral cystic wall.
13.2.1.5 Identification of Safe Entry Zones to the Brainstem Through Direct Mapping of the Floor of the Fourth Ventricle to Localize Cranial Motor Nerve Nuclei
For the great majority of surgical approaches to the intrinsic tumors of the pons and the medulla – especially for tumors located in the dorsal part of the pons and the open portion of the medulla – the access is by a suboccipital craniotomy and trans–fourth–ventricle route.
Entering the floor of the fourth ventricle requires a great understanding of the underlying structures.
At the level of the pons, the more prominent part of the median eminence, the facial colliculus, represents a highly dangerous brainstem “entry zone” through the rhomboid fossa [34]. Damage to this area invariably causes facial (VII) and abducens (VI) nerve paralysis as well as lateral gaze disturbances due to parapontine reticular formation dysfunction. Injury to the medial longitudinal fascicles, which border the median sulcus and lie between the abducens and oculomotor nuclei (the so-called VI–III pathway), may result in internuclear ophthalmoplegia.
More caudally, at the level of the medulla, within the small concavity of the calamus scriptorius situated above the obex and usually below the striae medullaris lie two triangles of great functional importance: the hypoglossal triangle and the ala cinerea or vagal triangle. Immediately below the two medial triangles lie the hypoglossal nuclei, which control the muscles of the tongue. Due to the close proximity of the two nuclei, surgical injury to this area almost always results in severe tongue paralysis and atrophy. Since hypoglossal paralysis represents one of the most devastating cranial nerve deficits, even a minor injury in this area must be avoided.
Lateral to the hypoglossal are the vagal triangles and under these lie the dorsal nuclei of the vagus from where motor fibers to the bronchi, heart, and stomach originate. Slightly deeper and lateral lays the nucleus ambiguous, which gives rise to fibers of the glossopharyngeal (IX), vagus (X), and accessory (XI) nerves, supplying musculature to the palate, pharynx, and larynx. Therefore, injury to this small area may result in deficits such as impaired swallowing, dysphonia, nose regurgitation, and coughing reflex loss, thus exposing the patient to the risk of aspiration pneumonia and incapacity to eat or drink [7].
Although anatomical landmarks have been described to safely enter the floor of the fourth ventricle [32, 34, 67], these may not be reliable due to distortion induced by the tumor (see Fig. 13.5). Displacement of a classical anatomical landmark such as the facial colliculus is commonly faced during brainstem surgery. Similarly, even when anatomy is not significantly distorted by the tumor, the identification of areas overlapping the lower cranial nerve motor nuclei can be challenging. Mapping techniques are now available to intraoperatively identify the VII, X–IX, and XII motor nuclei or their intramedullary tracts on the floor of the fourth ventricle.


Fig. 13.5
Identification of the facial colliculus through direct mapping of the floor of the fourth ventricle. Left panel: preoperative contrast-enhanced T1-weighted MR images of a left pontine astrocytoma in a 12-year-old girl, sagittal (top), coronal (middle), and axial (bottom) view. The tumor was approached through a median suboccipital craniectomy. At surgery, the median sulcus on the floor of the ventricle was dislocated to the right, and the left median eminence was expanded. Middle upper panel: mapping of the facial nerve motor nuclei on the floor of the fourth ventricle. (a) On the left side, about 1.5 cm rostral to the striae medullares, a response was obtained from the left orbicularis oculi (LU) at 1.5 mA. (b) A response from the left orbicularis oris (LL) was recorded at a lower stimulation (0.5 mA) when the handheld probe was moved caudally and to the right. (c) When the probe was moved further down and to the right, the stimulation elicited a consistent response from the right orbicularis oculi (RL) at an even lower intensity (0.2 mA). (d) Finally, by moving the probe paramedially to the left side, a clear response was recorded from both the left orbicularis oculi (LU) and oris (LL) at a similar intensity (0.2 mA). Middle lower panel: schematic summary of the mapping results (a–d) corresponding to the stimulating points illustrated in the upper panel. The conclusion was made that the actual location of the nuclei (green color) was more caudal than expected according to the normal anatomy (red color), especially on the left side, due to the tumor mass effect. Accordingly, the incision (I) was carried on transversally in correspondence of the stimulating point A. Right panel: postoperative contrast-enhanced T1-weighted MR images, sagittal (top), coronal (middle), and axial (bottom) view, showing complete removal of the tumor (Modified from Sala and Lanteri [61])
Similarly to what described for mapping the oculomotor nuclei in the midbrain, a handheld monopolar stimulating probe can be used. CMAPs are then elicited in the muscles innervated by the cranial motor nerves. To record the responses from cranial motor nerves VII, IX/X, and XII, wire electrodes are inserted in their innervated muscles, as described above for direct mapping of the peripheral cranial nerves. A single stimulus of 0.2-msec duration is delivered at a repetitive rate of 1–2 Hz. There are two different mapping strategies that can be used. One can look, for each site, at the threshold intensity which allows recording a CMAP. Moving the tip of the stimulator 1 mm apart, it is then possible to explore the floor of the fourth ventricle and identify the area with the lowest threshold (which is the one closer to either the nucleus or the intramedullary root of the nerve) and with the highest threshold or no response at all. These latter are likely the safer entry zones as the nuclei or tracts are far from the tip of the stimulator. The other possibility is to work with an intensity of approximately 0.5–1 mA and determine for each point the amplitude of the muscle response. The point corresponding to the highest amplitude indicates the vicinity of the mapped nucleus, while small amplitudes or, better, no response at all suggests a safe distance from the nucleus or tracts (Fig. 13.5). In any case, no stimulation intensity higher than 2 mA should be used to avoid cardiovascular derangements. Based on mapping studies, characteristic patterns of motor cranial nerve displacement, secondary to tumor growth, have been described (Fig. 13.6) [42]. These studies, although based on a small number of patients, suggest that motor nuclei dislocation is no random but rather corresponds to reproducible patterns so that the surgeon may to some extent predict where to look for the nuclei based on the preoperative MRI. Obviously, intraoperative confirmation with direct mapping is absolutely crucial.




Fig. 13.6
Displacement of facial nerve nuclei. (a) Sagittal and axial T1-weighted MR images of a bleeding upper pontine cavernoma. (b) Intraoperative view of the distorted floor of the fourth ventricle with no anatomical landmarks to identify the facial colliculi. Results of neurophysiological mapping are displaced. Responses from the right lower facial muscle, orbicularis oris, (RL) are recorded following monopolar stimulation with 2 mA (0.5 ms duration) at the upper asterisk. Responses from the right lower facial muscle, orbicularis oris, (RL) are recorded at lower intensity (0.5 mA) from the lower right asterisk, indicating a closer relationship with the right facial nerve nucleus or intramedullary root. Finally, responses from the left lower facial muscle, orbicularis oris, (LL) are recorded at low intensity (0.4 mA) from the lower left asterisk, indicating a close relationship with the left facial nerve nucleus or intramedullary root. (c) These results suggest a downward and lateral displacement of the facial nerve nuclei, consistent with the report of Morota et al. (see ref. [42])
Despite the relative straightforwardness of the fourth ventricle mapping technique and its indisputable usefulness in planning the most appropriate surgical strategy to enter the brainstem, postoperative functional outcome is not always predicted by postresection responses. In the case of mapping of the motor nuclei of the seventh cranial nerve, brainstem mapping cannot detect injury to the supranuclear tracts originating in the motor cortex and ending on the cranial nerve motor nuclei. Consequently, a supranuclear paralysis would not be detected, although lower motoneuron integrity has been preserved. Similarly, the possibility of stimulating the intramedullary root more than the nuclei itself exists. This could result in a false-negative peripheral response still being recorded despite an injury to the motor nuclei [41].
Mapping of the glossopharyngeal nuclei has also some limitations. Recording activity from the muscles of the posterior pharyngeal wall after stimulation of the ninth cranial nerve motor nuclei on the floor of the fourth ventricle assesses only the functional integrity of the efferent arc of the swallowing reflex. No information on the integrity of afferent pathways and afferent/efferent connections within the brainstem is provided, despite the fact that these pathways are indeed necessary to provide functions involving reflexive swallowing, coughing, and the complex act of articulation.
13.2.1.6 Brainstem Mapping for Fourth Ventricle Tumors
The same mapping techniques used to localize motor nuclei or cranial nerve intramedullary tracts during surgery for intrinsic brainstem tumors can also be used when dealing with tumors that either grow exophytically from the brainstem or grow primarily in the fourth ventricle and secondarily infiltrate the floor, entering the brainstem. In these cases brainstem mapping is used to decide when to stop removal rather than to select the entry zone to approach the tumor. Both in the case of a dorsally exophytic medullary glioma or of a fourth ventricle tumor, through a dorsal approach, the surgeon is faced first with the tumor, while the brainstem is displaced ventrally. In this situation, there is no point in mapping the nuclei at the beginning of the tumor removal, as these are ventral to the tumor, and CMAPs are likely not obtainable. Yet, when most of the exophytic component of the glioma or the intraventricular part of the tumor has been removed, it is important to avoid entering the brainstem as the lower cranial nerve nuclei are just a few millimeters underneath the infiltrated ependyma. At this point it is useful to alternate a piecemeal removal of tumoral tissue with periodical mapping of the tumor to see if a CMAP is elicited. Using a monopolar probe, current spreads in a way that if we use intensity around 1–1.5 mA, it is possible to have some response even when there is still margin for more tumor removal. However, from now on, great care should be taken to avoid irreversible damage to the nuclei and is recommendable to stop removing the tumor when the threshold drops to less than 0.7–0.5 mA. In this setting, it is extremely valuable to combine direct mapping with the continuous monitoring of the corticobulbar tracts (Fig. 13.7).




Fig. 13.7
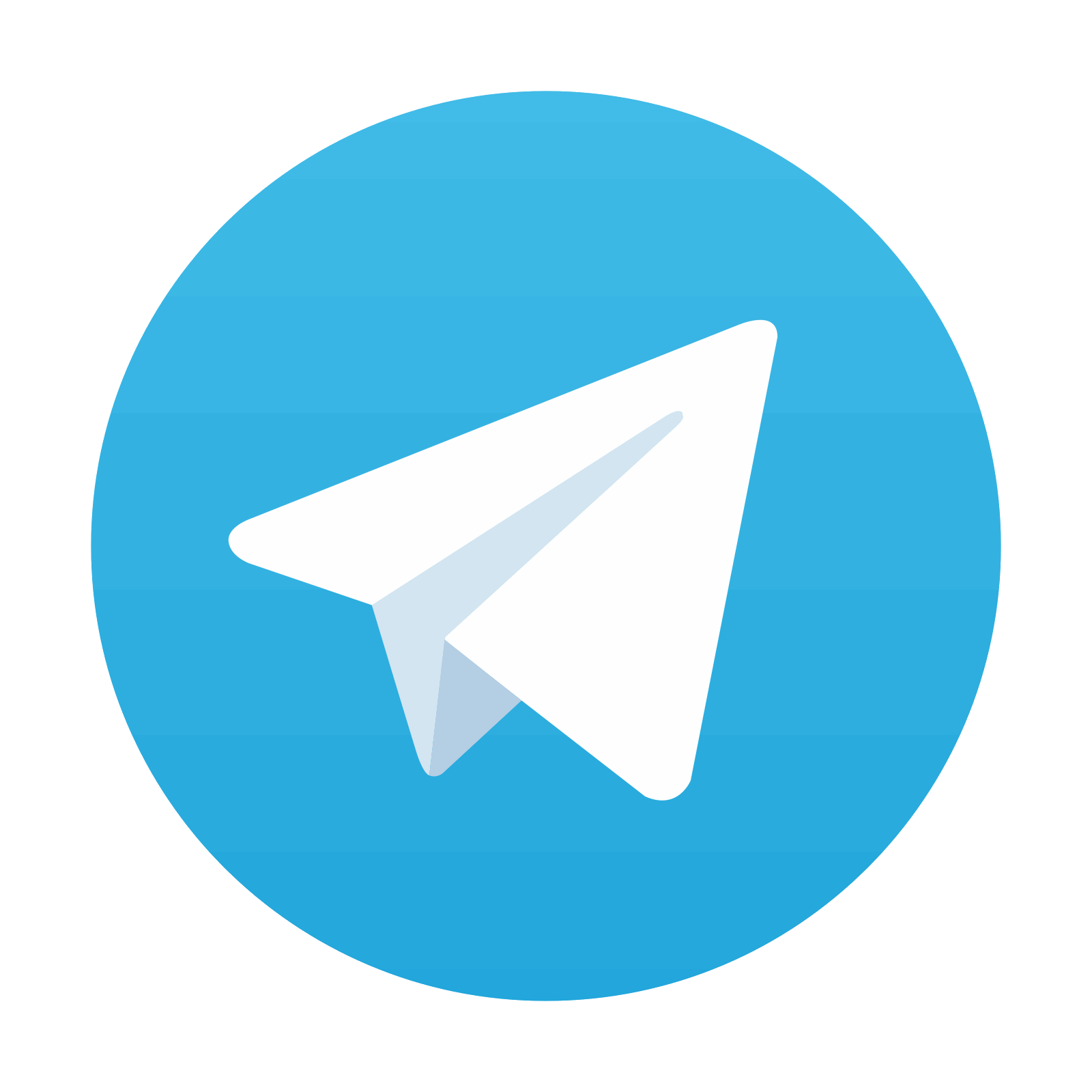
Monitoring and mapping during surgery for fourth ventricle tumors. (a) (top to bottom): sagittal, coronal, and axial gadolinium-enhanced T1-weighted MR images of a fourth ventricle ependymoma infiltrating the floor at the level of the calamus scriptorius. During surgery, corticobulbar motor-evoked potentials (MEPs) were continuously monitored from the hypoglossal muscles after transcranial electrical stimulation at C3/Cz and C4/Cz with a train of four stimuli at 60 mA. (b) While using the CUSA (left panel) a significant drop in the amplitude of the left hypoglossal MEP was observed (upper arrow) and persisted for several minutes. At this point, surgery was transiently stopped to facilitate recovery of the corticobulbar MEPs. (c) When the amplitude recovered and a more consistent left hypoglossal MEP was recorded (lower arrow), surgery was resumed. Yet, the microscopic view (let panel) suggested that the ependyma was infiltrated. (d) From now on, removal of little amount of tumor from the floor of the fourth ventricle was alternated with direct stimulation of the floor to localize the subependymal lower motor cranial nerve nuclei (left panel, surgical view). As soon as a clear compound muscle action potential (CMAP) was obtained from the left posterior wall of the pharynx muscles (glossopharyngeal/vagus complex) and the tongue muscles (hypoglossal nerve) (right panel), the decision was made to abandon surgery to avoid injuring the nearby nuclei. (e) (top to bottom): sagittal and coronal gadolinium-enhanced T1-weighted postoperative MR images showing gross total removal of the tumor and only a pinpoint enhancement at the bottom of the calamus scriptorius
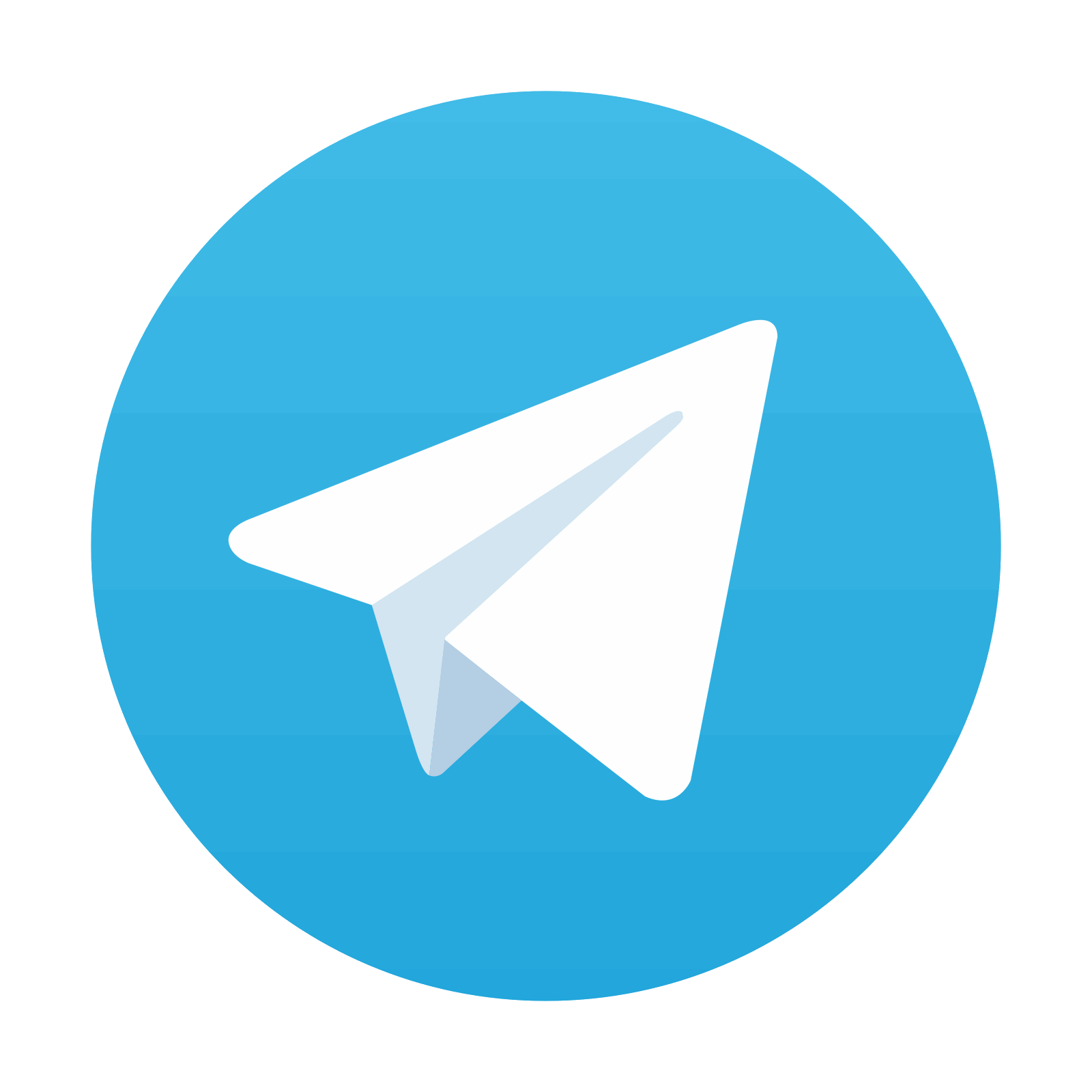
Stay updated, free articles. Join our Telegram channel

Full access? Get Clinical Tree
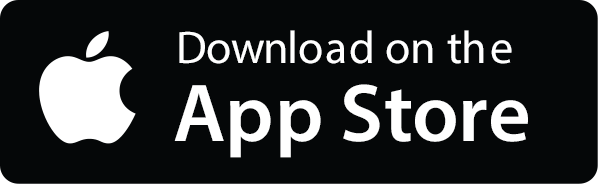
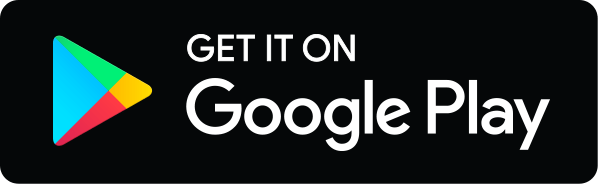
