The Organization of Cognition
Functionally Related Areas of Cortex Lie Close Together
Sensory Information Is Processed in the Cortex in Serial Pathways
Parallel Pathways in Each Sensory Modality Lead to Dorsal and Ventral Association Areas
The Dorsal Visual Pathway Carries Spatial Information and Leads to Parietal Association Cortex
The Ventral Visual Pathway Processes Information About Form and Leads to Temporal Association Cortex
Goal-Directed Motor Behavior Is Controlled in the Frontal Lobe
Pre-Frontal Cortex Is Important for the Executive Control of Behavior
Dorsolateral Pre-Frontal Cortex Contributes to Cognitive Control of Behavior
Orbital-Ventromedial Pre-Frontal Cortex Contributes to Emotional Control of Behavior
Limbic Association Cortex Is a Gateway to the Hippocampal Memory System
IN THE PREVIOUS CHAPTER WE EXAMINED how the activity of single nerve cells can be related to the internal representations required for simple cognitive tasks. In this chapter we survey the anatomical and physiological organization of the cortex through which the activity of populations of neurons mediate complex aspects of cognition. For this purpose we draw on the insights that have emerged from studies of the neural mechanisms of cognition in monkeys and studies of cognitive impairment resulting from brain injury in humans. In the following chapter we extend this analysis to the premotor cortex and the control of voluntary movement. In Chapter 20 we discuss how neuro-imaging studies of cognition in humans are consistent with the findings from these experimental and clinical studies.
Cognitive functions are mediated by specialized areas of neocortex distributed across the cerebral hemisphere in an orderly arrangement. This was already well established in 1962 when Alexander Luria published his landmark Higher Cortical Functions in Man. Neurologists of Luria’s generation knew that lesions at neighboring sites on the cortical surface tend to produce related symptoms. For example, lesions of the occipital cortex give rise to lower-order visual deficits (cortical blindness), whereas lesions of adjacent temporal cortex result in higher-order visual deficits (object agnosia). Similarly, lesions in the posterior sector of the frontal lobe give rise to lower-order motor deficits (weakness and paralysis), whereas more anterior lesions give rise to higher-order deficits of executive control (the pre-frontal syndrome). How can these observations be explained?
Luria proposed that sensory and motor cortex comprise multiple specialized subareas that are connected hierarchically. A primary sensory area lies next to a secondary sensory area that in turn borders a tertiary area. These areas have progressively more complex functions, culminating in the integration of multiple sensory modalities in the tertiary zones. Areas in the frontal lobe concerned with motor behavior are similarly organized. The primary motor cortex lies next to a secondary motor area (the premotor cortex) that in turn borders a tertiary area, the pre-frontal cortex, concerned with the executive control of behavior. In Luria’s scheme sensory information flows into the central nervous system through a series of synaptic relays from primary to secondary to tertiary sensory areas, whereas motor commands flow from tertiary to secondary to primary motor areas. The tertiary areas at the peak of these sensory and motor hierarchies interact and are the seats of cognitive function.
More than 45 years after publication of Luria’s book these general principles are still accepted. However, our understanding of the neural systems of cognition is far richer than that of Luria and his contemporaries, in large part because of newer methodologies.
Functionally Related Areas of Cortex Lie Close Together
The cortex of each cerebral hemisphere is a continuous sheet of gray matter. At the coarsest level, it consists of five lobes as illustrated in Figure 18-1. Within each of these lobes the cortex is further subdivided into anatomically and functionally defined areas.
Figure 18-1 The cerebral cortex of the macaque monkey. Lateral and medial views show the location of five cortical lobes. The labeled areas on the unfolded hemisphere (lower panel) are those defined in physiological and anatomical studies. (Adapted, with permission, from Van Essen et al. 2001.)
The anatomical labels are as follows. The numbered areas are Brodmann’s areas; AB, auditory belt; AIP, anterior intraparietal area; CA1, CA3, cornu ammonis fields of hippocampus; Core, primary auditory cortex; DP, dorsal prelunate area; ER, entorhinal cortex; FEF, frontal eye field; FST, floor of superior temporal sulcus; G, gustatory cortex; Id, Ig, insular cortex, dysgranular and granular divisions; IT, inferotemporal cortex; LIP, lateral intraparietal area; MDP, medial dorsal parietal area; M1, primary motor cortex; MIP, medial intraparietal area; MSTd, MSTI, medial superior temporal sulcus, dorsal and lateral divisions; MT, middle temporal area; PA, postauditory area; PAC, periamygdaloid cortex; PaS, parasubiculum; PB, auditory parabelt cortex; PIP, posterior intraparietal; Pir, piriform cortex; PM, premotor cortex; PO, parieto-occipital area; Pro, orbital proisocortex; PrS, presubiculum; Ri, retroinsular area; S, subiculum; SEF, supplementary eye field; S-ll, secondary somatosensory area; SMA, supplementary motor and adjacent cingulate motor areas; STGc, STGr, superior temporal gyrus, caudal and rostral divisions of auditory cortex; STPa, STPp, superior temporal polysensory area, anterior and posterior divisions; TEO, temporo-occipital area; TF, TH, parahippocampal areas; V1, V2, V3, V3a, V4, V4t, visual areas; VIP, ventral intraparietal area.
Functional areas are distinguished by cellular structure, connectivity, and the physiological response properties of neurons. Identification of the functions of a cortical area requires characterizing the behavioral conditions under which its neurons are electrically active (by means of single-neuron recording) and determining its anatomical connections with other areas (by means of neuroanatomical tracers).
These invasive methods cannot be used in humans except under rare circumstances in which there is some clinical benefit. Consequently the major advances in identifying the function of anatomically or physiologically discrete areas of cortex have come from studies of animals, in particular the rhesus monkey, a species of the macaque (Macaca), an Old World monkey. Old World monkeys are our closest living relatives aside from the apes; like us, they are able to carry out demanding tasks that require well-developed cognitive abilities such as attention, memory, and pattern discrimination.
In the macaque a region of cortex is defined as functionally distinct if neurons within it have similar functional properties (such as responding to similar types of visual stimulation) and common connections (such as receiving the same input from primary visual cortex). In addition, to qualify as an area of lower-order sensory or motor cortex the neurons in that area must be organized into a single, coherent neural map of the sensory or motor periphery. The number of functionally distinct areas identified by these criteria (Figure 18-1) is much greater than the number of areas defined cytoarchitecturally because an area with anatomically similar cells may contain several functionally and connectionally distinct areas.
A few simple precepts govern the organization of functional areas in the macaque cerebral cortex: (1) all areas fall into a few major functional categories; (2) areas in a given category occupy a discrete, continuous portion of the cortical sheet; and (3) areas that are functionally related occupy neighboring sites (Figure 18-2).
Figure 18-2 The cerebral cortex is divided into discrete functional categories. Cortical areas in each functional category occupy a continuous physical region of the cortical sheet, shown here in a schematic version of the unfolded hemisphere in Figure 18-1. The table indicatesthefunctionally distinct areas within each category. Primary sensory areas send their outputs to multiple secondary sensory areas, which in turn provide inputs to tertiary (higher-order) areas. Multimodal areas are tertiary areas with significant input from more than one sensory system.
Sensory Information Is Processed in the Cortex in Serial Pathways
In analyzing how the areas of the cerebral cortex act together to produce behavior it is useful to ask, as in the study of social organizations, who talks to whom? Cortical areas communicate with each other by means of bundles of axons traveling together in identifiable tracts. As a result of neuro-anatomical tracing studies in the monkey, the neural tracts (or projections) running from area to area are now well understood. A dye injected into one population of neurons is carried by axonal transport to distant clusters of neurons that can be identified because they are labeled with the dye (see Box 4-2).
These tracing studies have confirmed Luria’s idea that the sensory areas of cortex are organized hierarchically. Within each sensory system (visual, auditory, etc.) signals from the periphery arrive at a primary sensory area, such as the primary visual cortex (VI), primary auditory cortex (Al), or primary somatosensory cortex (S-I).
Primary sensory areas possess four properties characteristic of their role in the early stages of information processing.
1. Their input is from thalamic sensory relay nuclei. (The thalamus is the main subcortical source of input to all areas of cortex, but only some thalamic nuclei relay sensory signals).
2. The neurons in a primary sensory area have small receptive fields—the region on the receptor surface that must be stimulated in order for the neuron to fire—and are arranged to form a precise somatotopic map of the sensory receptor surface (retina, cochlea, or skin).
3. Injury to a part of the map causes a simple sensory loss confined to the corresponding part of the contralateral sensory receptor surface.
4. Connections to other cortical areas are limited, confined almost entirely to nearby areas that process information in the same modality.
Higher-order sensory areas have a different set of properties that are important to their role in the later stages of information processing.
1. They receive little input from the sensory relay nuclei in the thalamus; instead, their input arises from other thalamic nuclei and lower-order areas of sensory cortex.
2. Their neurons have large receptive fields and are organized into imprecise maps of the array of receptors in the periphery.
3. Injury results in abnormalities of perception and of related cognitive functions but does not impair the ability to detect sensory stimuli.
4. They are connected not only to nearby unimodal sensory areas but also to distant areas in the frontal and limbic lobes.
Thus, sensory information is processed serially, with each area in the chain carrying out certain computations and conveying the results to the next area. For example, in the ventral pathway of the visual system, which is concerned with processing information about form, the pathway begins with neurons that respond to detailed features of a visual stimulus and proceeds to neurons that encode the overall form. Receptive fields of individual neurons in the primary visual cortex (V1) span approximately 1 degree of visual angle; those of neurons in V4 (a mid-order area) span approximately 10 degrees; and those in the inferotemporal cortex (a higher-order area) span up to 100 degrees. Thus an individual neuron in V1 could be sensitive to a small detail in a face, such as an eyebrow aligned in a certain direction within its small receptive field, whereas a neuron in the inferotemporal cortex can respond to an entire face.
However, sensory pathways are not exclusively serial; in each functional pathway higher-order areas project back to the lower-order areas from which they receive input. In this way neurons in higher-order areas, sensitive to the global pattern of sensory input, can modulate the activity of neurons in lower-order areas that are sensitive to local detail. For example, top-down signals originating in the inferotemporal cortex might help neurons in V1 to resolve a detail in a part of the face.
The hierarchical chain of sensory processing leads to areas with functions so complex that they cannot be described as simply sensory. In the late 19th century Santiago Ramón y Cajal proposed that areas with sensory functions were fundamentally distinct from those with cognitive functions, calling the latter the association cortex. This term is rooted in the idea that cognition depends upon our learning which of the myriad stimuli impinging on our senses are associated with one another. Modern neuroscientists apply the term association cortex to regions of cortex where injury causes cognitive deficits that cannot be explained by impairment of sensory or motor function alone.
Large regions of association cortex are contained within each of the four lobes and contribute to cognition in distinctive ways.
The parietal association cortex is critical for sensory guidance of motor behavior and spatial awareness.
The temporal association cortex is important for recognition of sensory stimuli and for storage of semantic (factual) knowledge.
The frontal association cortex plays a key role in organizing behavior and in working memory.
The limbic association cortex serves complex functions related to emotion and episodic (autobiographical) memory.
Association areas have much more extensive input and output connections than do lower-order sensory and motor areas. Some association areas have a variety of visual, auditory, somatosensory, and motor connections that permit them to integrate sensory modalities or to use sensory information to guide motor behavior (see Chapter 19). In addition, all association areas are interconnected by a dense network of pathways within and between the parietal, temporal, frontal, and limbic lobes.
Parallel Pathways in Each Sensory Modality Lead to Dorsal and Ventral Association Areas
In addition to serial processing another principle of cortical organization is that the same information is processed differently in parallel pathways. In the visual system for example, two major parallel pathways terminate in different higher-order areas of cortex. The dorsal stream processes spatial information (position, motion, speed) and projects to parietal association cortex. The ventral stream processes information about form (color, shape, texture) and projects to temporal association cortex.
Dorsal and ventral pathways exist in other sensory systems as well (Figure 18-3). In the auditory and somatosensory systems dorsal pathways serve motor and spatial functions, whereas ventral pathways serve recognition functions. The dorsal-ventral division extends into frontal association cortex.
Figure 18-3 The dorsal and ventral systems of the cerebral cortex. Lower-order sensory areas send their output in parallel to the parietal (dorsal stream) and temporal (ventral stream) association cortices, which in turn send their output to the frontal association cortex. The parietal cortex projects primarily to dorsal areas of frontal cortex, areas that serve motor and executive control functions for which spatial information is important. The temporal cortex projects primarily to ventral regions of frontal cortex, including the orbital pre-frontal cortex, areas that mediate emotional responses to things in the environment. Emotional significance can be assigned to an object only after the object has been recognized, an ability that depends on areas of the temporal lobe.
The Dorsal Visual Pathway Carries Spatial Information and Leads to Parietal Association Cortex
The parietal cortex plays a key role in the visual guidance of motor behavior and in spatial perception and cognition (and understanding where objects are relative to each other). These two functions are related because visuomotor control requires processing spatial information. Reaching for your coffee cup while you read the newspaper requires that the brain take into account where the image of the cup is on your retina and where your eyes are pointing so as to determine where the cup is relative to your hand.
The parietal cortex is ideally suited for such computations because it is connected to visual, somatosensory, and motor areas of cortex. Parietal cortex may have initially developed the capacity to represent where things are relative to the body to guide actions such as grasping, and then developed the ability to represent where things are relative to each other without reference to the body.
As we have learned in Chapter 17, injury to the parietal cortex in humans results in a wide range of behavioral impairments, which can be classified into two broad categories. In the first category are impairments of body awareness, motor control, and visual guidance of motor behavior. These deficits result from damage to dorsal parts of the parietal cortex close to and connected with the somatosensory cortex. In the second category are impairments of spatial perception and cognition. These deficits result from damage to ventral parts of parietal cortex close to and connected with the visual cortex. Thus the parietal cortex can be thought of as having two subdivisions: a dorsal component serving primarily motor functions and a ventral component serving primarily spatial functions.
Specific impairments in the first category include asomatognosia, a disorder of body awareness in which patients deny the existence of the arm or leg contralateral to the lesion or refuse to acknowledge that it belongs to them even when they can see it. Another is ideomotor apraxia, which arises from damage to the dominant hemisphere; patients are unable to execute certain movements such as waving goodbye, either on command or by imitation, although they may spontaneously make the same movement under circumstances that evoke it habitually. A third deficit in this category, optic ataxia, results from damage to the dor-somedial parietal cortex. Patients with this deficit have difficulty reaching for an object in the peripheral visual field (as when reaching for a coffee cup while reading the newspaper). The hand may go to the wrong location, or it may be misoriented when attempting to grasp the object (Figure 18-4). Patients can, however, perform a reaching task that does not depend on vision, for example touching one’s knee in the dark, and can report the locations of visible objects correctly. This collection of symptoms cannot be explained by a purely motor or purely visual mechanism but instead reflects difficulty in coordinating visual input and motor output.
Figure 18-4 Patients with damage to the dorsomedial parietal cortex have difficulty with visually guided grasping and reaching (optic ataxia). When required to grasp an object, patients fail to shape their hand appropriately. When required to place their fingers through a slot in a plate, they reach to the wrong location and fail to orient the hand correctly. (Adapted, with permission, from Jeannerod 1986 [left panels]; and Perenin and Vighetto 1988 [right panels].)
Specific impairments in the second category include hemispatial neglect. Patients with this defect are profoundly inattentive to events in the half of space opposite the injured side (see Chapter 17). Another is constructional apraxia, an inability to appreciate the structure and arrangement of things by looking at them. Patients suffering from constructional apraxia have difficulty arranging a set of tiles or matchsticks according to a model placed in plain view. They may also be deficient in tests of writing and drawing because these require putting marks on a page in a precise arrangement (Figure 18-5).
Figure 18-5 Disorders of copying and drawing result from damage to the parietal cortex. Drawings of complex figures are grossly inaccurate whether drawn from a model or from memory. The problem arises from an inability to perceive the spatial relations of the parts of an object. (Reproduced, with permission, from Critchley 1953; and Trojano and Grossi 1998.)
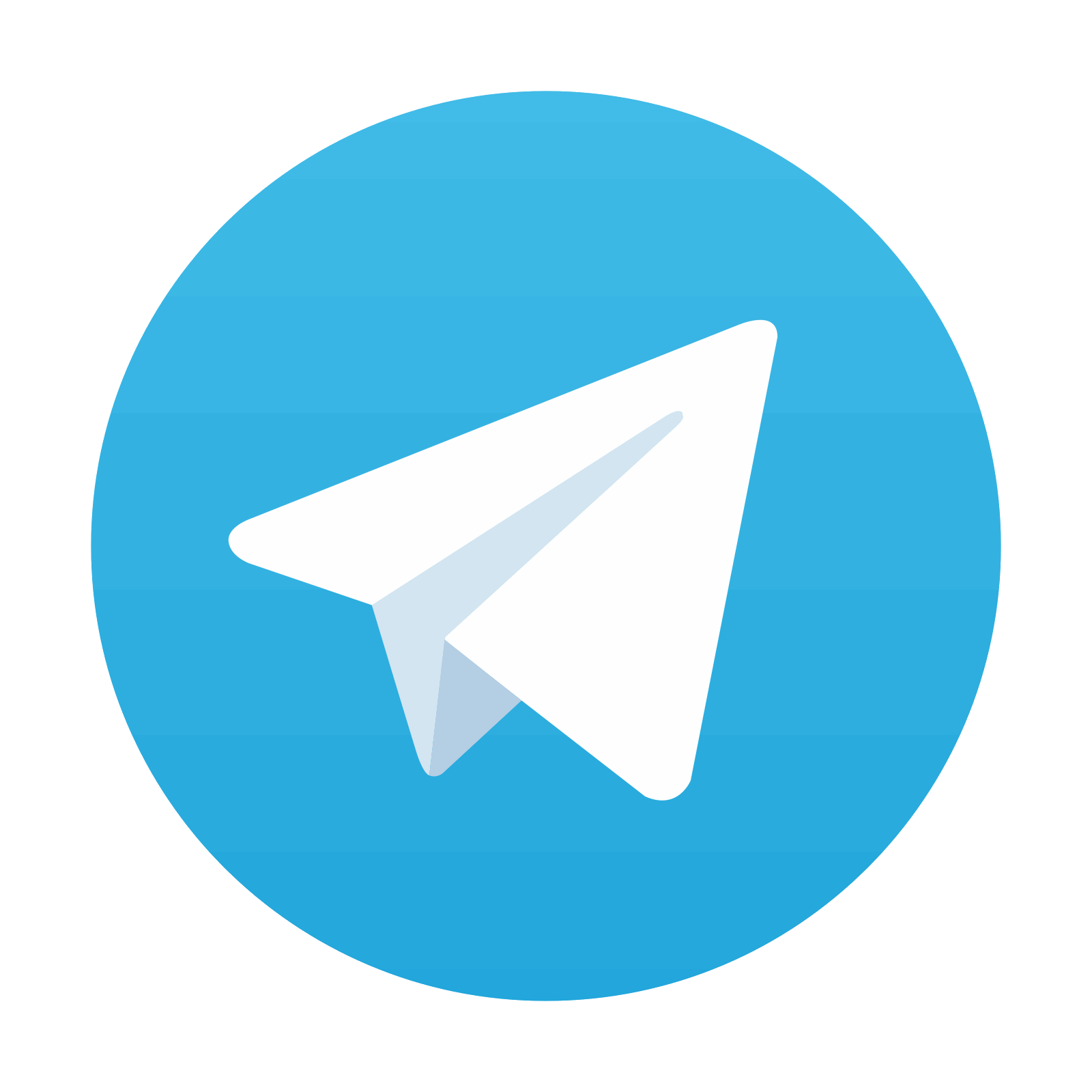
Stay updated, free articles. Join our Telegram channel

Full access? Get Clinical Tree
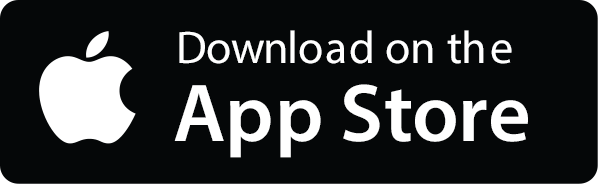
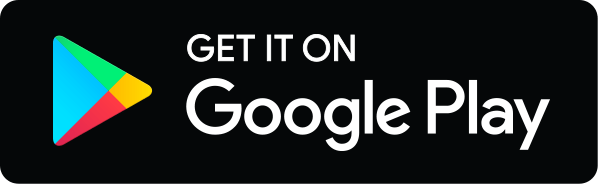