Early schematics of a “mobile stroke unit” first developed in Homburg. Dimensions indicated in millimeters. (a) Integrated small CT, (b) operation console, (c) isolation against radiation produced by the CT, (d) metal free stretcher as CT table
In this chapter, we summarize up-to-date evidence for the prehospital acute ischemic stroke care. We highlight challenges and solutions in prehospital stroke care and focus on innovations with the Mobile Stroke Unit.
2 The Prehospital Phase
2.1 Engaging the Stroke Rescue Chain
The prehospital phase refers to the stroke diagnosis and management before the patient arrives at the hospital. It starts with symptom onset and includes the activation of EMS, onsite management and transport to the target hospital. Though various structural models exist, effective prehospital care systems must have certain core elements. Important components of the prehospital stroke rescue chain are early identification of stroke symptoms, early activation of EMS, recognition by dispatchers and paramedics, effective onsite diagnosis and management, and effective prenotification of the target hospital. Prehospital management is augmented by mobile telemedicine for remote clinical examination and imaging, integration of CT scanners and point-of-care laboratories in ambulances [34].
2.2 Prenotification
Prenotification of the target hospital regarding an en route stroke patient is an evidence-based measure to accelerate in-hospital management and is recommended by international guidelines [4, 5]. MSUs enable the prehospital team to provide the target hospital with detailed information about the nature of the stroke and any additional information to expedite subsequent specialized treatment.
In the Get With The Guidelines registry, EMS personnel provided prenotification to the target emergency department for 67% of the total 371,988 stroke patients. EMS prenotification was associated with an increased likelihood of thrombolytic treatment within 3 h (82.8% vs. 79.2%, P < 0.0001), shorter door-to-imaging times (26 vs. 31 min, P < 0.0001), shorter door-to-needle times (78 vs. 80 min, P < 0.0001), and shorter symptom onset-to-needle times (141 vs. 145 min, P < 0.0001). EMS hospital prenotification was associated with better clinical evaluation, timelier treatment, and more eligible patients treated with thrombolysis [35].
2.3 Primary Stroke Centers and Comprehensive Stroke Centers: Drip and Ship and Mothership
The organization of acute stroke care has evolved significantly during the past few decades [36]. Primary stroke centers (PSCs) have been implemented to improve stroke care. PSCs include acute stroke teams, stroke units, written care protocols, and an integrated emergency response system [37]. Comprehensive stroke centers (CSCs) integrate specialized services for the management of severe cerebrovascular disease. These are typically staffed with experts in neurointervention and vascular neurology, have advanced neuroimaging capabilities including MRI and cerebral angiography, specialize in surgical and endovascular techniques (including clipping and coiling of intracranial aneurysms, carotid endarterectomy, and intra-arterial thrombolytic therapy), and have specific infrastructure such as an intensive care unit [38].
As of 2017, comprehensive stroke centers accounted for roughly one-third of all stroke centers in the USA (327 of 1148) and in France (37 of 132) [39–41]. In 2011, 66% of Americans were within a 60-min ground transfer to a primary stroke center and 81% to an intra-arterial therapy (IAT) capable hospital. However, only 56% of Americans had 60 min ground transfer proximity to a CSC [39]. As a result, in some settings it is difficult to admit patients directly to CSC, especially with standard ambulance services. As a proposed solution, patients could receive intravenous therapy in a hospital before being transferred to a CSC for mechanical thrombectomy. This is known as the drip-and-ship paradigm, in contrast to direct admission to a CSC, referred to as mothership.
Along with the development of specialized stroke units, there have been significant advances in reperfusion techniques. Intravenous thrombolysis has been the leading reperfusion method with its efficacy window having been extended to 4.5 h after symptom onset [42–44]. Previously, mechanical thrombectomy was limited to patients with basilar artery occlusions and those with contraindications to intravenous thrombolysis [45–48]. Recently, there has been growing evidence supporting the use of mechanical thrombectomy in a greater proportion of patients—including those with occlusion of the proximal anterior circulation—as time windows for efficacy grow up to 24 h since last known normal [9, 49–51]. With an increasing proportion of patients becoming eligible for mechanical thrombectomy, it becomes important to optimize patient transport from the emergency site either by drip-and-ship or mothership. Further to these is bridging therapy whereby intravenous therapy within 4.5 h is followed by mechanical thrombectomy within 6 h of symptom onset [39]. There is evidence from clinical trials supporting bridging therapy over intravenous thrombolysis for patients with large vessel occlusion (LVO) of the anterior circulation [52–57]. These approaches to patient transfer have important implications for the optimization of prehospital care.
3 The Mobile Stroke Unit
3.1 The Mobile Stroke Unit Concept
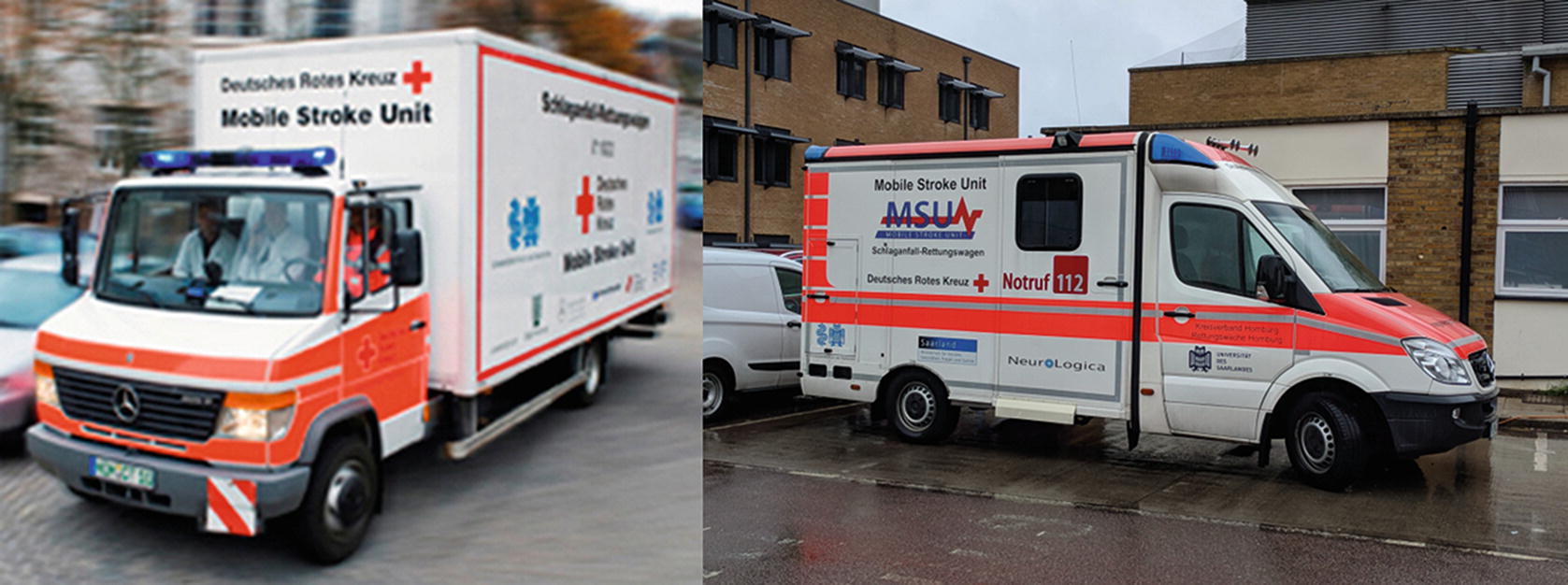
Generations of mobile stroke units used in Homburg
The MSU strategy allows for the administration of treatment directly at the emergency site. Treatments include thrombolysis for acute cerebral ischemia, anticoagulant reversal for acute intracranial hemorrhage, management of physiological variables for ischemic or hemorrhagic stroke, and management of further emergencies [31]. The MSU extends specialized stroke care to the prehospital phase of stroke management.
Acute stroke management involves multidisciplinary cooperation. Healthcare professionals (including paramedics, nurses, radiographers, technicians, physicians) are required to quickly collect medical history, perform clinical examinations, and handover information. In a hospital setting, these professionals may be working in different locations and join the patient care pathway at different times. The resulting handovers and multiple interfaces can contribute to errors and delays. With the MSU concept, crucial time is saved by substantially reducing those interfaces. In the MSU, a single, specialized, interdisciplinary team, consisting of paramedics, physicians, nurses, and technicians, can perform a complete diagnostic workup and acute treatment. The MSU acts synergistically with hospital stroke units to close the existing treatment gaps.
3.2 The Mobile Stroke Unit Ambulance
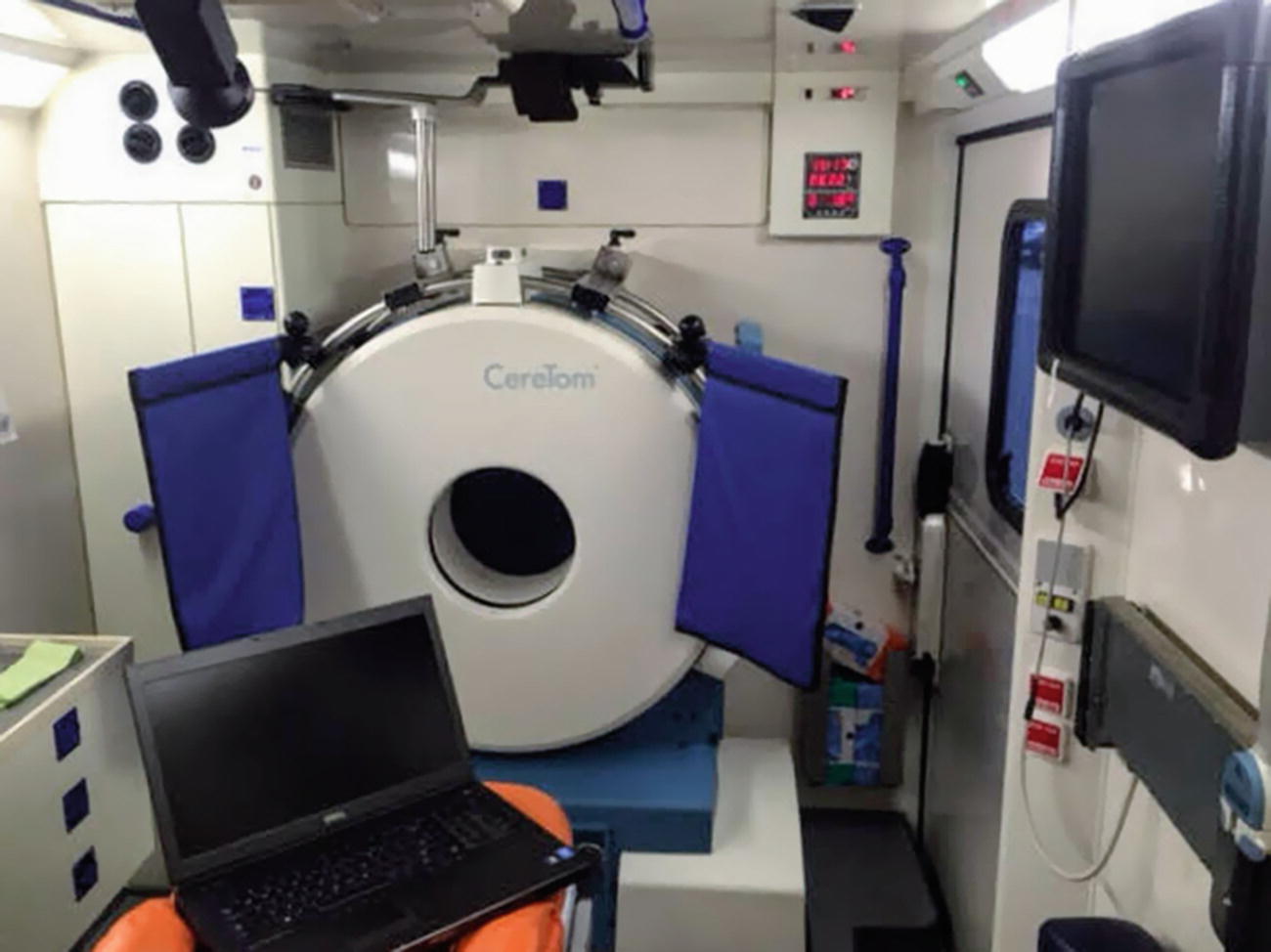
Homburg Mobile Stroke Unit interior with CT scanner. The Mobile Stroke Unit is an ambulance which contains a multimodal CT scanner, a point-of-care laboratory, as well as a telemedicine system, which allows for transfer of CT images and videos of patient examination to the clinical information system as well as a bidirectional communication
Subsequent iterations of the MSU have adapted to their respective settings. Larger vehicles have the advantage of carrying larger scanners, robustness in rural off-road conditions, and allowing relatives to accompany patients to provide history and procedural consent [59]. Smaller vehicles may have greater access to narrow roads and lower cost. With this in mind, vehicle models should be selected according to the specific needs of the region and healthcare setting [31].
3.3 Prehospital Brain Imaging
Imaging is important for triage and management of acute stroke. Broadly, in acute events, ischemic stroke (IS) must be reliably distinguished from hemorrhagic stroke (HS) [4, 5]. This distinction is necessary for initiation of thrombolysis and for transport decisions [30].
Mobile Stroke Units benefit from advances in portability and image quality of mobile CT scanners. Multimodal imaging (non-contrast CT, CT angiography, and CT perfusion) has been integrated into existing MSU programs [31, 33]. There are several portable CT scanners on the market, including the CereTom (Neurologica), Tomoscan M (Philips, Eindhoven, The Netherlands), and Somatom Scope (Siemens). Images from most portable CT scanners are of sufficient quality for assessment of the parenchyma and for CT angiography of the intracranial circulation including the circle of Willis. The Somatom Scope, currently in use in Memphis, allows assessment of proximal neck vessels and the aortic arch [31].
The CereTom scanner is in use on the Homburg MSU. The CereTom is an 8-slice small-bore portable CT scanner that allows for non-contrast, angiography, and contrast perfusion. As a space saving measure, the scanner’s gantry—rather than the patient—moves along the craniocaudal axis. As a result, a dedicated translating exam table is not required [60]. Furthermore, CereTom operates with an on-board 120 V rechargeable battery pack and can be plugged into a standard 110 V outlet obviating the need for a high-voltage power supply [61].
Both size and weight are factors in selecting a suitable scanner. For example, the CereTom scanner measures 1.5 × 0.7 × 1.3 m and weighs 362 kg (about 800 lbs). At present, more detailed scanners exceed size and weight limitations for roadworthy ambulances. It is likely that with continued innovation, more detailed and advanced scanners may be suitable for MSUs in the future as scanner size decreases and vehicle size increases.
Endovascular intervention is becoming more suitable for an increasing population of patients [50, 62]. However, not all primary stroke centers (PSCs) are equipped and staffed for endovascular neurointervention. This poses a challenge for prehospital triage. Preferably, patients with large vessel occlusion (LVO) should be sent to endovascular-capable centers directly. Congruently, patients who are unlikely to have an LVO or undergo thrombectomy should be transported to PSCs closest to their homes.
Despite continued progress, it remains difficult in a prehospital setting to reliably identify LVO clinically [63–65]. Advances in imaging in the prehospital phase provide an opportunity for screening patients for LVO. CT-Angiogram (CTA) enables prehospital diagnosis of severe strokes with LVO and can aid in guiding transport decisions for the patient [66, 67]. For non-contrast CT (NCCT), the Alberta Stroke Program Early CT Score (ASPECTS) is a systematic approach used to assess early ischemic changes within the middle cerebral artery territory [68]. However, limitations due to interrater variability and scan quality impact its utility [53]. Automated assessment by artificial intelligence (AI) based software, such as e-ASPECTS (Brainomix, Oxford, UK), may facilitate rapid and remote assessment of images [69]. CT Perfusion may have clinical utility in the prehospital selection of candidates for endovascular therapy as it may have greater sensitivity than NCCT in identifying the ischemic changes [70].
3.4 Prehospital Point-of-Care Testing
Laboratory testing is an important component of stroke care. However, in many cases, it is more important to not delay the administration of thrombolysis due to laboratory testing. According to leading guidelines, blood glucose is the only assessment which must precede the initiation of thrombolysis in all patients [5]. If there is strong suspicion of coagulopathy, tests such as international normalized ratio (INR), activated partial thromboplastin time (aPTT), and platelet count, may be necessary. Other recommended tests are leukocyte and erythrocyte counts, hemoglobin levels, gamma-glutamyltransferase (GGT) and pancreatic amylase activity [31]. Creatinine level is helpful for concerns regarding renal function [30]. Still, thrombolysis should not be delayed while waiting for hematological or coagulation testing if there is no reason to suspect an abnormal test considering the low risk of coagulopathy in the general population. In the same vein, baseline troponin assessment is recommended but should not delay initiation of thrombolysis [5].
Point-of-care testing (POCT) allows for faster results than use of the hospital laboratory system [71–73]. In a German study, the use of a point-of-care laboratory has been shown to decrease time from door-to-therapy decision (end of all diagnostic procedures) from 84 min (SD 26) to 40 min (SD 24, P < 0.0001) compared with use of a centralized hospital laboratory [28]. With that in mind, further studies are needed to clarify the relevance of point-of-care results on treatment decisions. Further advantages of POCT are portability, simple measurement procedures, use of low sample volumes, and automated data processing. POCT devices require extensive testing, validation, and conformity with legislative directives and health standards before they can be used in a clinical setting [31].
POCT devices are valuable and adaptable to the MSU setting. In the Homburg trial, the laboratory system allowed for measurement of platelet count, leukocyte count, erythrocyte count, hemoglobin, and hematocrit (PocH 100i, Sysmex, Hamburg, Germany), international normalized ratio and activated partial thromboplastin time (Hemochron Jr., ITC, Edison, NY, USA), and gamma-glutamyltransferase, p-amylase, and glucose (Reflotron plus, Roche Diagnostics, Mannheim, Germany) [26]. Similar POCT has been used in MSU trials in the USA [74].
3.5 Telemedicine: Communicating with the Ambulance
Telecommunication approaches between standard ambulances and the stroke center via systems can provide real-time remote specialist advice. Telemedicine includes telestroke assessment (real-time bidirectional videoconferencing and high-speed transmission of videos) and teleradiology (transmission of high-quality images) [31]. Commercially available systems allow MSUs to transmit digital imaging and communication data to hospital records. MSUs provide a valuable resource to rural and remote settings where patient may not have easy access to in-hospital stroke care [75].
However, there are limitations to the management tasks which can be carried out remotely. The treatment of acute stroke in an MSU is a complex exercise involving multiple parallel tasks being carried out expediently by several healthcare professionals within the confined space of an MSU. This includes neurological assessment, monitoring of vital signs, patient positioning, management of patient comfort and possible restraint, CT scanning, point-of-care laboratory testing, and medication preparation and administration [74]. Furthermore, the clinical decision on whether to administer thrombolysis requires training, experience, and careful clinical judgment.
Nevertheless, limitations due to EMS staffing, cost-effectiveness and geographic accessibility necessitate telemedicine. EMS response varies between the majority of European and US settings. In the USA, ambulances are typically not staffed by physicians. As a result, in developing an MSU program, a decision has to be made as to whether to include an on-board physician. Early experience from Houston and Germany suggests the ratio of MSU alerts (from EMS dispatch) to tPA treatments is at least 10 to 1, making it impractical to have a vascular neurologist aboard the MSU for all calls [74].
The Pre-hospital Utility of Rapid Stroke Evaluation Using In-Ambulance Telemedicine (PURSUIT) study assessed the feasibility and accuracy of telemedicine assessment of actors simulating patients with stroke in ambulances [76]. Remote, real-time assessments of National Institutes of Health Stroke Scale (NIHSS) showed absolute agreement for intraclass correlation (ICC) of 0.997 (95% CI 0.992–0.999) and matching within two points of NIHSS occurred for 88% of scenarios.
As part of the BEST-MSU study, 174 patients were simultaneously, independently assessed by an on-board vascular neurologist and a telemedicine vascular neurologist to evaluate interrater variability. There was 98% satisfactory connectivity and 88% agreement on the thrombolysis decision [77]. This level of agreement is comparable to two vascular neurologists evaluating the same patients face-to-face in the emergency department [78].
Technical innovation in the transmission of data between the hospital and MSU plays an important role. Early studies encountered difficulties in telecommunication in part due to suboptimal 3G public network availability [76, 79–82]. Fortunately, with improved technology and 4G mobile systems, telecommunication is becoming more reliable [31]. Telemedicine encounters between the MSU and hospital has been shown to be successfully completed for 99% of patients with 4G connectivity [83].
In the iTREAT study, a low-cost, tablet-based platform and commercial cellular networks (4G/LTE) were used to reliably perform prehospital neurologic assessments (NIHSS) of actors in both rural (central Virginia) and urban settings (San Francisco Bay Area) via videoconferencing [84]. With innovation, it is important to ensure that bidirectional telecommunication is encrypted and secure, and meets the standards for transmission of protected health information.
3.6 Staffing the MSU
Emergency Medical Services (EMS) staffing varies greatly across different countries [85, 86]. In many European settings, EMS services are staffed by a physician. In contrast, in many other countries, including most US settings, ambulances are staffed by paramedics with no physician present [17, 31]. MSU staffing, accordingly, must be adapted to disparity in local legislation. Additionally, to date most MSUs operate in addition to conventional emergency medical services due to legislative limitations [31]. Staffing conventions also impact the cost-effectiveness and sustainability of an MSU practice. In the USA, an MSU with a physician present may not be cost-effective [87]. However, remote physician assessment through the use of telemedicine may serve as a safe and reliable workaround [77]. Most research projects to date have included a vascular neurologist on board the ambulance [33]. However, with increasingly reliable telecommunication, other staffing configurations with different combinations of paramedics, nurses, radiographers, neurologists, and neuroradiologists are possible via telemedicine [31].
In the Homburg model, the MSU team includes a paramedic, a stroke physician, and a neuroradiologist. Additionally, conventional EMS which includes an emergency physician for critically ill patients accompanies the MSU [26]. In Houston, the MSU is staffed by a paramedic, a certified CT technician, a vascular neurologist, and a registered nurse experienced in both clinical research and acute stroke management [74].
In Norway, anesthesiologists trained in the prehospital clinical assessment of acute stroke patients and the interpretation of CT imaging have reliably been able to identify radiological contraindications for thrombolysis and also identify subarachnoid hemorrhage [88–90].
3.7 Dispatching the MSU
Early symptom recognition is essential for timely care in stroke. Once engaged, EMS dispatchers have an important role in identifying and prioritizing stroke patients for the most efficient use of this specialized vehicle. Education programs for EMS dispatchers increase the number of stroke patients treated in timely manner [17]. Shortening times from call-to-dispatch, dispatch-to-ambulance arrival, on-scene time, and scene-to-hospital transport are all important. In a study of 184,179 cases in the USA, median time from call to ED arrival is 36 min (IQR 28.7–48.0) with the longest component being on-scene time (15 min). Strikingly, dispatch identified only 52% of cases as stroke [91].
Identification of potential stroke patients by telephone is a challenging task undertaken by EMS dispatchers [17, 92]. Several prehospital stroke screening scales have been developed and employed in an effort to assist dispatchers in identifying stroke patients with the greatest specificity and sensitivity. Recognition of Stroke in the Emergency Room (ROSIER) scale (sensitivity 93%, specificity 83%) and Dispatcher Identification Algorithm of Stroke Emergency (DIASE) (sensitivity 53%, specificity 97%) have been employed in this regard [93–95]. Furthermore, there are several scales which assist EMS personnel in the early identification of stroke, namely the Cincinnati Pre-Hospital Stroke Scale (CPSS), Los Angeles Pre-Hospital Stroke Screen (LAPSS), Melbourne Ambulance Stroke Screen (MASS), Medic Prehospital Assessment for Code Stroke (Med PACS), Ontario Prehospital Stroke Screening Tool (OPSS), and Face Arm Speech Test (FAST) [94].
4 Mobiles Stroke Units for Time Saving
4.1 MSU Trial Evidence
Results from the first MSU trial conducted in Homburg, Germany demonstrating time savings across all metrics
MSU group (n = 53) | Control group (n = 47) | P value | Difference (95% CI) | |
---|---|---|---|---|
Therapy decision | ||||
Alarm to therapy decision (min) | 35 (31–39) | 76 (63–94) | <0.0001 | 41 (36–48) |
Symptom onset to therapy decision (min) | 56 (43–103) | 104 (80–156) | <0.0001 | 43 (30–58) |
Intravenous thrombolysis | ||||
Alarm to intravenous thrombolysis (min) | 38 (34–42) | 73 (60–93) | <0.0001 | 34 (23–54) |
Symptom onset to intravenous thrombolysis (min) | 72 (53–108) | 153 (136–198) | 0.0011 | 80 (40–115) |
Intravenous thrombolysis or IAT | ||||
Alarm to intravenous thrombolysis or intra-arterial recanalization (min) | 38 (34–42) | 78 (61–110) | <0.0001 | 44 (27–73) |
Symptom onset to intravenous thrombolysis or intra-arterial recanalization (min) | 72 (53–108) | 152 (135–209) | <0.0001 | 80 (46–115) |
End of CT | ||||
Alarm to end of CT (min) | 34 (30–38) | 71 (62–87) | <0.0001 | 38 (33–43) |
Symptom onset to end of CT (min) | 56 (43–103) | 97 (74–156) | <0.0001 | 39 (26–52) |
End of laboratory analysis | ||||
Alarm to end of laboratory analysis (min) | 28 (26–34) | 69 (55–81) | <0.0001 | 38 (32–44) |
Symptom onset to end of laboratory analysis (min) | 51 (40–95) | 99 (70–140) | <0.0001 | 39 (26–56) |
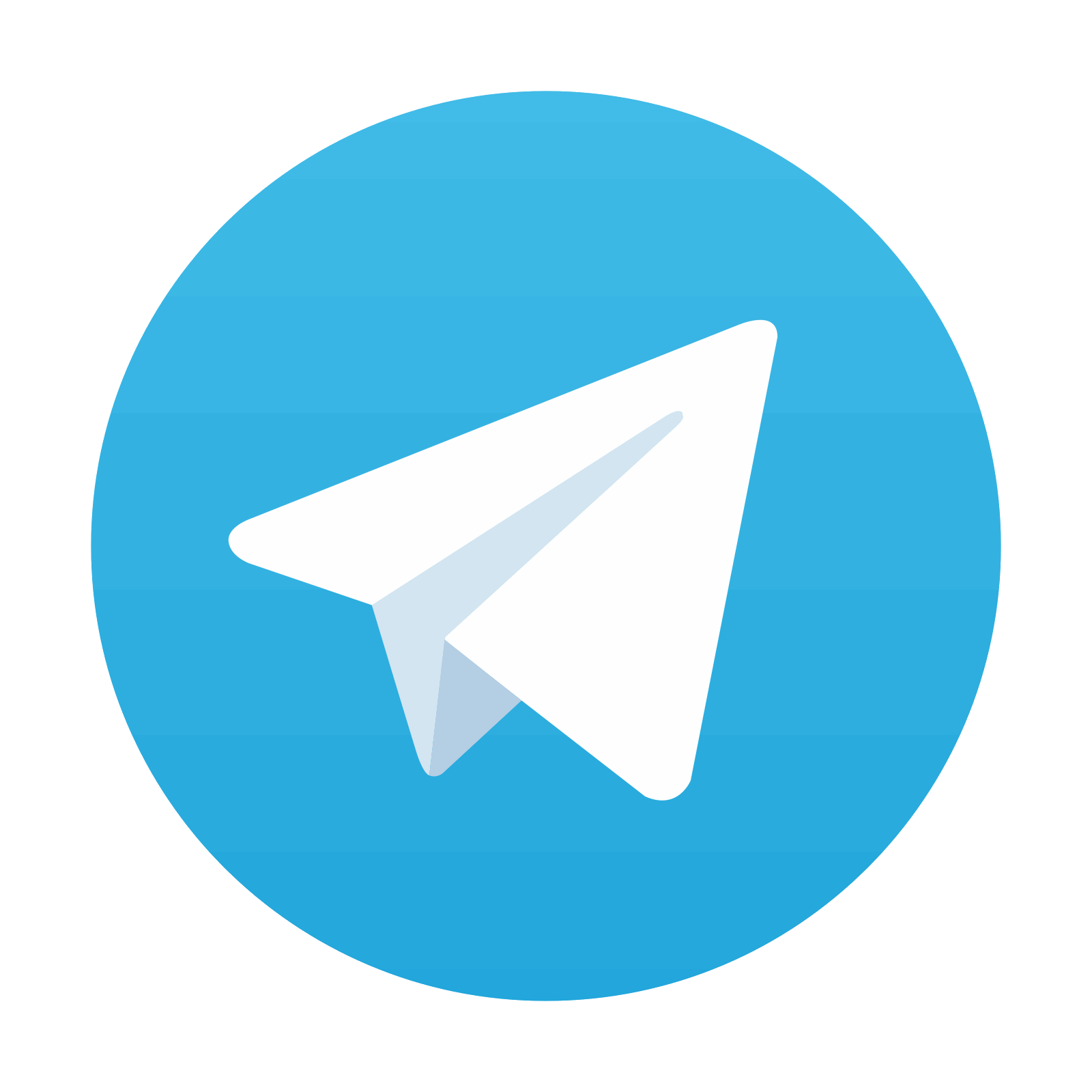
Stay updated, free articles. Join our Telegram channel

Full access? Get Clinical Tree
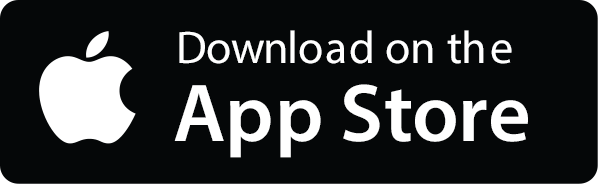
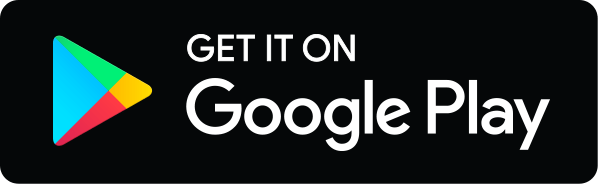