Fig. 3.1
A framework for understanding the impact of lithium treatment for bipolar disorder. Lithium inhibits (red arrows) and facilitates (green arrows) a number of neurotransmitter and cellular signal transduction mechanisms involved in degeneration and neuroprotection. In terms of key mechanisms, lithium inhibits neuroprogression through the excitotoxic damage-GSK-3β-mTOR and excitotoxic damage-GSK-3β-CREB-neurotrophin encoding pathways involved in neural degeneration and neuroprotection, where excitotoxic damage has occurred through oxidative and nitrosative damage pathways. These mechanisms are framed within the wider context of the therapeutic effects of lithium on the neurocircuitry dysfunction and mood dysregulation and neurocognitive dysfunction characteristic of bipolar disorder. This simplified framework is based on a previous review (see Malhi et al. 2013; 2016a)
3.2 Neuroprogression, Bipolar Disorder and Lithium Treatment
Proponents of neuroprogression perspectives (e.g. Frank et al. 2015) view bipolar disorder as an illness that has deteriorating course, with progressive changes across multiple systems. Lithium has multiple potential actions that counteract neuroprogression and lead to therapeutic benefits. The process of neuroprogression and the effects of lithium treatment on this process can be examined at multiple levels of ‘magnification’, ranging from microscopic changes in cellular functions to macroscopic changes that manifest clinically (Fries et al. 2012; Malhi et al. 2013; see Fig. 3.2). Many interacting neurotransmitter and cellular signal transduction processes underlie the pathophysiology of bipolar disorder and its responses to therapy, but their influences are distal from bipolar disorder’s characteristic mood dysregulation, neurocognitive dysfunction and clinical presentation. Therefore, it is important to consider intermediate levels, such as changes to neurocircuitry, that may occur with neuroprogression of the illness and in response to therapy (Nock et al. 2009; Berk et al. 2011b; Fries et al. 2012; Malhi et al. 2013). It is likely that through a series of interconnected pathways involving changes in neurotransmission and cellular and neurocircuitry mechanisms, lithium exerts its therapeutic effects as a mood stabilizer and serves as a prophylactic treatment (Berk 2009; Berk et al. 2011b; Fries et al. 2012; Malhi et al. 2013).


Fig. 3.2
Neuroprogression and the effects of lithium treatment in bipolar disorder can be understood from multiple levels of understanding, from cellular and intracellular changes to clinical presentation. To understand how neuroprogression and therapeutic changes at the cellular level result in changes in clinical presentation, the more intermediate changes at the neurotransmission, neurocircuitry and mood dysregulation and neurocognitive dysfunction levels are considered. It is important to consider these intermediate changes as they are more proximal mechanisms to both cellular and intracellular changes and clinical presentation (Malhi et al. 2013)
Clinically, treatment resistance in bipolar disorder is correlated to the number of episodes the individual has experienced—in other words the total illness burden and therefore lithium treatment response is likely to be a function of the level of underlying neuroprogression that has already occurred (Berk et al. 2011a; Malhi et al. 2011b, 2012). Interestingly, lithium attenuates neural degradation and augments neuroprotection pathways by directly acting on deficits in neurotransmission and cellular signal transduction processes (Alda 2015), and these effects ultimately lead to changes in the structure and function of neurocircuitry implicated in mood dysregulation and neurocognitive dysfunction in bipolar disorder (Goodwin and Malhi 2007; Tsaltas et al. 2009; Malhi et al. 2011a, 2013; see Fig. 3.1).
Key neurotransmitters implicated in neuroprogressive changes in bipolar disorder include dopamine, glutamate and γ-aminobutyric acid (GABA; Berk 2009; Berk et al. 2011b; Malhi et al. 2013). Dopamine is an excitatory neurotransmitter thought to be involved in reward systems and cyclical mood dysregulation (Post et al. 1980; Staunton et al. 1982; Jacobs and Silverstone 1986; Berk et al. 2007; Cousins et al. 2010; Malhi et al. 2012). Glutamate is also an excitatory neurotransmitter but when in excess can produce toxic effects, and notably brain concentrations of glutamate have been found to be elevated during mania (Michael et al. 2003; Chuang and Manji 2007; Marmol 2008; Mauer et al. 2014). In contrast, GABA is an inhibitory neurotransmitter that acts to balance and regulate both dopamine and glutamate neurotransmission, and hence it is thought to contribute to mood stabilization (Brambilla et al. 2003; Kato 2008; Ng et al. 2009; Berk 2009; Ghasemi and Dehpour 2011; Berk et al. 2011b). In bipolar disorder, over-excitation of dopaminergic and glutamatergic pathways, along with diminished inhibition from underactive GABAergic pathways, may be the cause of oxidative and nitrosative damage in postsynaptic cells (Berk et al. 2011b). Countering these actions in bipolar disorder, lithium inhibits excitotoxic degradation and facilitates the availability of neuroprotective factors (Chen and Chuang 1999; Marmol 2008; Chiu and Chuang 2010; Malhi et al. 2013), stabilizing perhaps not only acute episodes of illness but also limiting further neuroprogression of the illness. The process of neuroprogression that leads to degenerative cellular changes and decreases in neuroprotective factors and the opposing actions of lithium are discussed in greater detail in the following sections.
3.3 Neurotransmission
Bipolar disorder is associated with changes in a wide range of neurotransmitters that eventuate in neural dysfunction. Lithium acts both pre- and postsynaptically to modulate neurotransmission (see Fig. 3.1), and though multiple interactions between neurotransmission pathways are implicated, the modulation and regulation of dopamine (Post et al. 1980; Staunton et al. 1982; Jacobs and Silverstone 1986; Berk et al. 2007; Cousins et al. 2010), glutamate (Dixon and Hokin 1998; Berk 1999; Brunello and Tascedda 2003; Ongür et al. 2008; Ghasemi and Dehpour 2011) and GABA (Ahluwalia et al. 1981; Vargas et al. 1998; Brambilla et al. 2003; Brunello and Tascedda 2003; Kato 2008; Ng et al. 2009; Ghasemi and Dehpour 2011) are thought to be key to the therapeutic effects of lithium (Malhi et al. 2013).
3.3.1 Dopamine
Dopamine agonists and antagonists produce robust manic and anti-manic behavioural effects (Berk et al. 2011b). An increase in dopaminergic activity in bipolar disorder produces oxidative damage to postsynaptic cells, because this increases the concentration of potentially damaging dopamine metabolites (Berk 2009; Berk et al. 2011b; Malhi et al. 2013). Increased dopamine requires increased metabolism via monoamine oxidase with the resulting production of hydrogen peroxide (H2O2) and dihydroxyphenylacetic acid (Maker et al. 1981; Berman and Hastings 1999). However, the metabolism of dopamine can also occur through non-enzymatic hydroxylation with iron (Fe2+) and water (H2O2) leading to the formation of free radicals, which in turn induces oxidation of DNA, proteins and lipids (Graham et al. 1978). In animal models, lithium decreases extracellular dopamine, which in turn decreases reactivity to harmful stimuli (Gambarana et al. 1999; Ichikawa et al. 2005). When lithium treatment is withdrawn, dopamine levels increase and remain elevated for several days (Ferrie et al. 2005). Thus, regulation of dopamine may explain both treatment response and relapse in patients as lithium levels move into and out of the therapeutic window (Malhi et al. 2011b).
The action of lithium on dopaminergic pathways is not only evident in normalizing presynaptic neurotransmission but also affects postsynaptic actions. G-protein-coupled dopamine receptors stimulate cellular signal transduction mechanisms (discussed in the next section), which initiate a cascade of processes that modulate dopamine neurotransmission. Chronic lithium administration alters the function of G-protein active and inactive subunits (Manji and Lenox 2000a), modulating the stimulation of the transduction mechanisms. The aforementioned oxidation pathway activates the production of GSK-3β (Stokes et al. 1999; Obata 2002; Grima et al. 2003), which is a component of the cell survival-promoting signalling pathway (discussed in the next section; Gould et al. 2006). Along with dopaminergic-mediated oxidative damage leading to compromised anti-oxidation, glutamatergic-mediated nitrosative damage induces membrane damage, protein aggregation and apoptosis initiation (Andreazza et al. 2009; Ghasemi and Dehpour 2011).
3.3.2 Glutamate
In bipolar disorder, increased glutamatergic neurotransmission in bipolar disorder increases cellular damage via the activation of the N-methyl-D-aspartic acid (NMDA) receptor (Berk et al. 2000; Plein and Berk 2000; Zuo et al. 2007; Zou et al. 2010; Diazgranados et al. 2010), which facilities calcium (Ca2+) influx (Nonaka et al. 1998; Bown et al. 2003) and consequent nitric oxide (NO) production (another highly reactive free radical). This leads to nitrosative damage to DNA, proteins and lipids (Andreazza et al. 2009; Ghasemi and Dehpour 2011). Glutamate is usually cleared by astrocytes, where it is converted to glutamine (Choi et al. 1987; Berk et al. 2000, 2011b; Kato 2007), but its clearance may be reduced by the effects of inflammatory cytokines on astrocytes (Zou et al. 2010). Bipolar disorder patients have elevated intracellular Ca2+ mediated in part by increase glutamate receptor activity. Raised levels of glutamate have been associated with neuronal death and are thus also implicated in the pathogenesis of neurodegenerative diseases (Zou et al. 2010) including Alzheimer’s disease (Robinson 2000) and Parkinson’s disease (Zipp et al. 1998; Zoghbi and Orr 2000; Orr et al. 2002).
Lithium is known to act on glutamatergic pathways and this may partly explain its long-term mood-stabilizing properties (Dixon and Hokin 1998; Brunello and Tascedda 2003; Ghasemi and Dehpour 2011). Specifically, lithium selectively competes with magnesium at binding sites on NMDA glutamate receptors (Tsapakis and Travis 2002), leading to acute stimulation, which in turn increases the availability of glutamate in the postsynaptic neuron (Hokin et al. 1996). However, with chronic lithium administration, glutamate transmission is restored by directly downregulating the NMDA receptor and increasing glutamate reuptake. Given that lithium downregulates glutamate neurotransmission at the NMDA receptor, it is able to ameliorate the damaging excitotoxic effects of Ca2+ influx via the aforementioned NO-nitrosative pathway (Nonaka et al. 1998; Bown et al. 2003). Interestingly, other NMDA antagonists also have rapid effects on mood (Diazgranados et al. 2010).
3.3.3 GABA
The final neurotransmitter pathway that plays a significant role in the neuroprogression of bipolar disorder is the GABAergic pathway. Patients with bipolar disorder have decreased GABAergic neurotransmission, decreasing inhibitive signalling (Lenox et al. 1998; Shiah and Yatham 1998; Brambilla et al. 2003; Kato 2008; Ng et al. 2009). Low GABA levels result in an increase in excitatory dopaminergic and glutamatergic neurotransmission that, as previously discussed, can lead to excitotoxicity (Rajkowska 2002). This again contributes to activity within the oxidative and nitrosative pathways that results in apoptosis and cell loss (Rajkowska 2002).
Lithium modifies GABAergic neurotransmission, and increases in GABAergic transmission occur in relation to lithium’s long-term mood-stabilizing effects. Specifically, lithium increases the level of GABA in the plasma and cerebrospinal fluid (Ahluwalia et al. 1981; Vargas et al. 1998; Brunello and Tascedda 2003). Given that increasing GABA levels decreases excitatory neurotransmission, lithium’s effects on GABA also promote the release of neuroprotective proteins (Chuang et al. 2002), and an increase in GABA, in response to lithium, reduces the level of glutamate, which further downregulates NMDA receptor activity (Ghasemi and Dehpour 2011). Hence, long-term mood stabilization effects of lithium stem from a combination of its effects on GABA and the excitatory dopaminergic and glutamatergic neurotransmitter systems.
3.4 Cellular Signal Transduction Mechanisms
Cellular signal transduction mechanisms are involved in the process of neural degradation and neuroprotection in bipolar disorder (see Fig. 3.1). However, these processes themselves are also adversely impacted by the illness. The actions of lithium on several interacting cellular signal transduction mechanisms are thought to contribute to its therapeutic effects (Malhi et al. 2013; Alda 2015).
Centred on GSK-3β, there are some key therapeutic mechanisms involving neural degradation and neuroprotection processes that curb neuroprogression. GSK-3β is an enzyme that regulates glycogen synthesis and has direct involvement in the genetic transcription of neuroprotective factors (Ikonomov and Manji 1999; Berk 2009; Machado-Vieira et al. 2009; Berk et al. 2011b). It is a downstream target of monoaminergic systems and is thus implicated in mood regulation and neurocognition (Einat et al. 2006). During mania, GSK-3β is activated via dopamine overactivity (Beaulieu et al. 2004), and interestingly manipulations of GSK-3β can cause hyperactivity in animal models (Prickaerts et al. 2006). In the context of bipolar disorder and the effects of lithium, two key GSK-3β-mediated pathways will be discussed in the following sections (see Fig. 3.1).
3.4.1 The Excitotoxic Damage-GSK-3β-mTOR Cell Survival Pathway
GSK-3β is a component of the cell survival signalling pathway, which plays a critical role in multiple processes, including metabolism, proliferation, differentiation, cell structure, axogenesis and synaptogenesis (Ikonomov and Manji 1999; Gould et al. 2006; Berk 2009; Machado-Vieira et al. 2009; Berk et al. 2011b). Oxidative and nitrosative damage-mediated excitotoxicity activates the production of GSK-3β (Stokes et al. 1999; Obata 2002; Grima et al. 2003). However, lithium directly inhibits GSK-3β activity (Klein and Melton 1996; Chalecka-Franaszek and Chuang 1999; Manji and Lenox 2000b) and enhances inhibition of GSK-3β activity through other cellular process (Chalecka-Franaszek and Chuang 1999; Grimes and Jope 2001). GSK-3β inhibition activates the mammalian target of rapamycin (mTOR), which is an inhibitor of the autophagy process (Sarkar et al. 2008). Therefore, lithium inhibition of GSK-3β increases mTOR activity, which decreases neural degradation and increases neuroprotection through the facilitation of the Akt pathway (Tajes et al. 2009). These actions of lithium, therefore, inhibit autophagy and are likely to be protective for neurocognitive dysfunction. In addition to being involved in the pathophysiology of bipolar disorder, the excitotoxic damage-GSK-3β-mTOR pathway is implicated in the pathophysiology of neurodegenerative disorders (Parlato and Liss 2014) such as Alzheimer’s (Hooper et al. 2008; Pei and Hugon 2008) and Parkinson’s (Liu et al. 2013; Jiang et al. 2013; Li et al. 2014), with GSK-3β inhibition and mTOR being potential therapeutic targets. In addition to the impact of GSK-3β on the mTOR pathway, the inhibition of GSK-3β leads to modulation of neurotrophin expression.
3.4.2 The Excitotoxic Damage-GSK-3β-CREB-Neurotrophin Encoding Pathway
Neuroprogression in bipolar disorder occurs because of the excitotoxic consequences of recurrent affective episodes and reductions in cellular resiliency with time (Manji and Chen 2002; Rajkowska 2002). The excitotoxic damage-GSK-3β-CREB-neurotrophin encoding pathway is implicated in the neuroprogression of bipolar disorder and its effective treatment with lithium (Berk et al. 2011b; Malhi et al. 2013). GSK-3β inhibits the transcription factors β-catenin and cyclic AMP (cAMP) response element binding protein (CREB), by phosphorylation. This results in a decrease in the transcription of important genes encoding for neurotrophins (Gould et al. 2006; Böer et al. 2008; Berk et al. 2011b). Neurotrophins such as brain-derived neurotrophic factor (BDNF) and B-cell lymphoma 2 (Bcl-2) play a key role in neuronal survival and proliferation, and these are implicated in the neuroprogressive pathways of bipolar disorder (Kim et al. 2010; Berk et al. 2011b). BDNF is decreased in acute episodes of mania and depression (Cunha et al. 2006; Kapczinski et al. 2008; de Oliveira et al. 2009), and decreases in the BDNF levels correlate with increasing severity of clinical episodes (Cunha et al. 2006; Machado-Vieira et al. 2007; Fernandes et al. 2009). BDNF levels decrease during acute episodes, with further decrements as the disorder advances (Kapczinski et al. 2008), thereby perpetuating neuroprogression (Post 2007). However, in animals it has been shown that after 5 days of lithium administration, BDNF levels increase (Hashimoto et al. 2002) and, in patients, this delay is thought to reflect the time required for BDNF to reach neuroprotective levels and achieve mood-stabilizing and neurocognitive effects (Einat et al. 2006). This is supported by the finding that lithium nonresponders have lower BDNF levels than both lithium responders and healthy controls, suggesting further that a specific therapeutic effect of lithium is to facilitate BDNF (Rybakowski and Suwalska 2010). This is also in keeping with the finding that patients who responded to treatment during a manic episode had increased BDNF after the resolution of the episode (Tramontina et al. 2009). Both lithium’s direct and indirect effects on GSK-3β facilitate BDNF expression through CREB activation (Tramontina et al. 2009), with lithium’s additional actions mediated via cAMP (Lien et al. 2008; Manji et al. 2000; Chang et al. 2009).
In addition to BDNF, Bcl-2 is a neuroprotective protein that regulates cellular apoptotic pathways, preventing degradation (Manji et al. 2000) and, like BDNF, decreases in Bcl-2 are associated with mania (Lien et al. 2008). Interestingly, increased CREB increases Bcl-2 expression (Lien et al. 2008; Manji et al. 2000; Chang et al. 2009), thereby providing another action that facilitates neuroprotection. Similarly, chronic lithium therapy increases Bcl-2 expression (Chen and Chuang 1999), which reduces apoptosis (Ghribi et al. 2002). Therefore, increased BDNF and Bcl-2 expression with lithium treatment is thought to be a key mechanism by which it provides neuroprotection against glutamate-induced excitotoxicity (Hashimoto et al. 2002). However, the system is complicated and multifaceted given that reduced activation of the G-protein coupled dopamine receptor and the NMDA receptor regulate the second messenger excitotoxic damage-GSK-3β-mTOR and excitotoxic damage-GSK-3β-CREB-neurotrophin encoding pathways (Jope 1999; Manji and Lenox 2000a; Gould et al. 2002; Brunello and Tascedda 2003; Montezinho et al. 2007; Marmol 2008).
Hence, though the various potential cellular signal transduction mechanisms underpinning the actions of lithium on neural degradation and neuroprotection have been reasonably well characterized (see Malhi et al. 2016a), the precise pathways that are important in producing specific therapeutic outcomes remain unknown (Malhi et al. 2013). The manner in which these pathways possibly converge and produce specific therapeutic outcomes is also unclear. However, it appears that GSK-3β is fundamental to the therapeutic actions of lithium in terms of inhibiting neuroprogression in bipolar disorder (Freland and Beaulieu 2012; Ikonomov and Manji 1999; Machado-Vieira et al. 2009; Berk et al. 2011b; Malhi et al. 2013). However, the manner in which GSK-3β inhibition translates to clinical outcomes, or can be an effective sole treatment target, is unknown (Eldar-Finkelman 2002). Therefore, it is perhaps useful to consider the effects of lithium on neurocircuitry and how they relate to neuroprogression in bipolar disorder as an intermediate level of understanding between cellular changes and clinical outcomes.
3.5 Neurocircuitry
In addition to considering neurotransmitter and cellular signal transduction mechanisms that putatively underlie neuroprogression in bipolar disorder, it is important to also consider the structure and function of brain neurocircuitry, given that this is necessarily more proximal to clinical dysfunction of neurocognition and the capacity to regulate mood (see Fig. 3.2; Berk et al. 2011b; Fries et al. 2012; Malhi et al. 2013, 2015).
Bipolar disorder is associated with structural and functional abnormalities involving structures in the fronto-limbic network, which have implications for deficits in neurocognition and mood dysregulation (Emsell and McDonald 2009; Strakowski et al. 2012; Phillips et al. 2015; Malhi et al. 2015).
3.5.1 Structure
Bipolar disorder patients have reduced grey matter in the prefrontal cortex (Brooks et al. 2009) and anterior limbic regions (Bora et al. 2010). The reductions are widespread and include areas of the dorsomedial and ventromedial prefrontal cortex, the anterior cingulate cortex, insula and hippocampus. The rate of grey matter volume reduction is more rapid in bipolar disorder patients compared with controls (Brambilla et al. 2001; Lyoo et al. 2006) and different in some key regions such as the hippocampus relative to major depressive disorder (Wise et al. 2016). Grey matter loss is attributed to episodic excitotoxicity (Yksel and Öngür 2010) and subsequent oxidative and nitrosative damage (Rajkowska 2002), which is reflected in reduced neuronal densities (Ongür et al. 1998; Rajkowska et al. 2001). These findings are consistent with the neurotransmission and cellular signal transduction findings that suggest bipolar disorder is a neuroprogressive disorder (Berk et al. 2011b; Fries et al. 2012). Prefrontal and limbic structural abnormalities in bipolar disorder are thought to be related to executive control, emotion processing and mood regulation difficulties and other neurocognitive function abnormalities observed clinically (Bora et al. 2009, 2010; Berk et al. 2011b).
Effective lithium treatment normalizes the fronto-limbic network structural deficits implicated in bipolar disorder. Lithium-treated patients, compared with non-treated patients or healthy controls, show increases in total (Sassi et al. 2002; Bearden et al. 2007; Kempton et al. 2008) and regional prefrontal (Adler et al. 2005; Bearden et al. 2007) and limbic grey matter volume (Yucel et al. 2008; Foland et al. 2008; Usher et al. 2010; Bora et al. 2010; Hallahan et al. 2011; Hajek et al. 2012; van Erp et al. 2012), with increases in prefrontal grey matter volume shown to be specifically associated with lithium response (Moore et al. 2009). This is supported by the finding in rats that chronic administration of lithium promotes proliferation of neurons in the hippocampus (Chen et al. 2000). These findings clearly highlight the potential neuroprotective effects of lithium (Kempton et al. 2008).
3.5.2 Function
Patients with bipolar disorder display prefrontal cortex hypoactivity and limbic hyperactivity during emotional and cognitive tasks, and these findings correlate with trait and state emotional lability and mood disturbances (see Strakowski et al. 2012). In addition, bipolar disorder is characterized by dysfunctional connectivity among ventral prefrontal networks and limbic brain regions, particularly the amygdala (Chen et al. 2011; Houenou et al. 2011; Townsend and Altshuler 2012; Blond et al. 2012; Strakowski et al. 2012), indicating both difficulty in regulating mood and a dysfunction of emotion processing. Impaired prefrontal cortex regulation subsequently leads to a loss of neurological emotional homeostasis, emotional lability and mood disturbances (Strakowski et al. 2012). It is posited that a disruption of frontal regulatory networks allows for extreme mood states, switching among mood states and mixed states. These abnormalities have been conceptualized as dysfunction within oscillatory mechanisms, which perhaps worsen over time, and result in the many manifestations of the illness (Schneider et al. 2012; Malhi et al. 2015).
In comparison to the impacts of neuroprogression on the neurocircuitry of bipolar disorder, the impacts of lithium therapy are relatively understudied. In functional neuroimaging studies, lithium administration is associated with prophylactic effects on neurocircuitry after 14 days of treatment, acting on frontal regions in the euthymic phase of bipolar disorder with little impact during the depressed phase (Silverstone et al. 2005; Bell et al. 2005a, b). There appears to be normalization of the cognitive and emotional neural networks implicated in bipolar disorder with treatment when patients present in the depressed or manic phases of illness (Silverstone et al. 2005; Bell et al. 2005a, b). Thus, the impacts of lithium on neural processing are broad, yet the observed effects are dependent on both mood state and the task undertaken (Silverstone et al. 2005; Bell et al. 2005a, b). However, this line of research is preliminary given the relatively small sample sizes recruited by most studies to date. In terms of neurocognitive assessment-based findings, we have found that the benefits of lithium for neurocognitive functions—such as executive function, attention, memory and learning and processing and psychomotor speed—are mixed and need to be clarified and replicated in longitudinal research (for review, see Malhi et al. 2016b).
Nevertheless, there are promising findings from these relatively new lines of research that should encourage future work. Such research may provide further insights into the impacts of lithium on the neurocircuitry affected by neuroprogression (Malhi et al. 2013) and afford clinically useful markers for predicting treatment response and treatment monitoring (see Outhred et al. 2014).
The neuroprotective effects of lithium in bipolar disorder patients have been proposed on the basis of preservation of grey matter volume and functional changes within fronto-limbic neurocircuitry. However, the mechanisms linking these changes to neurotransmission and cellular signal transduction changes, and the significance of these for mood dysregulation, are not fully understood. Given the broad effects of lithium on neurotransmission, cellular signal transduction mechanisms and brain neurocircuitry, determining the specific pathways leading to beneficial therapeutic outcomes with lithium through changes in neural functioning poses the next challenge for research in this domain.
3.6 The Impact of Lithium on Mechanisms Underlying Neuroprogression in Bipolar Disorder
Given that lithium interacts with multiple targets across a number of neurotransmitter systems, more sophisticated and integrated investigations are required to unravel the complexity of its actions on neurotransmission. As such, it is difficult to pinpoint the specific mechanisms that are involved in producing the therapeutic effects of lithium for bipolar disorder. With further elucidation of these processes, it is envisaged that the potential targets for lithium and the development of novel medications for bipolar disorder, as well as other neurodegenerative disorders, will be realized (Chuang 2005; Chuang and Manji 2007; Marmol 2008). Regardless, it is likely that the actions of lithium on modulating dopaminergic, glutamatergic and GABAergic neurotransmission, which in turn impact cellular signal transduction pathways, are involved in inhibiting neurodegeneration and promoting neuroprotection, key benefits in themselves.
Based on extant research, the impacts of lithium on neurotransmission and cellular signal transduction mechanisms underlying neuroprogression can perhaps be best understood in the context of its effects on the neurocircuitry involved in mood dysregulation and neurocognitive dysfunction that characterize bipolar disorder. In a simplified manner, Fig. 3.1 depicts the actions of lithium in terms of inhibition and facilitation of several neurotransmitters and cellular signal transduction mechanisms involved in neurodegeneration and neuroprotection. Key actions appear to be the attenuation of excitotoxic dopaminergic and glutamatergic neurotransmission and facilitation of inhibitory GABAergic neurotransmission and the consequent and direct modulation of excitotoxic damage-GSK-3β-mTOR and excitotoxic damage-GSK-3β-CREB-neurotrophin encoding pathways. These mechanisms are thought to underpin the therapeutic effects of lithium that ultimately ameliorate the neurocircuitry dysfunction and mood dysregulation and neurocognitive functions that occur in bipolar disorder. Presently, much more is known about the changes to the neurotransmitter and cellular signal transduction pathways with lithium treatment than is known about how these changes then translate to clinical outcomes (Malhi et al. 2013). By examining the specific effects of integrating them within a framework of therapeutic changes that rectify mood dysregulation and neurocognitive dysfunction, future research may be guided towards a more complete depiction of the pathophysiology of bipolar disorder and its modification with lithium therapy.
3.7 Future Research
Given that lithium has broad effects on multiple processes that are potentially therapeutic (Malhi et al. 2013; 2016a), the task for researchers is to isolate those processes that lead to therapeutic response. By investigating each level and every process within a level (see Fig. 3.2), future research should be able to correlate neurotransmission, cellular signal transduction pathway and neurocircuitry changes to therapeutic improvement, specifically restoration of neurocognition. In doing so, the aetiology of bipolar disorder and its lifelong neuroprogression is likely to be better understood.
For lithium treatment, researchers need to associate changes in markers of neurotransmission and cellular changes with changes in the structure and function of key neurocircuits (Malhi et al. 2016a). Research into the precise effects of lithium treatment on neurocircuitry underpinning mood dysregulation and neurocognition is currently lacking. Future research along these lines may also provide valuable insights into the anti-suicidal and neuroprotective properties of lithium along with its mood-stabilizing effect and prophylaxis. Determining the specific pathways leading to therapeutic outcome in patients may also open up avenues for the development of more specific treatment targets. In doing so, the development of new pharmaceuticals that focus more specifically on neuroprogressive processes may be facilitated by employing reverse engineering (Malhi et al. 2013). Drugs that selectively target key therapeutic processes may have greater tolerability, reduced risk and wider application.
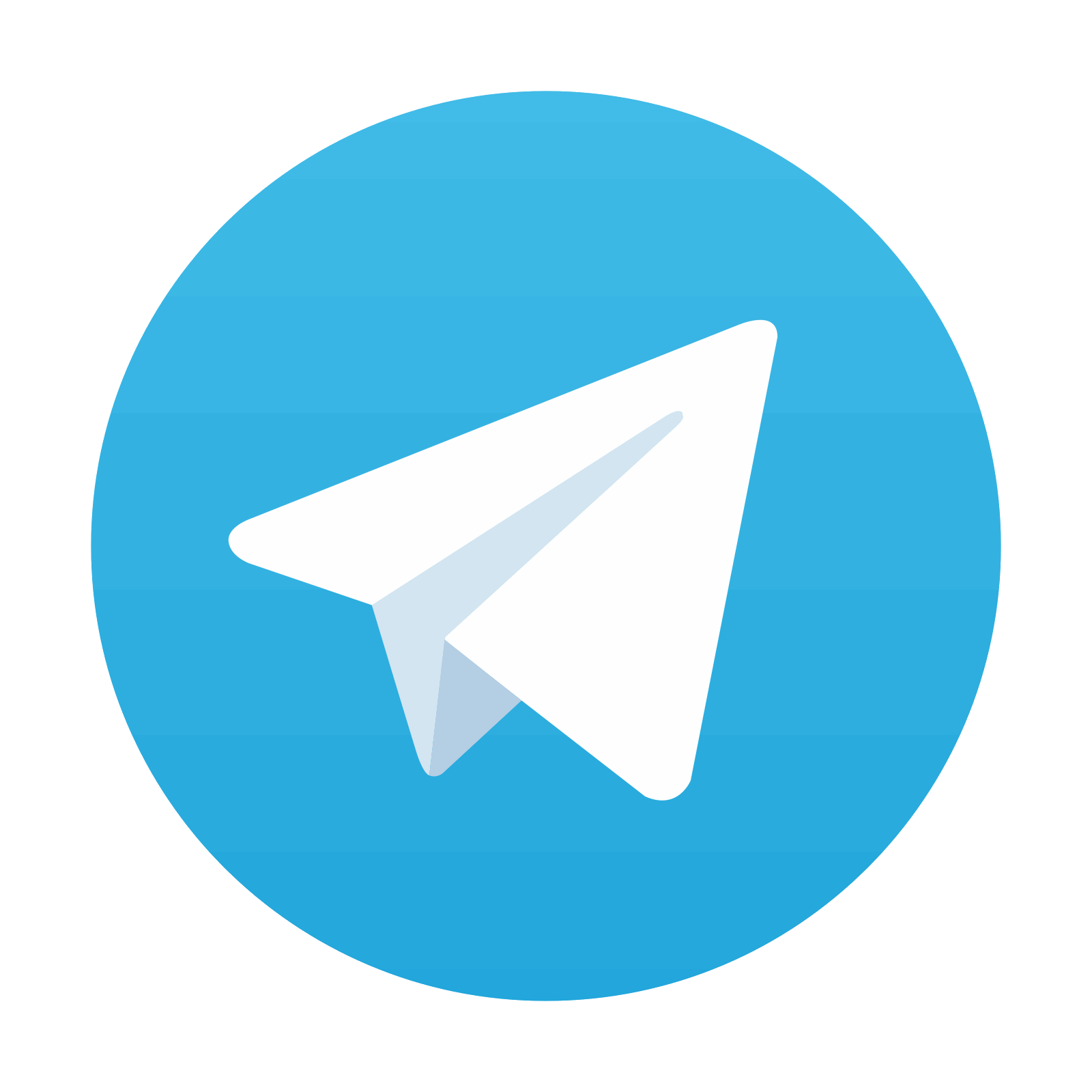
Stay updated, free articles. Join our Telegram channel

Full access? Get Clinical Tree
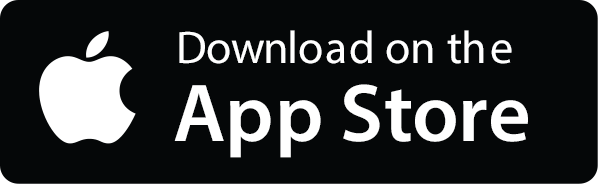
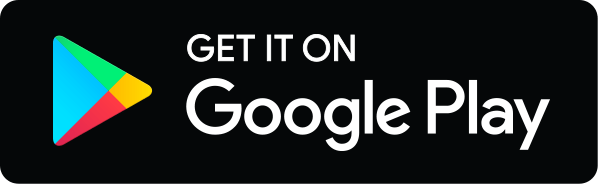