Learning and Memory
Short-Term and Long-Term Memory Involve Different Neural Systems
Short-Term Memory Maintains Transient Representations of Information Relevant to Immediate Goals
Short-Term Memory Is Selectively Transferred to Long-Term Memory
Long-Term Memory Can Be Classified As Explicit or Implicit
Explicit Memory Has Episodic and Semantic Forms
Explicit Memory Processing Involves at Least Four Distinct Operations
Episodic Knowledge Depends on Interaction Between the Medial Temporal Lobe and Association Cortices
Implicit Memory Supports Perceptual Priming
Operant Conditioning Involves Associating a Specific Behavior with a Reinforcing Event
Associative Learning Is Constrained by the Biology of the Organism
Errors and Imperfections in Memory Shed Light on Normal Memory Processes
IN HIS MASTERFUL NOVEL One Hundred Years of Solitude Gabriel Garcia Marquez describes a strange plague that invades a tiny village and robs people of their memories. The villagers first lose personal recollections, then the names and functions of common objects. To combat the plague, one man places written labels on every object in his home. But he soon realizes the futility of this strategy, because the plague eventually destroys even his knowledge of words and letters.
This fictional incident reminds us of how important learning and memory are in everyday life. Learning refers to a change in behavior that results from acquiring knowledge about the world, and memory is the process by which that knowledge is encoded, stored, and later retrieved. Marquez’s story challenges us to imagine life without the ability to learn and remember. We would forget people and places we once knew, and no longer be able to use and understand language or execute motor skills we had once learned; we would not recall the happiest or saddest moments of our lives, and would even lose our sense of personal identity. Learning and memory are essential to the full functioning and independent survival of people and animals.
In 1861 Pierre Paul Broca discovered that damage to the posterior portion of the left frontal lobe (Broca’s area) produces a specific deficit in language. Soon thereafter it became clear that other mental functions, such as perception and voluntary movement, are also mediated by discrete parts of the brain (see Chapter 1). This naturally led to the question: Are there discrete neural systems concerned with memory? If so, is there a “memory center” or is memory processing widely distributed throughout the brain?
Contrary to the prevalent view that cognitive functions are localized in the brain, many students of learning doubted that memory is localized. In fact, until the middle of the 20th century many psychologists doubted that memory is a discrete function, independent of perception, language, or movement. One reason for the persistent doubt is that memory storage involves many different parts of the brain. We now appreciate, however, that these regions are not all equally important. There are several fundamentally different types of memory, and certain regions of the brain are much more important for some types of storage than for others.
During the past several decades researchers have made significant progress in the analysis and understanding of learning and memory. In this chapter we focus on three insights. First, there are several forms of learning and memory, each with its distinctive cognitive properties and mediated by specific brain systems. Second, memory can be deconstructed into discrete encoding, storage consolidation, and retrieval processes. Finally, imperfections and errors in remembering can provide clues about the nature and function of learning and memory.
Memory can be classified along two dimensions: (1) the time course of storage and (2) the nature of the information stored. We shall first consider the time course.
Short-Term and Long-Term Memory Involve Different Neural Systems
Short-Term Memory Maintains Transient Representations of Information Relevant to Immediate Goals
When we reflect on the nature of memory we usually think of the long-term memory that William James referred to as “memory proper” or “secondary memory.” That is, we think of memory as “the knowledge of a former state of mind after it has already once dropped from consciousness.” This knowledge depends on the formation of a memory trace that is durable, in which the representation persists even when its content has been out of conscious awareness for a long period.
Not all forms of memory, however, constitute “former states of mind.” In fact, the ability to store information depends on short-term memory, called working memory, which maintains current, albeit transient, representations of goal-relevant knowledge. In humans working memory consists of at least two subsystems—one for verbal information and another for visuospatial information. The functioning of these two subsystems is coordinated by a third system called the executive control processes. Executive control processes are thought to allocate attentional resources to the verbal and visuospatial subsystems, and to monitor, manipulate, and update stored representations.
We use the verbal subsystem when we attempt to keep speech-based (phonological) information in conscious awareness, as when we mentally rehearse a phone number just obtained from an operator. The verbal subsystem consists of two interactive components: a store that represents phonological knowledge and a rehearsal mechanism that keeps these representations active while we need them. Neuropsychological and neuroimaging data indicate that phonological storage depends on posterior parietal cortices, and rehearsal partially depends on articulatory processes in Broca’s area.
The visuospatial subsystem of working memory retains mental images of visual objects and of the location of objects in space. The rehearsal of spatial and object information is thought to involve modulation of such representations in the parietal, inferior temporal, and extrastriate occipital cortices by the frontal and premotor cortices. Current research is concerned with whether visuospatial working memory might best be viewed as two subsystems, one for object knowledge and one for spatial knowledge.
Single-cell recordings in nonhuman primates indicate that some prefrontal neurons maintain spatial representations, others maintain object representations, and still others represent the integration of spatial and object knowledge. Although neurons concerned with working memory of objects tend to fall in the ventrolateral prefrontal cortex and those concerned with spatial knowledge tend to fall in the dorsolateral prefrontal cortex, all three classes of neurons are present in both prefrontal subregions (Figure 65–1).
Figure 65–1 The prefrontal cortex maintains a working memory. (Adapted, with permission, from Rainer, Asaad, and Miller 1998.)
A. The role of prefrontal cortex in maintaining information in working memory is often assessed in monkeys using electrophysiological methods in conjunction with the delayed-match-to-sample (DMS) task. In this type of task each trial begins when the monkey grabs a response lever and fixates a small target at the center of a computer screen. An initial stimulus (the “sample”) is briefly presented and must be held in working memory until the next stimulus (the “match”) appears. In the task illustrated here the monkey was required to remember the sample (“what”) and its location (“where”) and release the lever only in response to stimuli that “matched” on both dimensions.
B. Neural firing rates in the primate lateral prefrontal cortex during the delay period in the task are often above baseline and represent responses to the type of stimulus (what), the location (where), and the integration of the two (what and where). At left is the activity of a prefrontal neuron to preferred objects (to which the neuron responds robustly) and to nonpreferred objects (to which the neuron responds minimally) during the task. Activity is robust when the monkey encounters the preferred object (sample) and during the delay. In the sketch at right the symbols represent recording sites where neurons that maintained each type of information (what, where, and what and where) were found. Typically, several types of neurons were found at one site; hence many symbols overlap and some symbols indicate more than one neuron.
Short-Term Memory Is Selectively Transferred to Long-Term Memory
In the mid-1950s startling new evidence about the neural basis of long-term memory emerged from the study of patients who had undergone bilateral removal of the hippocampus and neighboring regions in the medial temporal lobe as treatment for epilepsy. The first and best-studied case was a patient called H.M. studied by the psychologist Brenda Milner and the surgeon William Scoville. After H.M. died on December 2, 2008, his full name, Henry Molaison, was revealed to the world.
H.M., a 27-year-old man, had suffered for more than 10 years from untreatable temporal lobe epilepsy caused by brain damage sustained at age 7 years in a bicycle accident. As an adult his seizures rendered him unable to work or lead a normal life. At surgery Scoville removed the hippocampal formation, the amygdala, and parts of the multimodal association area of the temporal cortex bilaterally (Figure 65–2). After the surgery H.M.’s seizures were better controlled, but he was left with a devastating memory deficit (or amnesia). What was so remarkable about H.M.’s deficit was its specificity.
Figure 65–2 The medial temporal lobe and memory storage.
A. The key components of the medial temporal lobe important for memory storage.
B. The areas of temporal lobe resected (gray shading) in the patient known as H.M., viewed from the ventral surface of the brain (left hemisphere is on the right side of the image). Surgery was a bilateral, single-stage procedure, but to illustrate the structures that were removed the right side of the image is shown here intact. The longitudinal extent of the lesion is shown in a ventral view of the brain (top). Cross sections 1 through 3 show the estimated extent of areas of the brain removed from H.M. (Adapted, with permission, from Corkin et al. 1997.)
C. Magnetic resonance image (MRI) scan of a parasagittal section from the left side of H.M.’s brain. The calibration bar at the right of the panel has 1-cm increments. The asterisk indicates the resected portion of the anterior temporal lobes. The arrowhead points to the remaining portion of the intraventricular portion of the hippocampal formation. Approximately 2 cm of preserved hippocampal formation is visible bilaterally. Note also the substantial degeneration in the enlarged folial spaces of the cerebellum. (Adapted, with permission, from Corkin et al. 1997.)
He still had normal working memory, for seconds or minutes, indicating that the medial temporal lobe is not necessary for transient memory. He also had long– term memory for events that had occurred before the operation. He remembered his name, the job he had held, and childhood events, although his memory of information acquired in the years just before surgery was not robust. In addition, he retained a command of language, including his vocabulary, indicating that semantic memory was preserved. His IQ remained unchanged in the range of bright–normal.
What H.M. now lacked, and lacked dramatically, was the ability to transfer new information from working memory into long-term memory. He was unable to retain for lengthy periods information about people, places, or objects that he had just encountered. Asked to remember a new telephone number, H.M. could repeat it immediately for seconds to minutes because of his intact working memory. But when distracted, even briefly, he forgot the number. H.M. could not recognize people he met after surgery, even when he met them again and again. For several years he saw Milner every month, yet each time she entered the room he reacted as though he had never seen her before. H.M. is not unique. All patients with extensive bilateral lesions of the limbic association areas of the medial temporal lobe from either surgery or disease show similar long-term memory deficits.
H.M. is a historic case because his deficit provided the first clear link between memory and the medial temporal lobe, including the hippocampus. Given the large size of the hippocampus proper, the question next arose: How extensive does a bilateral lesion have to be to produce a memory deficit? Clinical evidence from several patients as well as data from experimental animals suggests that a lesion restricted to any of the major components of the system can have a significant effect on long-term memory. For example Larry Squire, David Amaral, and their colleagues found that the patient R.B. had only one detectable lesion after a cardiac arrest—destruction of the pyramidal cells in the CA1 region of the hippocampus. Nevertheless, R.B.’s memory deficits were qualitatively similar to those of H.M., although quantitatively much milder.
The different subregions of the medial temporal lobe, which together comprise the medial temporal lobe memory system, may not have equivalent roles, however. For example, some areas in the medial temporal lobe circuit may be particularly important for object recognition. Damage to the perirhinal cortex that spares the underlying hippocampus produces a greater deficit in object recognition than do selective lesions of the hippocampus that spare the overlying cortex.
In contrast, some theorists have argued that the hippocampus may be relatively more important for spatial representation than for object recognition. In mice and rats lesions of the hippocampus interfere with memory for space and context, and single neurons in the hippocampus encode specific spatial information (see Chapter 67). Functional imaging of the brain in healthy humans shows that activity increases in the right hippocampus when spatial information is recalled, and in the left hippocampus when words, objects, or people are recalled. These physiological findings are consistent with the clinical observation that lesions of the right hippocampus give rise to problems with spatial orientation whereas lesions of the left hippocampus cause defects in verbal memory.
Long-Term Memory Can Be Classified As Explicit or Implicit
Another crucial finding about H.M. was that not all types of long-term memory were impaired. Even though H.M. and other patients with damage to the medial temporal lobe had profound memory deficits, they were able to form and retain certain types of durable memories as well as healthy subjects.
For example, H.M. learned to draw the outlines of a star while looking at the star and his hand in a mirror (Figure 65–3). Like healthy subjects learning to remap hand-eye coordination, H.M. initially made many mistakes, but after several days of training his performance was error-free and comparable to that of healthy subjects. Nevertheless, he did not consciously remember having performed the task.
Figure 65–3 The amnesic patient H.M. could learn skilled movements. He was taught to trace between two outlines of a star while viewing his hand in a mirror. The graph plots the number of times, during each attempt, that he strayed outside the outlines as he drew the star. As with healthy subjects, H.M. improved considerably with repeated attempts despite the fact that he had no recollection of having performed the task before. (Reproduced, with permission, from Blakemore 1977.)
Later work by Squire and others made it clear that the long-term memory capabilities of H.M. and other amnesic patients are not limited to motor skills. These patients retain simple reflexive learning, including habituation, sensitization, classical conditioning, and operant conditioning (to be discussed later in this chapter). Furthermore, they are able to improve their performance on certain perceptual and conceptual tasks. For example, they do well with a form of memory called priming, in which perception of a word or object is improved by prior exposure. Thus, when shown only the first few letters of previously studied words, a subject with amnesia is able to generate the same number of words as normal subjects, even though the amnesic patient has no conscious memory of having recently studied the words (Figure 65–4).
Figure 65–4 Amnesic and normal control subjects were tested on recall of words under two conditions. First they were presented with common words and then asked to recall the words (free recall). Amnesic patients did not do well on this test. However, when subjects were given the first three letters of a word and instructed simply to form the first word that came to mind (completion), the amnesic subjects performed as well as normal subjects. The baseline guessing rate in the word completion condition was 9%. (Reproduced, with permission, from Squire 1987.)
Is this distinction between forms of long-term memory in amnesic patients a fundamental difference in normal memory function? To address this question the cognitive psychologists Peter Graf and Daniel Schacter examined healthy subjects and found two types of long-term memory that differed in whether conscious awareness was required for the recall.
One type is an unconscious form of memory that is evident in the performance of a task and is known as implicit memory (also referred to as nondeclarative or procedural memory). Implicit memory is typically manifested in an automatic manner, with little conscious processing on the part of the subject. Different forms give rise to priming, skill learning, habit memory, and conditioning (Figure 65–5).
Figure 65–5 Long-term memory is either explicit (conscious) or implicit (subconscious).
The other type is the deliberate or conscious retrieval of previous experiences as well as conscious recall of factual knowledge about people, places, and things. This type is known as explicit memory (or declarative memory). Explicit memory is highly flexible; multiple pieces of information can be associated under different circumstances. Implicit memory, however, is tightly connected to the original conditions under which the learning occurred.
Explicit Memory Has Episodic and Semantic Forms
The Canadian psychologist Endel Tulving first developed the idea that explicit memory can be further classified into episodic memory (the memory of personal experiences or autobiographical memory) and semantic memory (memory for facts). Episodic memory is used to recall that we saw the first flowers of spring yesterday or that we heard Beethoven’s “Moonlight Sonata” several months ago. Semantic memory is used to learn the meanings of new words or concepts. The medial temporal lobe plays a critical role in both episodic and semantic memory, as is evident in patients like H.M., who have difficulties in forming and retaining new conscious memories of their personal experiences or the meanings of new concepts.
We have learned two additional important things about explicit memory. First, the brain does not have a single long-term store of explicit memories. Instead the storage of any item of knowledge is widely distributed among many brain regions and can be accessed independently (by visual, verbal, or other sensory clues). Second, explicit memory is mediated by at least four related but distinct types of processing: encoding, storage, consolidation, and retrieval.
Explicit Memory Processing Involves at Least Four Distinct Operations
Encoding is the process by which new information is attended and linked to existing information in memory. The extent of this process is critically important for determining how well the learned material will be remembered. For a memory to persist and be well remembered, the incoming information must be encoded thoroughly, what the psychologists Fergus Craik and Robert Lockhart called “deep” encoding. This is accomplished by attending to the information and associating it with knowledge that is already well established in memory. Memory encoding also is stronger when one is well motivated to remember.
Storage refers to the neural mechanisms and sites by which memory is retained over time. One of the remarkable features about long-term storage is that it seems to have an almost unlimited capacity; there is no known limit to the amount of information in long-term storage. In contrast, working memory storage is very limited; psychologists believe that human working memory can hold only a few pieces of information at any one time.
Consolidation is the process that makes the temporarily stored and still labile information more stable. As we shall learn in the next two chapters, consolidation involves expression of genes and protein synthesis that give rise to structural changes at synapses.
Finally, retrieval is the process by which stored information is recalled. It involves bringing back to mind different kinds of information that are stored in different sites. Retrieval of memory is much like perception; it is a constructive process and therefore subject to distortion much as perception is subject to illusions (Box 65–1).
Retrieval of information is most effective when a retrieval cue reminds individuals of how they initially encoded an experience. For example, in a classic behavioral experiment Craig Barclay and colleagues asked some subjects to encode sentences such as “The man lifted the piano.” On a later test, “something heavy” was a more effective cue for recalling piano than “something with a nice sound.” Other subjects, however, encoded the sentence “The man tuned the piano.” For them, “something with a nice sound” was a more effective retrieval cue for piano than “something heavy.” Retrieval, particularly of explicit memories, also is partially dependent on working memory.
Episodic Knowledge Depends on Interaction Between the Medial Temporal Lobe and Association Cortices
Although studies of amnesic patients during the past few decades have refined our understanding of various types of memory, medial temporal lobe damage affects all four operations of memory—encoding, storage, consolidation, and retrieval—and thus it is often difficult to discern how the medial temporal lobe contributes to each. Positron emission tomography (PET) and functional magnetic resonance imaging (fMRI) allow us to scan the healthy brain in the process of building new memories or retrieving existing memories, and thus to identify specific regions that are active during different processes.
Functional MRI scans show that activity in the medial temporal lobe is greater when subjects engage in deep encoding (eg, attending to the meaning of information by judging whether a word is concrete or abstract) than when they engage in shallow encoding (eg, judging whether a word is presented in upper or lower case letters). Activity in parts of the left prefrontal cortex is also enhanced during deep encoding, suggesting that frontal lobe and medial temporal lobe processing contribute to encoding episodic memory.
How accurate is explicit memory? This question was explored by the psychologist Frederic Bartlett in a series of studies in the 1930s in which the subjects were asked to read stories and then retell them. The recalled stories were shorter and more coherent than the original stories, reflecting reconstruction and condensation of the original.
The subjects were unaware that they were editing the original stories and often felt more certain about the edited parts than about the unedited parts of the retold stories. They were not confabulating; they were merely interpreting the original material so that it made sense on recall.
Observations such as these lead us to believe that explicit memory, at least episodic (autobiographical) memory, is a constructive process like sensory perception. In fact, explicit memory is a product of the perceptual process. Sensory perception is not a passive recording of the external world but a process in which sensory signals produce information that is shaped by the way in which afferent pathways process those signals.
It is also constructive in the sense that individuals perceive the environment from the standpoint of a specific point in space as well as a specific point in their own history. As described in Chapter 27, optical illusions nicely illustrate the active role of perceptual processes in arriving at our personal knowledge of our surroundings.
Likewise, once information is stored, recall is not an exact copy of the information stored. Past experiences are used in the present as cues that help the brain reconstruct a past event. During recall we use a variety of cognitive strategies, including comparison, inference, shrewd guessing, and supposition, to generate a memory that not only seems coherent to us but is also consistent with other memories and with our “memory of the memory.”
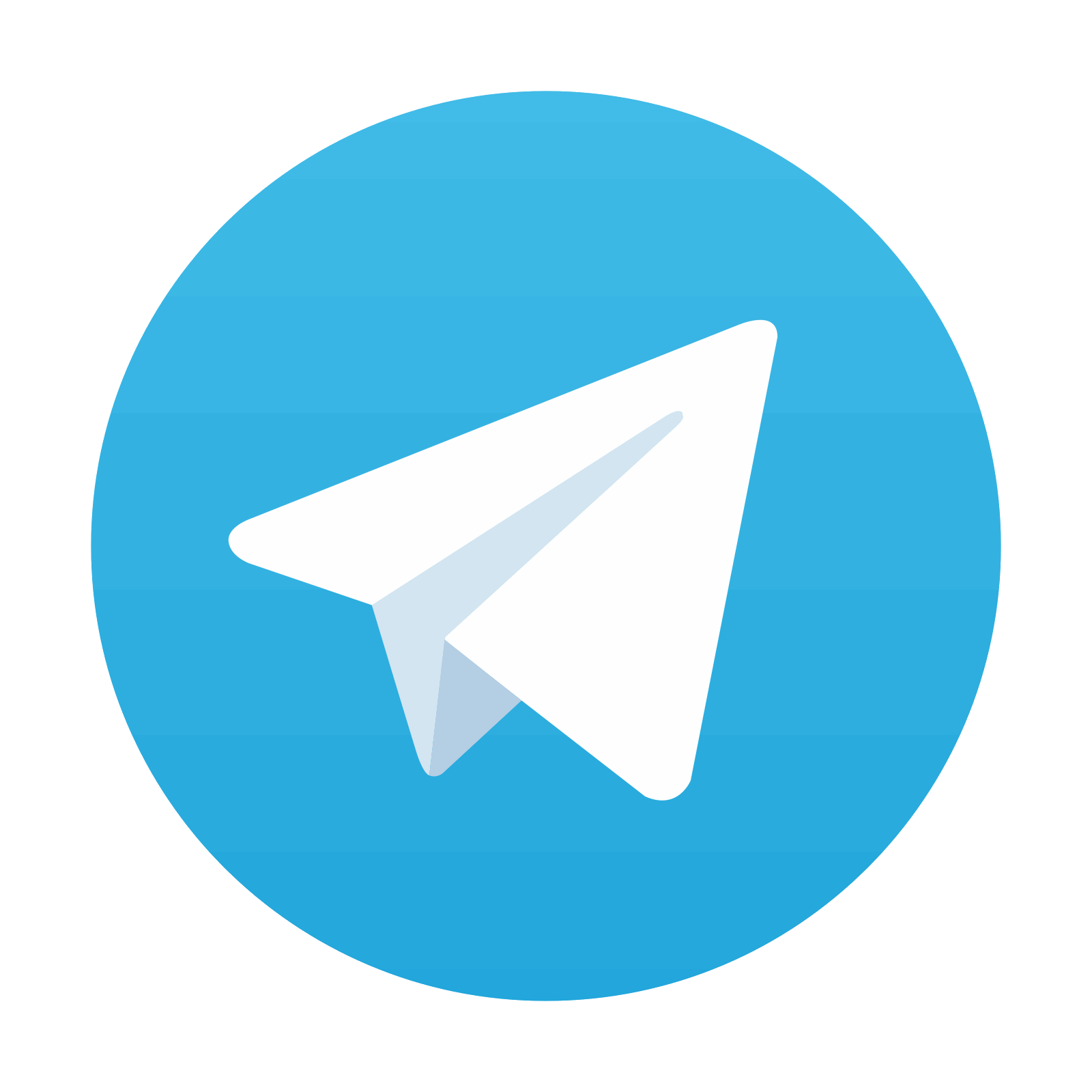
Stay updated, free articles. Join our Telegram channel

Full access? Get Clinical Tree
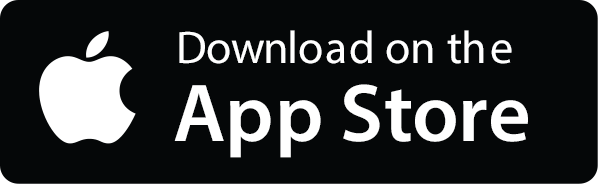
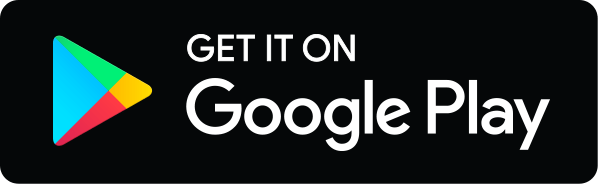