Fig. 2.1
Afferent nociceptive transmission utilizes a three neuron relay that involves nociceptors from the periphery that terminate in the dorsal horn of the spinal cord, get transmitted to higher centers including the thalamus, and then proceed to either the lateral or medial nociceptive pathways and structures. Descending pain modulation is initiated in the frontal cortex, amygdala, and hypothalamus, pass through the periaqueductal gray (PAG), and rostral ventromedial medulla (RVM) and terminates again in the spinal cord where it can influence subsequent ascending nociception
Rheumatoid Arthritis
RA is characterized by systemic inflammation that can be related to pain, stiffness, and damage to joints. Referring to the model of pain just presented, there are a number of peripheral drivers associated with initiating and maintaining the nociceptive cascade in RA including mechanical stimulation (e.g., weight bearing and joint movement), nociceptive factors in the synovium or synovial fluids, inflammatory cytokines (e.g., IL-6, TNF), and growth factors (Walsh & McWilliams, 2014). Biomedical treatment of RA often includes direct-acting analgesic agents such as nonsteroidal anti-inflammatory agents (NSAIDs) , agents that suppress inflammation such as glucocorticoids, DMARDs, and biologics (e.g., TNF blockers), and surgical approaches such as joint replacement (Walsh & McWilliams, 2014). For around 25 % of patients however, pain does not improve despite the use of anti-inflammatory agents and another 15 % are left with pain after completely removing and replacing the joint (e.g., 15 %) (Walsh & McWilliams, 2012). It is suspected that in these cases, while peripheral mechanisms are obviously active, there may be other centrally mediated aspects of nociception (e.g., higher cortical or descending modulatory influences) that are also contributing prominently to pain perception. For example, in studies of RA, subgroups of individuals with RA have been identified who have both lowered pain thresholds and impaired central descending analgesic activity (Gerecz-Simon, Tunks, Heale, Kean, & Buchanan, 1989; Hummel, Schiessl, Wendler, & Kobal, 2000), suggesting more involvement of the CNS in maintaining pain for these individuals.
Osteoarthritis
OA, found predominantly in elderly individuals (Lee et al., 2013) is characterized by degradation to articular cartilage, bone, synovial joint lining, and adjacent connective tissue (Zhang, Ren, & Dubner, 2013).
Historically, OA has been considered a prototypic nociceptive pain condition with peripheral mechanical and inflammatory influences triggering the pain. As such, treatments for OA have historically been peripherally focused and based upon relieving symptoms through direct-acting analgesic agents (e.g., NSAIDs), anti-inflammatory (e.g., intra-articular glucocorticoid injections), and joint replacement surgery (Hassan & Walsh, 2014). As in the case of RA however, many individuals do not respond to these standard interventions (Zhang et al., 2013). For example, despite undergoing total knee replacement surgery, 44 % of OA patients still report pain 3–4 years after surgery, with 15 % reporting it as severe (Wylde, Hewlett, Learmonth, & Dieppe, 2011). Failure to respond to surgical or peripheral agents draws into question whether pain is a direct correlate of damage. Population-based studies suggest it is not. These studies report that 30–50 % of individuals with moderate to severe radiographic changes of OA can actually report no pain; whereas 10 % of individuals with normal radiographs report moderate to severe knee pain (Creamer & Hochberg, 1997; Hannan, Felson, & Pincus, 2000). As with RA, when peripherally directed therapies are ineffective with OA, pain might be best attributed to central pain mechanisms (Hassan & Walsh, 2014; McDougall & Linton, 2012).
Central Pain Augmentation: Terminology
The term “central pain” originally referred to pain from identifiable lesions to the CNS such as those following a stroke or spinal cord injury. The term “central” was used to differentiate this type of nerve damage from peripheral nerve damage (i.e., neuropathic pain—such as trauma or diseases like diabetic neuropathy). More recently, however, the meaning of the term “central pain” has been expanded to describe any CNS dysfunction or pathology that may be contributing to the development or maintenance of chronic pain (Williams & Clauw, 2009) and is perhaps better termed “centralized pain” to describe pain that is influenced predominantly by the CNS.
Another term that often shares a similar meaning to centralized pain is “central sensitization.” Central sensitization originally referred to a very specific spinal mechanism that could account for pain perception exceeding what would be expected from peripheral tissue damage alone (Woolf & Thompson, 1991). In experimental studies, central sensitization has been characterized by the presence of tactile allodynia, secondary punctuate/pressure hyperalgesia, temporal summation, and sensory after effects (Woolf, 2011). Clinically, the hypersensitivity of central sensitization has been described as being disproportionate to the nature and extent of any injury (i.e., not nociceptive pain) and not being attributable to lesions or damage within the CNS (i.e., not neuropathic pain). Phenotypic characteristics of central sensitization include a widespread pain distribution, allodynia and/or hyperalgesia, and may include general hypersensitivity of all senses and perceptual systems (e.g., pressure, chemicals, heat/cold, stress, emotions, and mental load) (Nijs, Malfliet, Ickmans, Baert, & Meeus, 2014; Woolf, 2014).
CNS factors provide “gain” (using an electrophysical analogy) by which peripheral nociception is augmented or diminished in the determination of whether the nociceptive information is salient and subsequently painful (Legrain, Iannetti, Plaghki, & Mouraux, 2011). In nociceptive pain states, this gain appears to operate at a set point that facilitates a fairly good correspondence between the degree of tissue damage and the intensity of pain. In aberrant central pain states, this correspondence can be mismatched such that seemingly innocuous stimuli are experienced as being painful. A number of neurotransmitters and centrally mediated processes appear to be involved in determining this set point (Clauw, 2014).
In the next section of this chapter, we refer to pain arising from a predominance of CNS influences (e.g., set point, sensory augmentation, salience, and central sensitization) as “centralized pain.” In referring to centralized pain mechanisms, we also acknowledge that most forms of arthritis pain will be “mixed pain states” (i.e., incorporating a balance of peripheral and central drivers) (Phillips & Clauw, 2013).
Centralized Pain: Characteristics and Mechanisms
Centralized pain, as defined here, was originally thought to be confined to individuals with idiopathic or functional pain syndromes, such as fibromyalgia (FM), headache, irritable bowel syndrome (IBS), temporomandibular joint disorder (TMD), and interstitial cystitis (IC) (Clauw et al., 1997; Hudson & Pope, 1994). These pain syndromes have been shown to be familial/genetic, as they strongly coaggregate within individuals and within families (Diatchenko, Nackley, Slade, Fillingim, & Maixner, 2006; Williams & Clauw, 2009). The symptoms experienced by individuals with centralized pain syndromes have been well characterized and consist of multifocal pain (with a high current and lifetime history of pain in many bodily regions), and a cluster of cooccurring somatic symptoms (i.e., fatigue, sleep disturbances, difficulties with thinking/memory) (Warren et al., 2009; Williams & Clauw, 2009). We know now that these central influences are not just limited to individuals with conditions like FM but can influence pain perception for a variety of chronic pain states under a “mixed-pain state” model.
Multifocal Pain and Cooccurring Somatic Symptoms
Being prone to pain augmentation via central influences (i.e., having a low set point for pain) is a lifelong condition usually beginning in young adulthood and manifested by multiple prolonged pain experiences occurring in many different body regions and over many different time periods (Tracey & Bushnell, 2009; Williams & Clauw, 2009; Woolf, 2011). Over a lifetime, such individuals tend to accumulate multiple diagnostic labels associated with various regions of the body; but in all likelihood, aberrant central pain mechanics underlie much of this symptomatology.
Multifocal pain is thought to be related to pathophysiologic excitatory neurotransmitter activity such as high substance P and high glutamate levels in cortical structures associated with afferent pain processing (i.e., part of the “gain” in determining the central pain set point). In addition, descending pain inhibitory pathways depend upon adequate levels of norepinephrine, GABA, or serotonin, which in centralized pain conditions tend to be low (i.e., also enhancing the “gain” that determines the set point for pain) (Clauw, 2014; Williams & Clauw, 2009).
While the aforementioned neurotransmitters are critical to pain perception, they also mediate the symptoms that can accompany multifocal pain such as fatigue, sleep difficulties (e.g., insomnia or nonrefreshing sleep), thinking and memory problems, and mood disturbances (Bannister, Bee, & Dickenson, 2009; Fukuda et al., 1997, 1998; Williams & Clauw, 2009). The broader role of these neurotransmitters in both multifocal pain and in these cooccurring symptoms is best supported by the fact that when centrally acting analgesics such as serotonin–norepinephrine reuptake inhibitors (SNRIs), gabapentinoids, tricyclics, or gamma-hydroxybutyrate are effective in patients suspected of having centralized pain involvement, these drugs also lead to improvements in one or more of these other symptom domains (Fishbain, Detke, Wernicke, Chappell, & Kajdasz, 2008; Russell et al., 2011; Tzellos et al., 2010). Thus, the assessment of these cooccurring symptoms is useful in identifying the presence of a centralized pain state and for identifying likely responders to pharmacological therapies targeting centralized pain states (Aaron, Burke, & Buchwald, 2000; Arnold et al., 2012; Williams & Clauw, 2009).
Hyperalgesia
Another hallmark characteristic of centralized pain conditions is the presence of diffuse hyperalgesia identifiable using quantitative sensory testing (QST) and corroborated by functional neuroimaging (Clauw, 2009; Diatchenko, Nackley, Slade, Fillingim, et al., 2006; Tracey & Bushnell, 2009; Woolf, 2011). Key to understanding the relevance of hyperalgesia in centralized pain states is the term “diffuse” which emphasizes the point that hyperalgesia is not confined to a location of injury per se; but rather, is present over noninjury sites as well.
Within both the general population and within chronic pain conditions, sensory sensitivity is normally distributed with some individuals having higher pain thresholds and others having lower pain thresholds. A low pain threshold is disproportionately seen in those individuals with a centralized pain condition (Coghill, McHaffie, & Yen, 2003; Diatchenko, Nackley, Slade, Fillingim, et al., 2006; Gibson, Littlejohn, Gorman, Helme, & Granges, 1994; Giesecke, Gracely, et al., 2004; Giesecke, Reed, et al., 2004; Gwilym et al., 2009; Kashima, Rahman, Sakoda, & Shiba, 1999; Kosek, Ekholm, & Hansson, 1995; Leffler, Hansson, & Kosek, 2002; Maixner, Fillingim, Booker, & Sigurdsson, 1995; Tracey & Bushnell, 2009; Whitehead et al., 1990; Williams & Clauw, 2009) but can occur in other pain states (e.g., OA and RA) where subgroups of individuals display more of a “mixed-pain state” presentation (Gerecz-Simon et al., 1989; Hummel et al., 2000).
The baseline presence of hyperalgesia has also been shown to be an important risk factor for a number of adverse pain outcomes, including predicting the subsequent intensity of an acute painful experience, predicting increased analgesic requirements following surgery, and the subsequent transition from an acute to a chronic pain state (Arendt-Nielsen & Yarnitsky, 2009; Granot et al., 2008; Yarnitsky et al., 2008). This latter phenomenon (i.e., the transition from an acute to chronic pain state) was first demonstrated in a study by Diatchenko and colleagues, who performed a longitudinal study of 202 young pain-free women, and followed them for 2 years with the outcome of interest being those who developed new onset TMD (Diatchenko et al., 2005). In this study, an individual’s pain threshold at baseline (i.e., while completely asymptomatic) was a strong predictor of who would later develop TMD. In fact, those with a lower pain threshold while asymptomatic were three times more likely to develop TMD in the future than individuals with higher pain thresholds.
The above study raises the question of what might determine an asymptomatic baseline threshold for pain. In addition to demonstrating the importance of hyperalgesia in predicting the onset of new pain, this same TMD study was among the first to highlight the strong role that certain genes play in turning up the “gain” on pain processing (Diatchenko et al., 2005; Diatchenko, Nackley, Slade, Bhalang, et al., 2006; Diatchenko, Nackley, Slade, Fillingim, et al., 2006).
Genetics of Centralized Pain States
While several rare instances of single gene mutations associated with pain exist (Cox & Wood, 2013; Eijkelkamp et al., 2012), most instances of pain perception stem from polygenetic influences (Denk, McMahon, & Tracey, 2014). The genetic loci most associated with pain are those involving neurotransmitter systems (e.g., COMT, OPRM1, GCH1, 5HTR2A, ADRB2), ion channel functions (e.g., KCNS1, CACNA2D3), and immune functioning (IL1, TNF) (Denk et al., 2014; Mogil, 2012). In centralized pain states, genetic factors associated with metabolism or transport of monoamine compounds associated with sensory processing (e.g., heightened sensory sensitivity) and/or affective vulnerability and stress appear to be the most relevant in predicting the onset and maintenance of the condition (Buskila, 2007; Diatchenko, Nackley, Slade, Fillingim, et al., 2006).
A number of environmental “stressors” have also been associated with centralized pain states. These include early life trauma, physical trauma, certain infections such as Hepatitis C, Epstein–Barr virus, parvovirus, Lyme disease, emotional stress, and other regional pain or autoimmune disorders (Ablin & Clauw, 2009; Buskila, Neumann, Vaisberg, Alkalay, & Wolfe, 1997; Clauw & Chrousos, 1997). While these studies are informative, there does not appear to be any singular “cause” of centralized pain conditions; rather, in a genetically predisposed individual (i.e., someone predisposed to sensory hypersensitivity and/or affective vulnerability), any of these stressors can act as a temporary trigger for the subsequent development of the condition. The role of genetic predisposition is important given that in nonpredisposed individuals (i.e., 90 % of individuals), these same stressors tend to resolve and individuals regain their baseline state of health.
Conditioned Pain Modulation
As stated, there are central mechanisms that can influence the perception of pain. Conditioned pain modulation (CPM) or as it was previously labeled DNIC (i.e., diffuse noxious inhibitory controls) refers to studying the integrity of the descending endogenous analgesic pathways. CPM currently holds great promise as a means of “segmenting” individuals with chronic pain into those with and those without a central predominance to their pain.
The integrity of the pathway and the magnitude of pain inhibition can be tested experimentally by using two separate painful stimuli and observing how the experience of the first reduces the perceived intensity of the second. CPM is a powerful analgesic effect and is observed in 80–90 % of healthy individuals. It is attenuated or absent, however, in 60–80 % of individuals with centralized pain conditions (e.g., FM or IBS) (Edwards, Ness, Weigent, & Fillingim, 2003; Julien, Goffaux, Arsenault, & Marchand, 2005; Kosek & Hansson, 1997; Le Bars, Villanueva, Bouhassira, & Willer, 1992; Pud, Granovsky, & Yarnitsky, 2009; Wilder-Smith & Robert-Yap, 2007). Both CPM (i.e., descending pain modulation) and hyperalgesia (i.e., ascending pain processing) appear to be unique characteristics of centralized pain and are not seen in other conditions that hold high comorbidities with chronic pain such as depression (Giesecke et al., 2005; Normand et al., 2011).
Neuroimaging Studies
Perhaps some of the strongest evidence pointing to aberrant central mechanisms playing a predominant role in centralized pain states comes from functional, chemical, and structural neuroimaging studies. To date, numerous studies have shown significantly increased neuronal activity in pain processing regions of the brain when individuals with central pain states are exposed to stimuli that healthy individuals find innocuous (Cook et al., 2004; Giesecke, Gracely, et al., 2004; Gracely, Petzke, Wolf, & Clauw, 2002; Naliboff et al., 2001). Such findings have been used to support the notion that patients’ reports of pain to innocuous stimuli actually correspond with cortical pain processing activity rather than being attributable to biases in pain reporting or to hypervigilance on the part of the patient.
Neuroimaging studies have also helped to identify the separate but critical roles of both the sensory pathways and the affective pathways in creating a unified perception of pain. For example, within a single brain region such as the insula, the posterior insula is more involved in sensory processing whereas the anterior insula is more involved in affective processing. Even the left-to-right balance of insular activity may be associated with the emotional valence of pain (Craig, 2003). Recent studies also suggest that the balance between sensory and affective dimensions of pain do not remain stable even within the same individual, with the same injury, over time. For example, an initial injury may appear with the cortical signature of a sensory event; however with chronicity, pain can take on a cortical signature more closely resembling an emotion (Hashmi et al., 2013). This may be why attempts to treat chronic pain in the same way as acute pain (e.g., with peripherally acting agents) often fail (Lee et al., 2011).
Mechanism-Based Treatment
Historically, medical treatment of arthritis has focused upon treating the underlying disease process, which as stated, may or may not share a close relationship with pain. As such, the most common medical approach to arthritis pain is the use of nonsteroidal anti-inflammatory drugs (NSAIDs) or surgery. When patients with arthritis are nonresponsive to such pain treatment, they may have a stronger central driver of pain. This can be identified by the characteristics reviewed earlier in this chapter (e.g., chronic multifocal pain, multiple comorbid centrally mediated somatic symptoms, diffuse hyperalgesia, attenuated CPM, and ruling out nociceptive and neuropathic mechanisms). Given that central mechanisms act to enhance the gain on nociception, interventions that calm the CNS and/or restore balance within afferent and descending inhibitory pathways hold promise of being beneficial (Woolf, 2011). These treatments could be either biomedical or nonpharmacological in nature.
Examples of pharmacological interventions that have shown benefit in centralized pain conditions such as FM include tricyclic antidepressants (TCAs), SNRIs, and alpha-2 delta ligands. TCAs have many actions but are generally thought to exert their analgesic effects by inhibiting the reuptake of serotonin and norepinephrine. While a number of studies offer support for the use of TCAs in FM (Nishishinya et al., 2008), far fewer have examined their use in OA or RA. Those that have, however, tend to report significant reductions in pain (Ash, Dickens, Creed, Jayson, & Tomenson, 1999; Chuck, Swannell, House, & Pownall, 2000; Frank et al., 1988; Gringras, 1976; Macfarlane, Jalali, & Grace, 1986; Sarzi Puttini et al., 1988) that are independent of improvements in depression (Ash et al., 1999; Macfarlane et al., 1986). A drawback of using this class of medication in arthritis patients, however, are the well-known side effects of this class of drugs which can include dizziness and sedation, blurred vision, constipation, and dryness of mouth. SNRIs act similarly to TCAs but tend to be more selective and have fewer side effects than TCAs. By selectively increasing the amount of available norepinephrine and serotonin, SNRIs are thought to help restore the functioning of the descending pain inhibitory pathway in centralized pain states (Lee et al., 2011). At least one clinical trial has supported the use of SNRIs in the management of OA pain (Chappell et al., 2009) but as of this writing, none have been conducted with RA pain. Finally, alpha-2 delta ligands are anticonvulsants and have been used successfully in the treatment of neuropathic pain conditions. This class of medication interferes with the release of pain-promoting neurotransmitters such as glutamate, noradrenaline, serotonin, and substance P. Clinical trials using this class of anticonvulsant in patients with central pain states have also demonstrated improvements in pain severity (Crofford et al., 2005, 2008).
The three most strongly supported nonpharmacological interventions for centralized pain states are education, cognitive-behavioral therapy (CBT), and exercise (Goldenberg, 2008; Goldenberg, Burckhardt, & Crofford, 2004). These nonpharmacological interventions tend to have treatment responses that equal or even exceed the magnitude of response found with pharmacological agents (Clauw, 2014). Over 80 studies support the use of exercise in central pain states with most showing improvements in pain intensity, improved functional status, and/or improvements in associated symptoms (Hassett & Williams, 2011). The type of exercise can vary (e.g., aerobic, strength training, flexibility training), with some evidence that pool-based exercise may be slightly more advantageous given reductions in weight bearing (Brosseau et al., 2008a, 2008b; Hauser et al., 2010). CBT has been used successfully with psychiatric conditions (e.g., anxiety and depression) (Hofmann & Smits, 2008; Twomey, O’Reilly, & Byrne, 2015) as well as in medical conditions such as cardiovascular disease (Lundgren, Andersson, & Johansson, 2015), diabetes (Pal et al., 2014), asthma (Creer, 2008), obesity (Van Dorsten & Lindley, 2011), tinnitus (McKenna, Handscomb, Hoare, & Hall, 2014), and insomnia (Wang, Wang, & Tsai, 2005). While the specific skills taught in each variation of CBT can differ, each version is grounded in shared psychological principles of behavioral change (e.g., operant and classical conditioning), social learning theory, and approaches for modifying thoughts, beliefs, and attributions about illness. This form of therapy, which incorporates elements of education, has been found to be beneficial in reducing pain and improving function in centralized pain conditions (Glombiewski et al., 2010; Rossy et al., 1999) as well as in OA and RA (Keefe & Caldwell, 1997; Keefe et al., 1991).
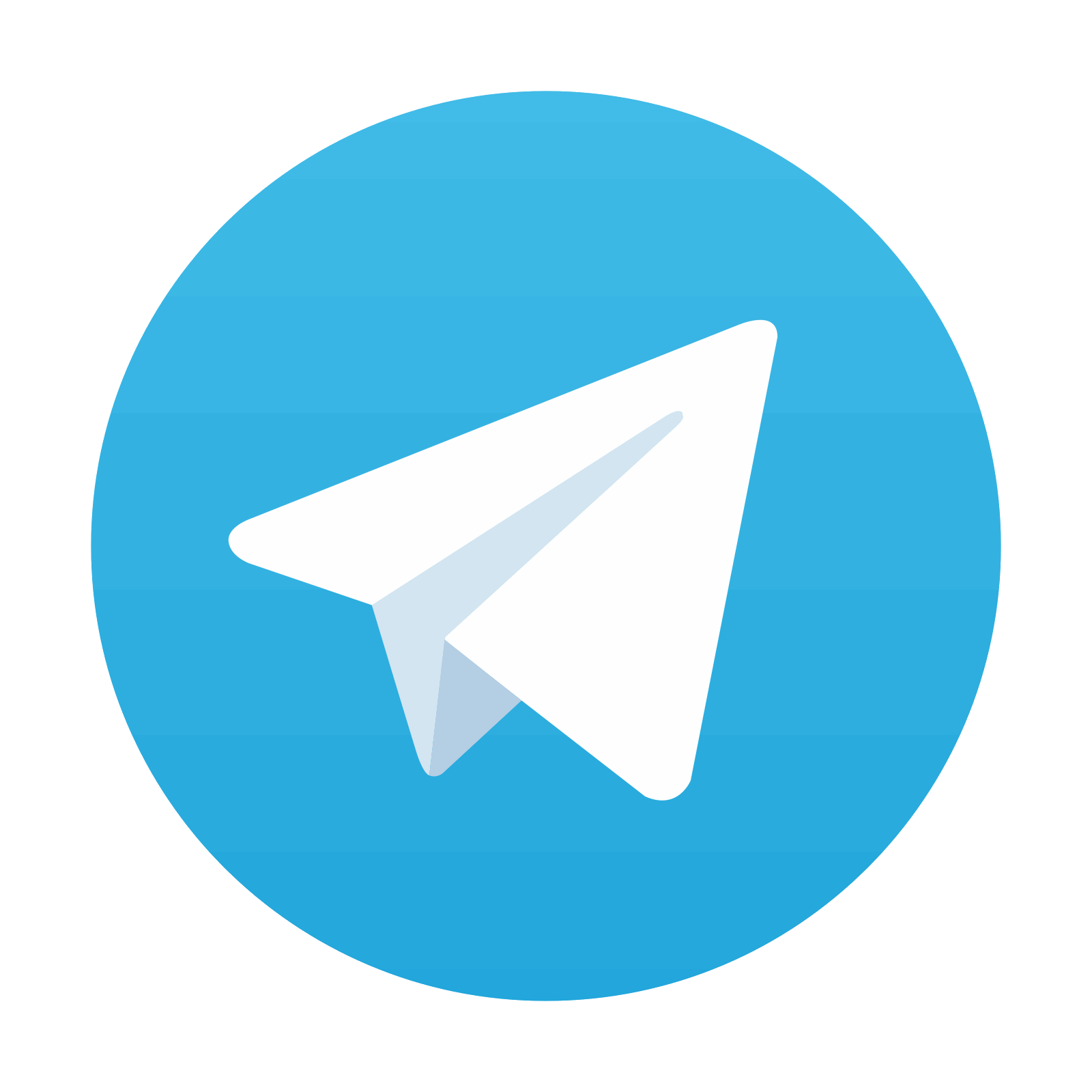
Stay updated, free articles. Join our Telegram channel

Full access? Get Clinical Tree
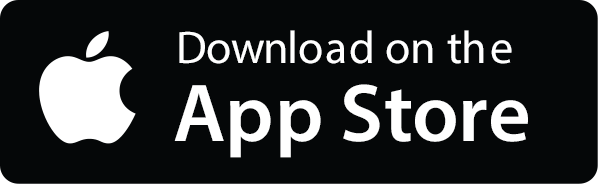
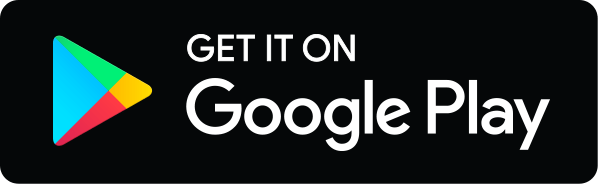