Chapter 11 Introduction
The inclusion for the first time of a specific Section on genetics in Principles and Practice of Sleep Medicine is a heralding event as it signifies that the study of the genetic control of the sleep–wake cycle is becoming an important approach for understanding not only the regulatory mechanisms underlying the regulation of sleep and wake but also for elucidating the function of sleep. Indeed, as genetic approaches are being used for the study of sleep in diverse species from flies to mice to humans (see Chapters 13 to Chapter 16), the evolutionary significance for the many functions of sleep that have evolved over time are becoming a tractable subject for research, as many researchers are bringing the tools of genetics and genomics to the sleep field. The complexity of the sleep–wake phenotypes and the difficulty in collecting phenotypic data on a large enough number of animals and humans to begin to unravel genetic mechanisms has in part been responsible for why few comprehensive attempts have been made to identify “sleep genes” beyond the circadian clock genes regulating the timing of sleep (see Chapter 12).
As noted by Landolt and Dijk (Chapter 15), sleep is a rich phenotype that can be broken down into a wide variety of sleep–wake traits based on the electroencephalogram (EEG) and the electromyogram (EMG).1 Furthermore, the genetic landscape for regulating multiple sleep–wake traits is clearly going to involve hundreds of genes and integrated molecular neurobiological networks.2 The fact that the environment also can have major effects on sleep–wake traits, particularly sleep duration in humans, also makes it difficult to uncover the underlying genetic control mechanisms. Indeed, while a large number of genome-wide association studies in humans have identified multiple genetic loci and genes involved in regulating a wide variety of physiologic systems and disease states, only a single relatively small and inconclusive genome-wide association study has been undertaken for sleep–wake traits in humans.3
A great deal of progress has been made in elucidating a number of circadian clock genes that regulate the diurnal timing of the sleep–wake cycle as well as most, if not all, of 24-hour behavioral, physiologic, and cellular rhythms of the body. The simplicity and ease of monitoring a representative “output rhythm” of the central circadian clock from literally thousands of rodents in a single laboratory, such as the precise rhythm of wheel running in rodents, was a major factor in uncovering the molecular transcriptional and translational feedback loops that give rise to 24-hour output signals.4,5 There is now substantial evidence demonstrating that deletion or mutations in many canonical circadian clock genes can lead to fundamental changes in other sleep–wake traits including the amount of sleep and the response to sleep deprivation.6 Indeed, as discussed in the Chapter 14, one can argue that many circadian clock genes are also “sleep genes.”
Another reason for the successful identification since the 1990s of the core clock genes in mammals is the remarkable conservation of the major clock genes from flies to mice to humans. Thus, core clock genes identified in flies, which involved mutagenesis and the screening of thousands of flies for mutant phenotypes, eventually led to finding the same genes in mice and humans. The relatively recent search for sleep genes in flies has been an active area of study since only about the turn of the century, and this approach is expected to uncover new sleep-related genes that will have mammalian orthologues. Indeed, the recent finding that different alleles of a core circadian gene, per, first indentified in flies, can affect the homeostatic response to sleep deprivation and the amount of slow-wave sleep (Chapter 15) argues that uncovering sleep genes in flies will directly lead to the genes underlying the changes in sleep–wake traits in mammals.
Early studies by Valtex and colleagues on inbred strains of mice7 and early human twin studies8 provided considerable evidence for a strong genetic basis for some sleep–wake traits, but little has been done to unravel the complex network of genetic interactions that must underlie this universal behavior in mammals. Tafti, Franken, and colleagues pioneered the use of quantitative trait loci in recombinant inbred mouse strains, which has led to the identification of a small number of genes that are associated with specific sleep–wake properties.9 More recently, the first attempt to record sleep in a large genetically segregating population of mice revealed considerable complexity to the genetic landscape for multiple sleep–wake traits.2 Using 2310 informative single-nucleotide polymorphisms, and assessing 20 sleep–wake traits in 269 mice from a genetically segregating population, led to the identification of 52 significant quantitative trait loci representing a minimum of 20 distinct genomic regions.2 Because this study involved only two inbred mouse strains contributing to the genetic diversity, it can be expected that many other loci will be identified once sleep is recorded in the offspring of different crosses of inbred and outbred mouse strains. Uncovering these loci, and understanding how interactions between genes and environment contribute to different sleep–wake states, is expected to not only reveal the molecular events underlying the sleep–wake cycle but also to yield new targets for drug discovery. New therapies based on the genetic control of sleep may be particularly important for treating genetic-based disorders of sleep (Chapter 16).
1 Tucker AM, Dinges DF, Van Dongen HPA. Trait interindividual differences in the sleep physiology of healthy young adults. J Sleep Res. 2007;16:170-180.
2 Winrow CJ, Williams D, Kasarskis A, Millstein J, et al. Uncovering the genetic landscape for multiple sleep–wake traits. PLoS One. 2009;4(4):e5161.
3 Gottlieb DJ, O’Connor GT, Wilk JB. Genome-wide association of sleep and circadian phenotypes. BMC Med Genet. 8(Suppl 1:59), 2007.
4 Vitaterna MH, King DP, Chang AM, Kornhauser JM, et al. Mutagenesis and mapping of a mouse gene, Clock, essential for circadian behavior. Science. 1994;264(5159):719-725.
5 King DP, Zhao Y, Sangoram AM, Wilsbacher LD, et al. Positional cloning of the mouse circadian clock gene. Cell. 1997;89(4):641-653.
6 Naylor E, Bergmann BM, Krauski K, Zee PC, et al. The circadian Clock mutation alters sleep homeostasis in the mouse. J Neuroscience. 2000;20(21):8138-8143.
7 Valatx JL, Bugat R, Jouvet M. Genetic studies of sleep in mice. Nature. 1972;238(5361):226-227.
8 Linkowski P, Spiegel K, Kerkhofs M, L’Hermite-Balériaux M, et al. Genetic and environmental influences on prolactin secretion during wake and during sleep. Am J Physiol. 1998;274(5 Pt 1):E909-E919.
9 Franken P, Chollet D, Tafti M. The homeostatic regulation of sleep need is under genetic control. J Neurosci. 2001;21(8):2610-2621.
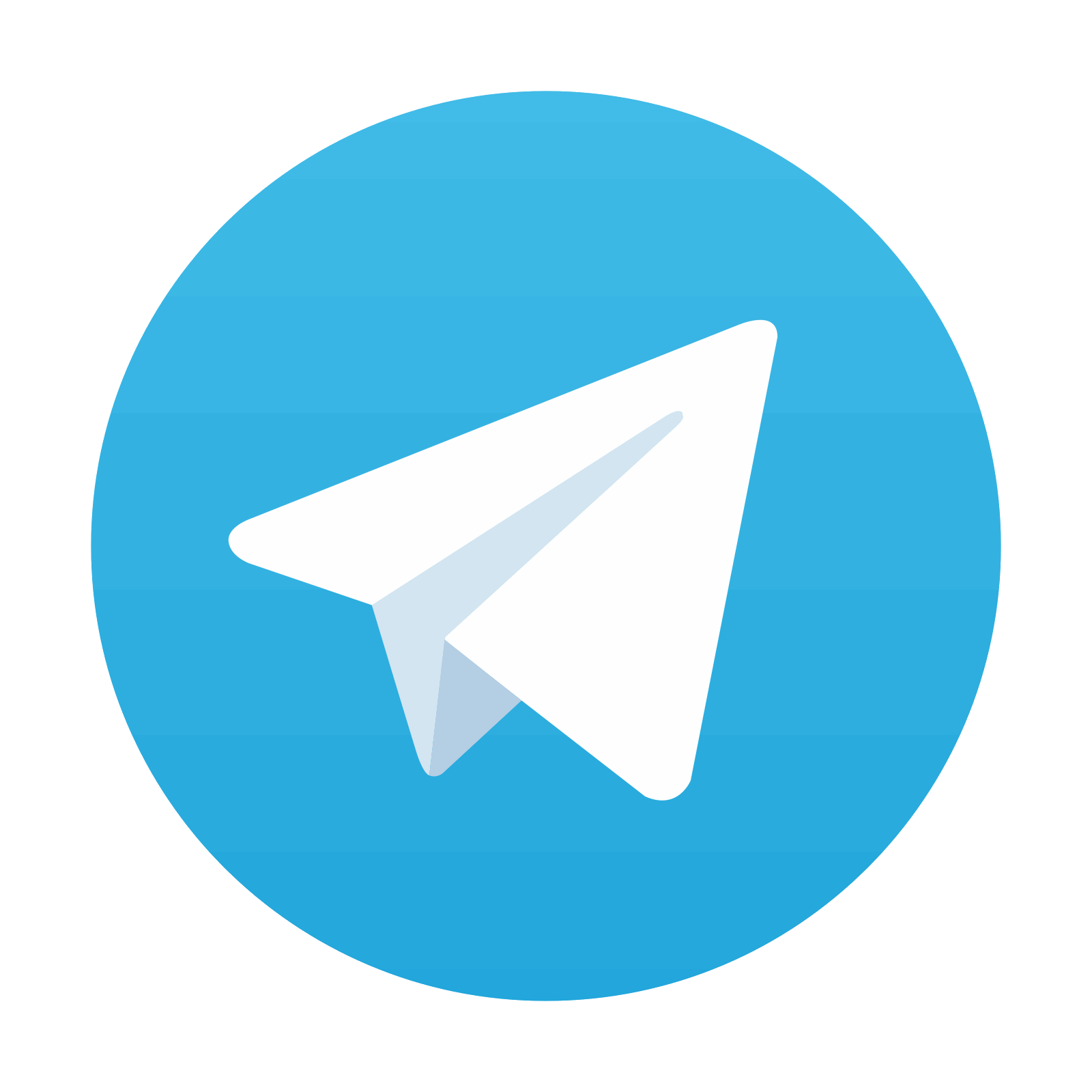
Stay updated, free articles. Join our Telegram channel

Full access? Get Clinical Tree
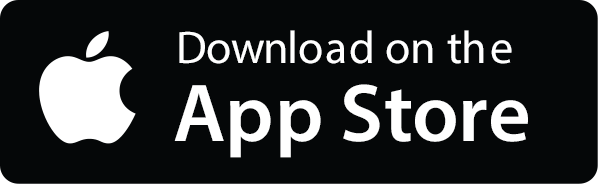
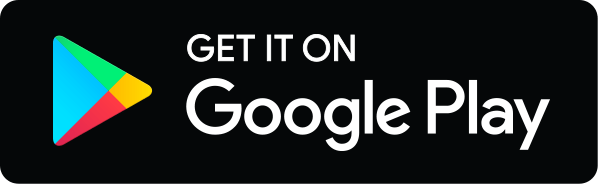