Intraventricular Hemorrhage and Post-Hemorrhagic Hydrocephalus Intraventricular hemorrhage (IVH) remains the most common severe neurologic complication of preterm birth and presents significant life-long challenges for affected individuals. Approximately 12.1% of children born in the United States are born preterm, and very low-birth-weight infants are the most likely to develop IVH.1 At the time of its publication in 1978, the landmark paper by Papile et al documented that 45% of low-birth-weight infants developed IVH.2 Although marked improvements in medical care of the newborn have resulted in considerably lower IVH rates (currently 15 to 20%), the neurologic outcomes of infants who experience IVH are among the worst in neonatal medicine.3,4 Post-hemorrhagic hydrocephalus (PHH) occurs in up to one-half of those with IVH and is associated with a three- to fourfold increase in the risk for cognitive and psychomotor disability. Infants with PHH who require ventriculoperitoneal (VP) shunts have the worst neurologic outcomes, however, with neurodevelopmental impairments observed in more than 85% of infants and cerebral palsy in nearly 70%.5 In the current chapter, we review the recent literature regarding state-of-the-art diagnostic modalities, surgical and nonsurgical treatments, and outcomes observed in treating this disease. For additional information on the topics discussed herein, we refer the reader to the recent comprehensive review published by Dr. Shenandoah Robinson.6 In tracking the effect of advances in antenatal, perinatal, and neonatal care over the past two decades, the Neonatal Research Network recently reported a survival rate of 92% for infants born before 28 weeks’ postmenstrual age (PMA).4 IVH occurred in 16% of very preterm infants, with the incidence of IVH demonstrating an inverse correlation with PMA.4 The classification of IVH in premature infants is listed in ▶ Table 12.1. At 22 weeks’ PMA, 30% of preterm infants had IVH; conversely, at 28 weeks’ PMA, only 3% of patients had IVH.4 When the incidence of IVH in preterm infants was considered by grade,2 48% were classified as having grade 1 IVH, 18% had grade 2 IVH, 15% had grade 3 IVH, and almost 19% had grade 4 IVH.7 Although reports vary somewhat, it is estimated that 25 to 50% of infants with IVH develop post-hemorrhagic ventricular dilatation requiring neurosurgical treatment.8 As previously noted, severe IVH is associated with significant neurologic disability, particularly in those infants who develop PHH and require a VP shunt.5 These children frequently have severe cognitive impairments, cerebral palsy,9 and in many cases seizure disorders.1,10 Alterations in blood flow to the developing germinal matrix microvasculature are believed to be the pathophysiologic basis of IVH in premature infants.8 At the age when IVH is most likely to occur, key elements of the blood–brain barrier, including tight junctions, basement membrane, glial foot processes, and other supporting cellular structures, remain incompletely developed, leaving microvessels susceptible to disruption.12 Thus, when hypotension, hypoxemia, or other systemic insults occur, corresponding changes in cerebral blood flow in the germinal matrix may result in hemorrhage into the surrounding tissue and/or the ventricle. In support of this concept, a retrospective case–control study matching premature infants with and without hypotension found that hypotension was a risk factor for the development of IVH and neurologic injury.13 Furthermore, if significant parenchymal hematoma is present, particularly in combination with ventricular distention, parenchymal infarction also may occur (grade 4 IVH).8 In cerebrovascular development, vessel growth and maturation progress from the pial surface and extend into the parenchyma, a factor that may contribute to the susceptibility of the deep white matter to hypoperfusion during the newborn period.14 Additionally, the capacity for autoregulation, which is mediated primarily by small cerebral resistance vessels, develops relatively late, limiting the ability of these small vessels to attenuate alterations in cerebral blood flow.15,16 Data from experimental models confirm this temporal sequence, with regulation of blood flow to the germinal matrix improved with increasing gestational age.17 In the setting of hypotension, hypoxemia, hypercapnia, or acidosis, cerebral blood flow appears to be affected to a large degree by inflammatory mediators like transforming growth factor-β (TGF-β), interleukin-6 (IL-6), tumor necrosis factor-α (TNF-α), and other factors that affect the cyclooxygenase pathway (COX-2).6 Proinflammatory molecules affect not just cerebral blood flow but also blood–brain barrier permeability via the disruption of tight junctions and specific barrier proteins.12 Growth factors, cytokines, nitric oxide, and other molecules mediate these and other events, such as leukocyte endothelial transmigration and activation of microglia; in turn, reactive oxygen species are produced that cause endothelial injury and parenchymal infarction.18,19 Evidence is accumulating to suggest that genetic factors also may play a role in IVH. Gould et al have shown that mutations in the collagen 4A1 gene result in IVH in experimental models and are associated with hemorrhage and porencephaly in infants.20,21 Additionally, genetic mutations associated with thrombophilic conditions, such as mutations in the genes for prothrombin and factor V Leiden, also have been implicated in IVH and porencephaly.22,23 Finally, polymorphisms or mutations in the genes for proinflammatory cytokines, such as IL-6 and TNF-α, may also be involved in the pathogenesis of IVH, although this is controversial.24–26 It is likely that additional candidate genes with etiologic involvement in IVH will be identified in the near future. The mechanism(s) by which IVH results in hydrocephalus (PHH) remains to be determined. Although PHH may have both obstructive and nonobstructive components, the condition generally results at least in part from impairment of cerebrospinal fluid (CSF) absorption at the level of the arachnoid villus or other absorptive pathway. In preterm infants, arachnoid granulations are immature and thus capable of undergoing robust fibrosis from the blood products or inflammatory mediators associated with IVH.27 Blood degradation products and debris are thought to initiate an inflammatory process consistent with chronic arachnoiditis whereby laminin, collagen, and other extracellular proteins are deposited throughout the ependyma and subarachnoid spaces.27 TGF-β and other cytokines, together with free radical elaboration, trigger a cascade of arachnoid fibrosis and ependymal–subependymal gliosis.28 In support of this hypothesis, infants with PHH have been found to have significantly higher levels of TGF-β1 and an aminoterminal propeptide of type 1 collagen in their CSF.29,30 Vascular endothelial growth factor (VEGF) has also been implicated in the pathogenesis of PHH, although its role is less well understood.12 In many cases, this inflammation-based impairment of CSF absorption is compounded by ventricular outflow obstruction due to blood clots and debris within the ventricular system. The effect of IVH and PHH on neurodevelopment is multifactorial. The period in which IVH occurs (before 34 weeks’ PMA) is a critical neurodevelopmental interval, and neurologic injury during this time is likely to impair the proliferation and migration of neural precursors, neurite outgrowth and myelination, and functional network development.31 Clearly, the combination of ischemia–infarct and hematoma in germinal matrix is likely to cause significant injury to the nests of glial and neural precursor cells that reside in the subependymal zone.31 Furthermore, inflammation, cellular infiltration, and the generation of free radicals cause damage to the periventricular white matter and contribute to scarring and leukomalacia.32,33 Particularly in the setting of PHH, local increases in pressure and anatomical parenchymal distortion may affect development in the adjacent periventricular region, while a global increase in intracranial pressure (ICP) may impair cerebral perfusion and cause ischemia.6 IVH may range from clinically silent to catastrophic, but most cases that go on to require neurosurgical attention take a saltatory course, with hemorrhage occurring within the first hours to days after birth and then progressing for days to weeks.3,6 IVH is frequently diagnosed via screening cranial ultrasound and then monitored clinically. Although IVH may be associated with neurologic changes (e.g., decreased level of arousal, motor asymmetry, hypotonia, others), the development of PHH and thus increased ICP is heralded by changes in cranial parameters, including a bulging fontanel, increasing head circumference, and splaying of the cranial sutures. All of these metrics are examiner-dependent and somewhat subjective, but splaying of the sagittal suture may be the most reliable indicator.6 Later in the disease course, nonspecific but serious signs, such as apnea, bradycardia, and decreased spontaneous activity, may be observed (▶ Table 12.2). In cases of rapidly expanding hydrocephalus, ventricular enlargement may outpace increases in head circumference because of the relative compressibility of the white matter.34 At many centers, cranial ultrasonography is the primary imaging modality used to screen preterm infants for IVH. Cranial ultrasonography avoids ionizing radiation, is performed at the bedside, and permits adequate visualization of intracranial hemorrhage within the neonatal intensive care unit. The American Academy of Neurology practice parameter for neuroimaging of the neonate states that cranial ultrasonography should be performed at two time points (7 to 14 days and 36 to 40 weeks‘ PMA) to look for IVH, ventriculomegaly, and/or periventricular leukomalacia.35 Widely accepted measurements of ventricular size used in preterm infants include the ventricular index,36 anterior horn width, and thalamo-occipital distance.37 Normative data for these ventricular measures in the preterm infant are available; however, the optimal threshold for clinical treatment has not been determined.36,37 Magnetic resonance (MR) imaging is the method of choice for providing anatomical detail in the developing brain. Computed tomography (CT) is generally avoided when possible in the preterm infant because of the risks associated with repeated exposure of the developing brain to radiation.38 Conventional T1- and T2-weighted MR sequences provide high-resolution images of the ventricular system and of cortical and subcortical structures, and MR imaging has become an essential part in the evaluation of neurologic injuries and congenital malformations in the newborn. In recent years, advanced MR imaging techniques, such as diffusion tensor imaging (DTI), also have been developed to assess subtle alterations in white matter integrity that remain undetected by conventional MR imaging sequences. Diffusion tensor imaging measures the magnitude and directionality of water displacement in tissue and provides quantifiable measures of diffusion, fractional anisotropy, and mean diffusivity. Fractional anisotropy shows an exponential increase with neurodevelopment, whereas mean diffusivity shows a corresponding decrease during this interval.39 Deviation of fractional anisotropy and the apparent diffusion coefficient from normative values is indicative of white matter damage, as demonstrated in various neurologic disorders, including hydrocephalus.40,41 Whereas DTI is used to examine static white matter microstructure, resting-state functional connectivity MR imaging (fc MRI) utilizes low-frequency (< 0.1 Hz) fluctuations in the blood oxygen level–dependent (BOLD) signal to explore the functional neuronal networks.42,43 Although preliminary, fc MRI is now being applied in preterm infants to study the effect of newborn brain injury on functional cerebral architecture, and specifically the effect of pressure-based deformation in PHH on short- and long-term neurologic outcomes. Prevention of IVH and thus PHH is a primary goal in care of the preterm infant, and significant progress has been made in this regard. As noted above, a number of risk factors for IVH have been identified: early PMA and maternal hemorrhage/infection/inflammation, history of sepsis, hypotension, hypoxemia, hypercapnia, acidosis, pulmonary hemorrhage or pneumothorax, and respiratory distress syndrome (reviewed by McCrea and Ment).12 It follows that prevention of IVH is best achieved by preventing or mitigating these conditions. Of particular interest, lack of antenatal steroid treatment during preterm labor has been shown to be a risk factor for IVH, and a number of studies have shown that antenatal steroid administration reduces the risk for IVH.44 The mechanisms underlying this finding remain to be determined; however, it is likely that steroids attenuate increases in cerebral blood flow at the level of the germinal matrix through their effect on COX-2 and prostaglandins and assuage the inflammatory cascade that results in further disruption of the developing blood–brain barrier.12 Additionally, steroids promote maturation of the immature choroid plexus.45 Of note, however, a recent publication suggests that postnatal corticosteroids may increase the risk for neurodevelopmental difficulties with no associated reduction in risk for IVH, periventricular leukomalacia, or mortality.46 A number of other pharmacologic agents have been evaluated for their ability to prevent IVH (▶ Table 12.3). Phenobarbital, which prevents fluctuations in blood pressure and is thought to mitigate free radical–mediated injury, has been extensively investigated.12 Whitelaw and Odd performed a meta-analysis of 10 trials and found no reduction in IVH or PHH.47 Reduction of IVH was observed serendipitously when indomethacin, a COX inhibitor, was used to close patent ductus arteriosus in newborns. Indomethacin was believed to facilitate germinal matrix vessel maturation,48 improve cerebral autoregulation,49 and decrease ischemia.50 Indomethacin was investigated formally for use in preventing IVH. Although rates and severity of IVH were indeed lower with indomethacin, the effect on long-term neurologic outcome is less clear.51 In one trial, despite a decrease in IVH, no improvement in survival or neurosensory outcome was observed.52 A second trial demonstrated benefit in decreasing IVH rates and severity and increasing neurodevelopmental test performance in males only. Fowlie et al recently published an update of their meta-analysis of the effect of indomethacin on IVH.53 The COX inhibitor ibuprofen has a mechanism of action similar to that of indomethacin and appears to improve cerebral autoregulation in animals. In clinical trials, however, ibuprofen was ineffective in reducing IVH.54 Other pharmacologic agents evaluated for the prevention of IVH include vitamin E, ethamsylate, and pancuronium (Pavulon). Through different mechanisms, each has been shown to decrease the incidence of IVH, but not mortality or neurologic impairments (reviewed by McCrea and Ment).12 The efficacy of serial lumbar punctures for the treatment of PHH has been investigated extensively and also has been reviewed as part of the Cochrane Library.55 Kruesser et al (1985) initially reported using lumbar punctures (optimally 10 mL/kg per lumbar puncture) to decrease ventricular size in patients with post-hemorrhagic ventricular dilatation.56 A number of studies followed, including four prospective clinical trials, to investigate the effect of CSF removal on survival, hydrocephalus and requirement for a VP shunt, and neurodevelopmental outcome. Evaluating these data is difficult because of considerable variation in the subject enrollment criteria, the interventions themselves (some used lumbar punctures, some used ventricular tapping; the volume of CSF removed was inconsistent and often insufficient), and imprecision in the description of neurodevelopmental outcomes. However, meta-analysis of the available data failed to show a significant benefit of CSF removal in reducing the need for shunt surgery or neurologic disability.55 Nonetheless, lumbar punctures continue to be used clinically in infants showing obvious signs of increased ICP or as a temporizing measure in infants about to undergo placement of a ventricular access device. In such scenarios, 10 to 20 mL/kg is typically removed to decrease ICP; however, fibrosis or other factors often reduce the volume of CSF that can be removed. Concurrent with studies evaluating lumbar punctures for PHH, external ventricular drainage (EVD) was proposed as a method for the continuous management of hydrocephalus and control of ICP. One series reported on 37 infants with PHH treated with EVD for a mean of 21 days. Of these, 68% required VP shunts, but 67% had normal cognitive function and 33% had normal motor function.57 Although EVD continues to be used at some centers,58 this method has not reached widespread acceptance for the management of PHH, presumably because of the risk for infection and technical concerns regarding the integrity of the scalp in the preterm infant. The latter concern was recently addressed in a technical report in which a modified umbilical vessel catheter was used as an EVD in this setting.59 Because of the discouraging results from the studies investigating CSF removal described above, efforts were refocused on the pharmacologic treatment of post-hemorrhagic ventricular dilatation. Diuretics were utilized because of their potential to reduce CSF production.27 Early studies investigating acetazolamide, an agent already in use for this purpose in idiopathic intracranial hypertension, reported encouraging results60; however, a large multicenter randomized controlled trial utilizing acetazolamide and furosemide for post-hemorrhagic ventricular dilatation demonstrated no benefit to using these agents in terms of survival or need for shunt surgery in post-hemorrhagic ventricular dilatation.61 Furthermore, diuretic therapy was associated with marginally worse motor outcomes at 1 year and nephrocalcinosis. Ultimately, a Cochrane Library meta-analysis showed that diuretic use was neither safe nor effective in the treatment of PHH.60 The concept of DRIFT (drainage, irrigation, and fibrinolytic therapy) grew out the belief that the optimal treatment of post-hemorrhagic ventricular dilatation would not just reduce ICP but would also eliminate injurious intraventricular blood and blood breakdown products (e.g., iron) and proinflammatory cytokines. The DRIFT treatment involved placement of right frontal and left occipital ventricular catheters and then injection of low-dose tissue plasminogen activator, which was left in the ventricle for 8 hours. After the 8-hour interval, artificial CSF was continuously infused through the right frontal catheter, with egress of the fluid through the left occipital catheter set to maintain the ICP at 7 mm Hg. The infusion was continued until the CSF cleared, usually in 3 to 7 days.62 The short-term outcomes (up to 6 months of age or at hospital discharge) from this randomized controlled trial showed no reduction in shunt surgery or death in the DRIFT intervention arm. At interim analysis, the trial was stopped because of suspected treatment futility and an association of DRIFT with secondary IVH. In the long-term follow-up study, however, DRIFT was associated with a reduction in cognitive disability in survivors; 31% of infants had severe cognitive disability in the DRIFT group versus 59% in the conventional treatment group.63 These results have sparked a number of research efforts investigating optimal treatment approaches for post-hemorrhagic ventricular dilatation. In infants weighing less than 2 kg, a temporary ventricular access device is often inserted for the purpose of treating PHH. Perhaps the most common type of ventricular access device, the ventricular reservoir was first described in 1983 by McComb et al.64 Once implanted, ventricular reservoirs allow serial percutaneous access of the ventricular system. Serial taps for CSF removal are then used to treat clinical and radiographic signs of elevated ICP. In general, the volume of CSF removed is 10 mL/kg per tap (although up to 20 mL/kg is considered acceptable and safe). The volume removed and the frequency of removal are then tailored to each infant based on vital signs, physical parameters (head circumference, tenseness of the anterior fontanel, and splaying of sutures), and ventricular measures obtained from serial cranial ultrasound studies or other imaging. Others have reported using flow velocities on transcranial Doppler ultrasound to guide the appropriate timing and volume of CSF removal for PHH.65 Ventriculosubgaleal shunts (VSGSs) are an alternative method for drainage of the CSF and treating PHH-related increases in ICP.66,67 A VSGS comprises a ventricular catheter connected through a right-angle device to a small segment of distal catheter that is inserted into a large subgaleal pocket. Some surgeons include an in-series reservoir to permit tapping in the event of VSGS failure or a distal slit valve to provide limited back pressure and encourage physiologic CSF absorption. VSGSs provide continuous drainage of CSF into the subgaleal space and thus, in theory, maintain the ICP within a normal range on a constant basis. VSGSs are closed systems; tapping is not required, and fluid and electrolytes are not lost. Furthermore, because tapping is not required, VSGSs offer the potential for earlier hospital discharge with close follow-up. Like reservoirs, VSGSs have very low infection rates, although this rate may vary up to 6%.68 Of note, the life span of a VSGS is generally longer than 30 days, and after this interval, if the hydrocephalus has not resolved, the surgeon can opt to revise the VSGS (usually reopening the scarred-in subgaleal pocket) or to place a permanent VP shunt.67 To date, no study has demonstrated an advantage of VSGSs over ventricular reservoirs. Wellons et al (2009) presented their results from a four-center retrospective review of preterm infants being treated for PHH.58 In their series, 31 of 36 subjects (86%) initially treated with VSGSs required permanent VP shunting, compared with 61 of 88 (69%) treated with ventricular reservoirs. The difference in the shunt placement rates was statistically significant, suggesting that infants treated with ventricular reservoirs may have a lower rate of shunt dependence. A recent study at our institution found that the rates of shunt infection, shunt revision, and need for permanent shunt placement did not differ between patients with VSGSs and those with ventricular reservoirs.69 Consistent with the findings of Lam and Heilman, the observed rate of permanent VP shunt implantation in our series was lower in the VSGS group (66.7% vs. 75.4%, p = 0.38), although this difference was not significant.70 The selection of type of temporizing neurosurgical procedure (ventricular reservoir vs. VSGS) remains controversial and is the subject of an ongoing prospective clinical trial through the Hydrocephalus Clinical Research Network (Shunting Outcomes in Post-Hemorrhagic Hydrocephalus, or SOPHH). Also controversial is the timing of intervention for PHH. At present, no evidence-based guidelines exist to inform the decision of whether or when to intervene in PHH. Two multi-institutional, retrospective studies from a cooperative Dutch neonatal network focused on early treatment of PHH with CSF removal via lumbar punctures or ventricular reservoirs. Both reports demonstrated lower rates of neurologic disability and shunt dependence if CSF removal was initiated when ventricular size reached the 97th percentile71,72 rather than the 97th percentile + 2 standard deviations. The first study71 included 95 infants with post-hemorrhagic ventricular dilatation, 22 of whom did not develop progressive PHH. Five of 31 infants (16%) in the early treatment group and 26 of 42 infants (62%) in the late treatment group required VP shunts.71 Moreover, the number of infants with moderate or severe handicap was lower in the early treatment group (16%) than in the late treatment group (26%). The second study showed shunt dependence rates of 27.2% and 72.7% for the early and late intervention groups, respectively.72 The developmental quotient was also significantly lower in the late intervention group. Of note, both of these studies used lumbar punctures almost exclusively, rather than ventricular access devices, and the authors acknowledge that lumbar punctures were frequently insufficient to achieve the standard volume of 10 mL/kg per tap. Thus, these reports may underestimate the true impact of early intervention on shunt rate and neurologic deficit. Based in large part on these data, an international, multi-institutional prospective randomized controlled trial examining early treatment of PHH is currently under way (Early versus Late Ventricular Intervention Study, or ELVIS). Placement of a VP shunt is the permanent solution for the diversion of spinal fluid. Often, patients who have a shunt placed during their prenatal period have problems with malfunctions.73 Some have shown that shunt failure can occur in up to 12.6% of cases within the first 3 months of shunt placement.74 For these reasons, the timing for initial shunt placement has been extensively studied. Most believe that a shunt should be placed once the patient reaches a weight of approximately 2 to 2.5 kg. Some advocate that that the protein level should be less than 1.5 g/L, as well.27 However, the data are mixed, with another single-institution study showing no correlation between failure rates and spinal fluid protein and glucose levels. Hemorrhage and inflammation can also complicate shunt placement in these infants and can often lead to trapped ventricles. Depending on the site of obstruction, cranial nerve findings, ataxia, and changes in vital signs may develop. These situations often require the placement of complicated shunt systems, although neuroendoscopy both at our institution and others has become an accepted treatment for patients with these complicated problems. Some have advocated the use of endoscopic third ventriculostomy (ETV) with or without choroid plexus coagulation in the treatment of PHH.75–77 Although the complication rate in experienced hands is low, the data reported thus far have not shown ETV to be particularly effective in treating PHH, with fewer than 50% of procedures being successful.77,78 In a recent publication, Warf and colleagues demonstrated the importance of the prepontine cistern status (i.e., scarring present in the cistern) and the predictive value of FIESTA (fast imaging employing steady-state acquisition) MR imaging in applying ETV to PHH. Four of 10 patients in this study required no further shunt surgery, and the absence of scarring within the prepontine cistern correlated with a good outcome.75 In a paper relating success of ETV to hypoabsorption, Lipina et al were successful in 37.5% of their patients with PHH, and they found that low levels of TGF-β1 correlated with successful ETV.79 ETVs with or without choroid plexus coagulation may prove to be useful in selected patients; limited data exist, however, to advocate the widespread use of this procedure at this time. A major shortcoming limiting efforts to improve the treatment of PHH is the lack of quantifiable metrics to inform clinical decision making. Biomarkers are emerging in various fields as valuable predictors of clinical course and therapeutic response.80–82 In the future, protein markers in CSF, blood, or urine and noninvasive techniques such as DTI, fc MRI, and quantitative MR angiography in particular may serve as useful metrics for use in the standardization and optimization of PHH treatment.83 Elevated levels of nerve growth factor (NGF), neurotrophin-3 (NT-3), vascular endothelial growth factor (VEGF), erythropoietin (EPO), and soluble FAS have been observed in pediatric hydrocephalus of various types,84–87 and TGF-β elevations have been identified in PHH.29,88 More recently, advanced high-throughput proteomics techniques have been used to study CSF from infants with PHH, and a number of new candidate CSF markers of PHH and PHH-related neurologic disability have been identified, including L1CAM, APP, NCAM1, and brevican.89 Such markers may be used to complement existing ventricular measures in order to optimize the timing of therapeutic intervention or to monitor therapeutic response. Real-time functional data also may be obtained from amplitude-integrated electroencephalographic (aEEG) monitoring of ongoing cerebral electrical activity in the preterm infant. Olischar et al (2004, 2008) have suggested that aEEG tracings demonstrate characteristic changes as ventricular dilation progresses.90,91 This technology is particularly compelling as it may be useful in signaling real-time physiologic changes in advance of anatomical changes of PHH observed on imaging. Pearls
12.1 Epidemiology
Grade
Hemorrhage location
Ventricular involvement
Grade 1
Germinal matrix hemorrhage
< 10%
Grade 2
IVH with no ventricular expansion
< 50%
Grade 3
IVH with ventricular expansion
> 50%
Grade 4
Extension into parenchyma
Abbreviation: IVH, intraventricular hemorrhage. Source: Adapted from Papile LA, Burstein J, Burstein R, Koffler H. Incidence and evolution of subependymal and intraventricular hemorrhage: a study of infants with birth weights less than 1,500 gm. J Pediatr 1978;92(4):529–534.2
12.2 Pathophysiology
12.3 Clinical Presentation
Fontanel
Full, tense, nonpulsatile
Sutures
Split
Head circumference
Crossing percentile curves
Systemic symptoms
Irritable, less active, apnea, bradycardia
12.4 Imaging of Neonatal Hydrocephalus
12.5 Clinical Treatment
12.5.1 Prevention as Treatment for Post-Hemorrhagic Hydrocephalus
Effect on IVH
Agent
Effect on neurodevelopmental outcome
No effect
Phenobarbital
None
Ibuprofen
None
Postnatal steroids
Worsened
Decrease risk
Indomethacin
Unclear, may improve in males
Antenatal steroids
Improved
Vitamin E
None
Ethamsylate
None
Pancuronium (Pavulon)
None
12.5.2 Therapeutic Removal of Cerebrospinal Fluid via Lumbar Puncture for Post-Hemorrhagic Hydrocephalus
12.5.3 External Ventricular Drainage
12.5.4 Diuretics
12.5.5 The DRIFT Trial: Early and Late Outcomes
12.5.6 Temporizing Neurosurgical Procedures for Post-Hemorrhagic Hydrocephalus
Ventricular Reservoir
Ventriculosubgaleal Shunt
Ventriculoperitoneal Shunts
12.5.7 Endoscopic Third Ventriculostomy
12.5.8 Toward Improving the Outcome of Infants with Post-Hemorrhagic Hydrocephalus
Stay updated, free articles. Join our Telegram channel

Full access? Get Clinical Tree
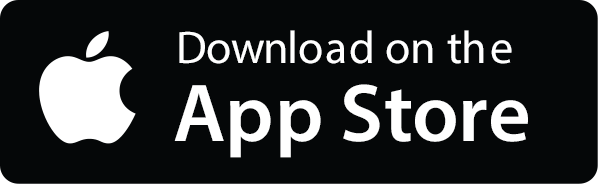
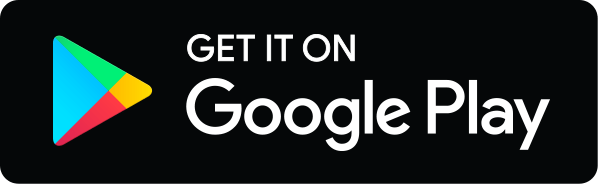