Vector
Capacity
Flexibility
Considerations
rAAV
4.7-5 kb
Tropism can be changed by pseudotyping and directed evolution.
The capsid may illicit an immune response when delivered peripherally, or with readministration centrally.
Limited to neurons, astrocytes, and oligodendrocytes.
Genome does not integrate, thus, infection of dividing cells will result in genome dilution.
Capacity can be enhanced by split genome approaches.
Retrovirus/ lentivirus
9 kb
Tropism can be changed by inserting various proteins in viral envelope.
Very easy to produce.
Virtually all cell types in the nervous system can be transduced.
Integrates into host cell genome, thus, viral genome dilution is not a concern.
Safety concerns remain.
Adenovirus
35 kb
Tropism can be altered by inserting various proteins in capsid.
Concerns with longevity of expression.
Transduces largely neurons and astrocytes.
Some concerns with natural cytotoxicity.
Very time consuming to produce.
DNA/ RNA
Unlimited
Uptake of nucleic acids is not sensitive to a particular cellular phenotype.
Does not utilize viral infection pathways and has to be delivered to target cells (e.g., electroporation or lipid-based transfection) .
Is sensitive to dilution by cell division.
1.1 Virus
Recombinant viral vectors are commonly used for the delivery of genetic material to the nervous system. Viruses have evolved over eons to bypass a host’s immune system and deliver its genetic material in to a host cell. Recombinant viral genomes are engineered to take advantage of the virus ’ ability to infect a host cell while removing the ability of the virus to replicate itself, or to produce damage to the host cell [1]. This book covers the three most commonly used viral vectors: adeno-associated virus (AAV ), lentiviruses (LV)/retroviruses, and adenovirus (Ad). Each of these vectors has its distinct advantages and disadvantages that need to be considered when planning an experiment.
1.1.1 AAV
AAV is by far the most commonly utilized gene therapy vector in the nervous system due to its strong transduction profile and its established safety profile both in animals and humans. Recombinant AAV (rAAV) retains only two small genetic elements from the wildtype genome: the two inverted terminal repeats (ITRs), which are required for the packaging of the viral genome in to the viral capsid . Since the discovery of AAV, a wealth of various AAV serotypes have been identified, each differing in the capsid surface. Viral entry to a host cell is mediated by interactions of the viral capsid with cell surface receptors, thus differences in capsid structure between serotypes result in viruses with unique cellular tropism [2, 3]. Therefore, today it is commonplace to package the AAV2 genome (ITRs) in the capsid that best suits the experiment. This is called pseudotyping, and the resultant vector is typically denoted rAAV 2/X, where 2 denotes the genomic origin of the ITRs and the X denotes the genomic origin of the capsid. AAV transduces neurons, astrocytes, and to a lesser extent, microglia. Based on the promoter chosen to express the transgene, expression can be guided to either of these cellular populations. One of the drawbacks of rAAV is the limited transgene capacity of the viral vector. Due to its small size, the AAV capsid can contain roughly ~4.7–5 kb of genetic material, and attempts to package genomes larger than this often result in lower packaging efficiency and reduced titers. Therefore, gene therapy approaches that require transfer of multiple genomes usually utilize viral vectors with greater transgene capacity such as lentivirus. One consideration with the use of rAAV is that the recombinant genome does not integrate. This means that if one aims to target dividing cells of the nervous system, genome dilution due to cell division will occur. In contrast, expression is maintained in non-dividing cells (i.e., neurons) for the lifetime of the cell. Another limitation to the use of AAV is that production, or packaging, is quite labor intensive and often requires specialized equipment (see Chapter 7).
1.1.2 Lentiviruses and Retroviruses
Retroviruses and lentiviruses (a subclass of the retrovirus family) are characterized by the ability to integrate in to the host-cell genome, and thus these vectors are not subject to genomic dilution with cell division. Although these two viruses are very similar, non-lentiviral retroviruses cannot traverse the nuclear membrane, thus their use is limited to only actively dividing cells. Lentiviruses on the other hand are a specialized subgroup of retroviruses which encode additional genes that facilitate nuclear import, and thus infect dividing and nondividing cells alike. Although the safety profile of the latest generation of retroviruses has been vastly improved, some safety concerns still remain: the generation of replication-competent lentivirus through recombination , mobilization (rescue and replication of the recombinant genome by the superinfection of another lentivirus such as HIV [4]), or insertional mutagenesis (such as the disruption of an essential gene or a tumor suppression gene by the insertion of the retroviral genome [5]). To combat the latter, integration-deficient LV can maintain stable expression in neurons in vivo [6]. However, remaining concerns has not precluded the use of these vectors in clinical trial s. Similar to rAAV, modifications can be made to the wild-type virus in order to change its tropism (i.e., pseudotyping). This is accomplished by inserting various surface glycoproteins into the viral envelope. The archetypical vector contains the vesicular stomatitis virus (VSV) G-protein (VSV-G ) in the envelope. But more recent studies have demonstrated an improved, and altered, tropism in the nervous system using surface proteins from other viral families such as Lyssavirus (rabies) and Filovirus (Ebola) [7–10]. The larger size of this family of viruses allows for the inclusion of roughly 9 kb of genetic material, nearly twice the transgene capacity of rAAV. Another distinct advantage to the use of LV and retroviruses in the laboratory is the ease of production, which consists of filtering and centrifugation of the culture media (see Chapter 8).
1.1.3 Adenovirus
Early-generation adenoviruses were plagued by cytotoxicity and immunogenicity, limiting the utility of this virus as a gene therapy vector. The latest generation of “gutless” vectors has had much of the viral coding sequence removed, providing for a safer and more efficient gene therapy vehicle. This high-capacity recombinant adenovirus (rAd) has the capacity to carry roughly 35 kb of genetic material making it an ideal vehicle for the delivery of large genes. However, for optimal packaging efficiency this virus requires a certain minimal amount of genetic material in the viral genome. Thus, if the transgene cassette is too small, specific “stuffer” DNA needs to be included in the viral genome [11]. Despite the fact that acute inflammation can be detected following stereotaxic delivery to the brain , transgene expression with gutless Ad persists for over 1 year [12, 13]. In contrast, the use of earlier generation Ad has been favored in the treatment of CNS neoplasms, such as glioblastoma multiforme, due to its natural cytotoxicity (see Chapter 31). Similarly, the natural immunogenicity of Ad has made it an ideal vector for vaccination studies. Comparatively, Ad production is relatively time consuming (see Chapter 9), restricting the use of this virus to studies and trials where the specific attributes of this vector are needed. Similar to rAAV and LV, recombinant adenoviruses can be pseudotyped by incorporating various attachment proteins on the viral surface resulting in enhanced neuronal tropism [14].
1.2 Non-viral
In contrast to the use of viral vectors, non-viral vectors do not rely upon the evolved capabilities of viruses to insert genetic material in to the target cell. Instead, non-viral gene-therapy relies upon the use of a physical force, or DNA conjugated to branched chemicals, in order to force the cell to take up the exogenous DNA, typically in the form of linear DNA, plasmid DNA, or siRNA . A variety of techniques have been devised to achieve this, including the gene gun, electroporation , or the use of branched molecules such as polyethylenimine [15]. Although this approach is beneficial in that it does not require the complexities involved in viral production, the efficacy and longevity of non-viral gene therapy are often inferior to viral-mediated gene therapy.
1.3 Produce or Purchase
Once a gene delivery vehicle has been selected, the second decision is whether to produce the vector in-house, or to utilize a core service (either academic or industry) to produce the vector. Moreover, if the choice to outsource vector production is made, what type of service is needed: Will you design, clone, grow, and send the viral genome to a core for packaging or will you utilize a “boutique” service where the complete process (genomic design, cloning , and packaging of the vector) is outsourced? This may depend on how frequently viral vectors will be utilized, as setting up production in-house can be both costly and time-consuming. However, once a laboratory has a working production protocol, outsourcing production is often more expensive and time consuming.
1.4 Growing of Genome
If one chooses to clone and grow the viral genome, there is a certain degree of esoteric knowledge that must be understood, as the cloning and handling of each type of viral vector genome carries with it certain nuances that are unique to that virus . For instance, the AAV and lentiviral genomes are contained within standard plasmids, and during the production of the recombinant virus these plasmids are inserted in to the production cell (e.g., HEK 293 cells) together with plasmids encoding helper functions and essential viral components (see Chapters 7 and 8). The AAV ITRs are genetic structural elements that are relatively unstable and readily recombine. However, this poses a problem as the ITRs are absolutely required for packaging of viable particles, and the functional titer is directly related to the ratio of plasmids with intact versus recombined ITRs. In order to grow these AAV genome plasmids, one must therefore utilize recombination deficient bacterial cells (e.g., SURE cells from Agilent). Moreover, reducing the growing temperature (from 37 °C to 30 °C) and the time of plasmid growth (from roughly 16 to 12–14 h) further improves the integrity of the population of ITRs. Finally, it is imperative that the integrity of the ITRs is checked prior to packaging using a restriction enzyme that gives a unique patterning following recombination (e.g., sma1 for AAV2 ITRs). The ITRs also create a structural barrier for certain manipulations of the viral genome. For instance, any type of mutagenesis that involves a PCR amplification of the entire bacterial plasmid is unlikely to succeed as the complimentary terminal repeats may cause PCR “skipping.” Thus, sub-cloning of the area of interest into a holding plasmid should be done prior to such manipulations. Similarly, the lentiviral LTRs (contained in the transfer plasmid) are also prone to recombination, thus, care should be taken while growing this plasmid in bacteria as well. In addition to the genomic component, when producing lentivirus, one must pay attention to what components must be provided in trans during packaging as the cis acting elements change with each generation of lentiviruses. For instance, the third generation of lentivirus requires the concomitant transfection of four plasmids during production: one transfer plasmid, two packaging plasmids, and one envelope plasmid.
Many plasmids containing viral genomes, helper and transfer plasmids, envelope plasmids, and various expression cassettes can be found at Addgene (http://www.addgene.org) or the National Gene Vector Repository (https://www.ngvbcc.org).
1.5 Handling and Storage
One of the greatest sources of discrepancies between seemingly identical gene therapy studies lies in how the virus is handled once it is produced. Again, this involves esoteric knowledge that is rarely published, if ever. For instance, both AAV and LV are sensitive to freeze-thawing, where the titer drops with each subsequent freeze cycle. In fact, in our hands we never freeze AAV, as this virus is stable at 4 °C for years. Similarly, following production, LV is immediately aliquoted and stored at −80 °C, but each aliquot is never re-frozen.
Outside a host cell, a viral vector can be defined as a complex protein/molecule. The protein structures that envelope these viruses are incredibly “sticky.” Accordingly, viral particles will aggregate in solutions with low ionic strength, or via the adherence of capsids to any contact surfaces. In order to prevent the loss of viral particles (and to avoid re-titering of the vector preparation) we have implemented certain precautions outlined in Chapter 14. Briefly, every surface that comes into contact with the virus is siliconized (e.g., by treating the surface with Sigmacote® (Sigma-Aldrich)). This reduces the surface charges which otherwise would facilitate virus binding. Alternatively, one can use an identical virus to “coat” surfaces that will come in contact with the vector. Regardless, despite taking all these measures, it is safe to assume that if a virus is transferred (e.g., aliquoted), that some virus will be lost to the procedure itself.
2 Target Cell Population
In designing your gene therapy experiment another important consideration is what population of cells you are aiming to transduce: Do I need to transduce only neurons, or astrocytes, or both? Is off-target transduction acceptable? How much volume of the brain do I need to cover? These questions highlight several variables that must be considered when designing your experiment in order to successfully implement a rational approach that best fits your particular situation: (1) the type and pseudotype of viral vector injected, (2) the expression cassette utilized to express your genetic payload, and (3) delivery method.
2.1 Pseudotype Variation
rAAV exhibits a very strong tropism for neurons, and to a lesser extent astrocytes. However, achieving microglial transduction using rAAV has proven difficult, and LV is a better viral candidate for this purpose. Regardless of whether you are using rAAV, LV, or Ad there are additional strategic variations that may be employed to further modulate the tropism of the viral vectors in order to target different cellular populations with various efficiencies. For instance, in the case of lentivirus, incorporating glycoproteins from lymphocytic choriomeningitis virus or Mokola virus in the envelope will efficiently guide expression specifically to neurons and astrocytes, respectively [7]. Similarly, by incorporating protein domains from canine Ad serotype 2 in to Ad5, the tropism of Ad shifts to neurons [14].
2.2 Expression Cassette
In many instances, ubiquitous expression is not a problem and the choice of expression cassette/promoter is largely dictated by the level of expression desired. However, in other instances researchers may need to guide expression to a phenotypically distinct cell type in the nervous system. In this case, specialized expression cassettes must be utilized.
Alternatives are possible, you to a specific subset of cells in the nervous system is in the choice of a specific promoter. A variety of both neuronal and non-neuronal promoters have been designed and validated in vivo (see Chapter 6). Moreover, the Pleiades Promoter Project (http://www.caneucre.org) [16] utilized a bioinformatics approach to design human mini-promoters (less than 4 kb) for a large number of proteins expressed in the brain . Although only a handful of these promoters have been validated in vivo, these promoter constructs are available in plasmid form through Addgene.
An alternate way of controlling expression is through the incorporation of micro RNA (MIR)-binding sites in an untranslated region of your transcript. The recognition of these cleavage sites results in the ablation of the transcript in cells expressing the specific MIR. For instance, the incorporation of MIR-9 (expressed in neurons but not microglia) binding sites effectively silences transcription in neurons [17].
Finally, FLEX vectors contain a loxP-flanked transgene coding sequence that is inverted with respect to the promoter (ON), or in line with the promoter (OFF). When used in combination with animals expressing CRE recombinase under the control of a phenotype-specific promoter, CRE-mediated recombination will “flip” the coding sequence either activating (ON) or disabling (OFF) transcription specifically within cells expressing CRE [18].
2.3 Delivery Method
Delivery methods are covered in more detail in Chapters 14–24. However, to some extent the delivery method can also dictate the type of cell that expresses the genetic payload. Direct stereotaxic injections of either Ad, LV, or rAAV have the benefit of containing the viral vector within a confined volume (with some spread along white matter tracts). Ventricular delivery of rAAV in the neonate transduces large portions of the CNS , but the cell specificity depends on the serotype and the age of the animal during the injection [19]. Similarly, systemic delivery of rAAV transduces either neurons or astrocytes, or both, again depending on the age of the animal and the serotype used. However, systemic delivery of rAAV also transduces a wide range of organ systems outside of the nervous system [20, 21], and care must be taken as this may confound the study.
2.4 Enriching Vector Targeting via Directed Evolution
In some instances, the approaches outlined in this chapter do not result in the effective transduction of certain populations of cells within the nervous system. In an effort to effectively target hard-to-infect cells, a recent approach has been to use standard molecular evolution techniques to generate viral capsid libraries based on the capsids of the various serotypes of AAV [22]. Following the injection of these highly diverse viral populations in to animals, scientists can isolate the cell/or tissue of interest, and from those infected cells/tissue, isolate and sequence the specific capsid mutations that facilitated the transduction. Perhaps one of the best examples of this methodology has been the identification of capsids that effectively transverses the seizure-affected mature blood–brain barrier [23].
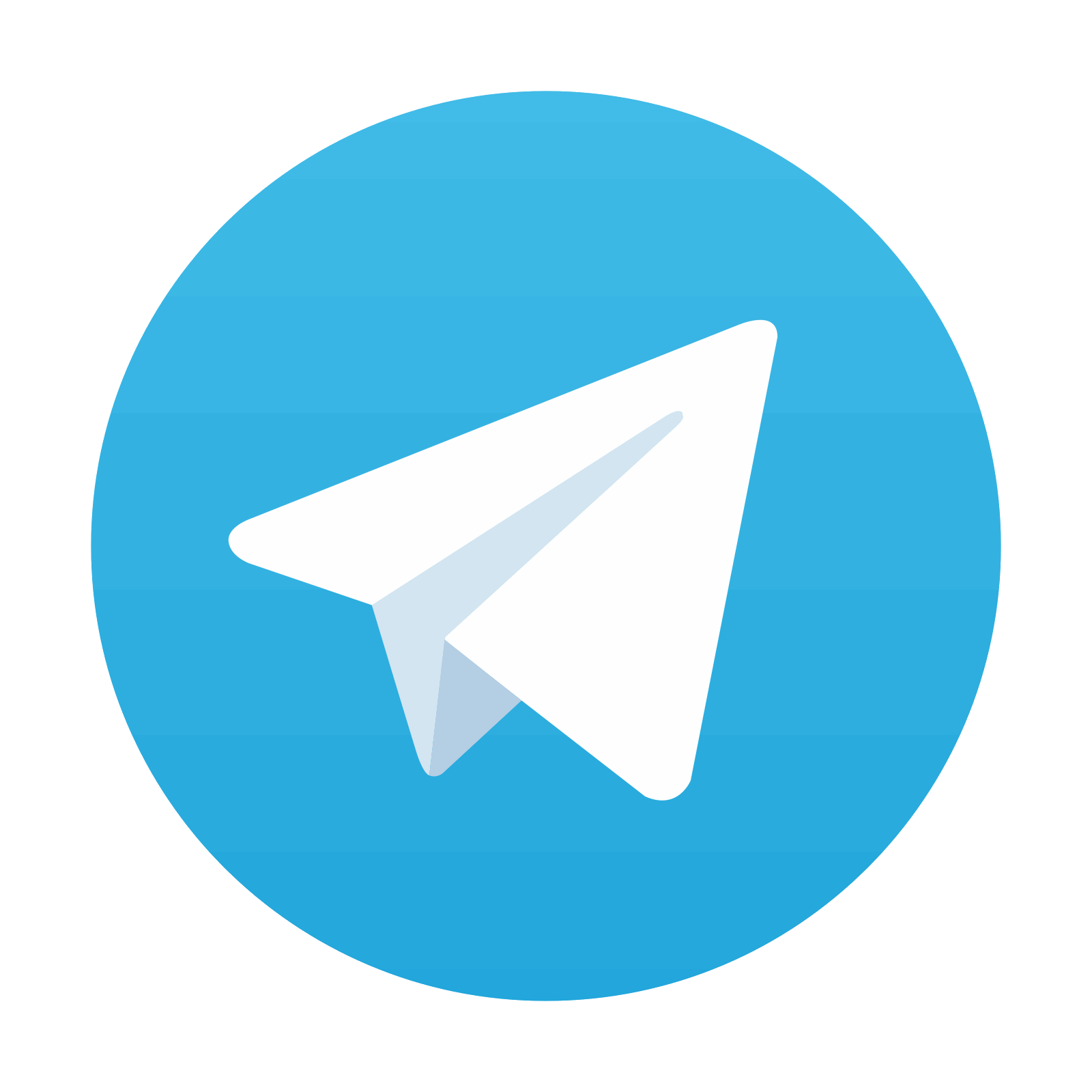
Stay updated, free articles. Join our Telegram channel

Full access? Get Clinical Tree
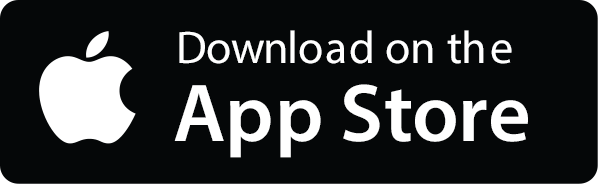
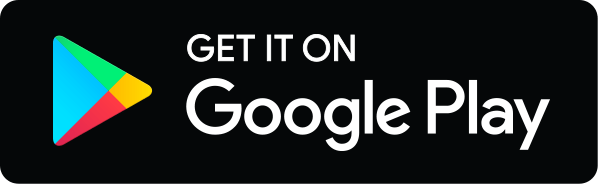