Chapter 29 Memory Processing in Relation to Sleep
Abstract
In 1867, Hervey de Saint Denys, fascinated by his dreams since the age of 14, published Les Rêves et les Moyens de les Diriger.1 In his book, he reported a series of ingenious experiments showing that experienced events are incorporated into our dreams, in which they can be combined to create original associations between “memory images” of the past. Hence, he strongly opposed the idea that sleep may be a sudden drop in a state of cognitive “non-being” in which our resting brain is disconnected. On the contrary, he claimed that “a sleep without dreams cannot exist, just as a wake state without mentation does not exist.” Besides dreaming activity, however, addressed in Section 7 of this book, we know that the sleeping brain houses a wide set of cognitive processes, including the ongoing treatment of elaborated external stimuli, the revival of experiences, and last, but not least, the consolidation of new information in memory. Interestingly, recognition that persistence of mental activity in the sleeper may be an integral part of the physiologic processes that subtend memory consolidation arose only in the last quarter of the 20th century. The hypothesis had to wait till now to receive widespread acknowledgement, as illustrated by the fact that for the first time a chapter devoted to sleep in relation to memory has been introduced in this fifth edition of Principles and Practices in Sleep Medicine.
This chapter introduces the issues surrounding the role that sleep may play in memory consolidation, focusing on human data. Reviews about relationships between sleep and memory in animals are addressed can be found elsewhere.2–4 We will here outline key findings and milestones in probing the sleep-for-memory hypothesis, as well as ongoing debates and thoughtful questions that remain. Before approaching the subject, however, we will briefly introduce the concepts of memory consolidation and memory systems in humans. Previous chapters have shown that sleep is a multidimensional state of vigilance, composed of rapid eye movement (REM) and non-REM (NREM) episodes that present distinctive features and rely on specific neuroanatomic substrates.4 From both cognitive and neurophysiologic perspectives, memory is not a unitary phenomenon. Rather, memory should be seen as a generic concept for information storage, encompassing a series of specific subdomains.5 Consequently, the interaction between multidimensional states of sleep and distinctive memory systems makes it logical that not all sleep manipulations will have the same impact on performance, depending on the various parameters embedded in the memory task.2,6,7 We will examine the growing number of behavioral studies that have enlightened our understanding of the role played by sleep episodes in memory consolidation. The rapid evolution of neurophysiologic techniques and analytical approaches allows scrutiny of the complex relations between cognitive processes and their underlying neural substrates. Part of this chapter will focus on the neurophysiologic mechanisms acting in sleep to support, or at least favor, memory consolidation processes. Molecular biology of memory consolidation is beyond the scope of this chapter and is reviewed elsewhere.4 The relationship between consolidation of learning and sleep is a crucial issue because memory is at the root of most of our daily behaviors, such as simple skill acquisition (e.g., typewriting), sophisticated operational procedures (e.g., using computer-based systems), and keeping track of personal events and relationships.
Memory Systems and Memory Consolidation
We are able to learn, store, and remember various types of information in different ways and for variable periods of time, from conscious acquisition strategies to incidental detection of environmental events. Cerebral damage can selectively alter some of these processes while leaving others undisturbed. These simple observations have led to the proposal that memory is not a unitary phenomenon but rather a complex construct of more or less specialized memory subdomains.5 First, a seminal distinction that dates back to William James is made between short- and long-term memory stores. The former is dedicated to the temporary storage of volatile information for up to seconds, whereas the latter, the main focus in this chapter, houses potentially lasting information that is deemed consolidated and less susceptible to disruption. Long-term memories in humans may further belong to multiple systems, primarily delineated between declarative and nondeclarative memories (Fig. 29-1).
Distinctive features of nondeclarative memories are that they are not easily accessible to verbal description and can be acquired and reexpressed implicitly. Thus, our behavioral performance can be affected by the new memory even if we are not consciously aware that new information has been encoded or is being retrieved. Memory abilities aggregated under the nondeclarative label also gather different forms: skills, habits, priming, and conditioning. Although grouped under the nondeclarative label, these various processes are subtended by distinct neuroanatomic substrates in both humans and animals, further suggesting their relative independence.5
Besides the transverse division between long-term memory subsystems proposed by these influential models, there is a dynamic longitudinal process. Newly acquired information is not immediately stored at the time of learning in its final form, if such a stable state exists. Rather, memories undergo a series of transformations over hours, days, or even years, during which time they are gradually incorporated into preexisting sets of mnemonic representations,8–10 or are subjected to forgetting.11 This is the concept of memory consolidation, which can be defined as the time-dependent process that converts labile memory traces into more permanent or enhanced forms.12 These transformations are made possible by our brain plasticity—that is, the capacity of the brain to modify its structure and function over time, within certain boundaries.13 Eventually, time-dependent processes of consolidation and the ensuing robust memory trace will enduringly adjust the behavioral responses to the recent environmental changes, thereby enlarging the organism’s behavioral repertoire.14
Scientific evidence suggests that sleep and the associated processes of brain plasticity4 are major players in time-dependent processes of memory consolidation, acting as key constituents in the chain of transformations that help integrate information for the long term. Furthermore, these studies suggest that distinct sleep stages may have distinct memory-related functions, which has been interpreted in two different but nonexclusive ways. According to the dual-process hypotheses, REM and NREM sleep act differently on memory traces depending on the memory system or process to which they belong. For example, it has been proposed that slow-wave sleep (SWS)—the deepest stage of NREM sleep—facilitates consolidation of declarative and spatial memories, whereas REM sleep facilitates consolidation of nondeclarative memories.15,16 Another interpretation is that particular sequences of sleep states reflect the succession of brain processing events supporting memory consolidation.17 An example is the proposal that REM sleep actively consolidates or integrates complex associative information, whereas NREM sleep passively prevents retroactive interference of recently acquired complex associative information.18 In this view, SWS and REM sleep play complementary roles and have to act serially to consolidate the new memory trace, in a double-step process.19,20 Both approaches assume that it is sleep on the first posttraining night that is important for memory consolidation. Nonetheless, it would be premature to claim that only sleep may achieve the necessary conditions to consolidate novel memories in the nervous system, as both sleeplike cognitive and neural processes of memory consolidation have also been observed during wakefulness.21–23
Methods for Studying the Role of Sleep for Memory Consolidation
Posttraining Sleep Deprivation
The first and probably most ancient line of investigation has probed the putatively detrimental effect of sleep deprivation on the night after learning, based on the assumption that memory performance over the long term will be better if participants are allowed to sleep after learning rather than being deprived of sleep. In classic procedures, subjects learn new material. Afterward, some participants are allowed to sleep normally whereas others either do not sleep at all (total sleep deprivation), are awakened at the onset of occurrences of the sleep stage under study (selective sleep deprivation), are kept awake during the period of the night in which the sleep stage is predominant (partial sleep deprivation), or have a shortened sleep duration (sleep restriction). Finally, prenight and postnight memory measures are compared between sleeping and sleep-deprived subgroups, either the next day or several days later. Jenkins and Dalenbach24 used this approach about 85 years ago and found that the forgetting curve for newly learned verbal material described by the German psychologist Ebinghaus was significantly dampened by the presence of an intermediate period of sleep. Nonetheless, they did not attribute this effect to a specific role of sleep per se in memory processing but merely to the protective role that sleep may have against “interference, inhibition, or obliteration of the old by the new.”24 However, this hypothesis of a purely passive role for sleep in memory processes has been challenged by studies of selective sleep deprivation that attribute a specific role to REM sleep in memory storage and consolidation, in both human and animal species.25 Also, it was demonstrated that memory over an interval with relatively high amounts of stage 4 sleep (i.e., in the first half of the night) was superior to memory over an interval with relatively high amounts of REM sleep (i.e., in the second half of the night).26 This seemingly apparent contradiction was resolved later, with the demonstration that recall of paired-associate lists was significantly better after sleep than wakefulness in the first part of the night only, whereas consolidation of mirror-tracing skills specifically benefited from sleep in the second part of the night.15 Results showed that memories belonging to the declarative and nondeclarative systems do not benefit from the same sleep components. Other experimental manipulations inferred a specific role for stage 2 sleep in the second part of the night for the consolidation of motor memories.27,28 Hence, sleep deprivation studies have suggested that each stage of human sleep (REM sleep, SWS, stage 2 sleep) might be involved in a distinctive way in learning and memory consolidation processes.6,7
In the recent past, neuroimaging investigations have complemented these findings using functional magnetic resonance imaging (fMRI). As in behavioral paradigms, subjects are trained to the task and then either deprived of sleep or allowed to sleep on the following night. A few days later, cerebral activity is recorded during memory retrieval and the two posttraining sleep conditions are compared. One or two additional nights of regular sleep are usually allowed after the posttraining night before testing in the scanner, to avoid different arousal states that may confound neural activity associated with memory retrieval between sleeping and sleep-deprived subjects. These neuroimaging studies have yielded two important contributions. First, they have demonstrated that sleep deprivation during the posttraining night eventually impedes the reorganization and optimization of the cerebral activity, subtending delayed retrieval of consolidated memories during wakefulness.29–32 Second, they have shown that sleep-dependent changes in memory-related brain activity patterns may be present even when similarity in behavioral performance between posttraining sleep conditions suggests an absence of sleep-related effect on memory.30,32 The latter results further indicate that long-term memory performance can be achieved using different cerebral strategies initiated as a function of the status of sleep during the posttraining night.
Posttraining Sleep Modifications
The second line of investigation stemmed from the reasoning that if newly acquired information underwent an ongoing process of consolidation during sleep, then this should be reflected in neural and physiologic features of posttraining sleep. Using electroencephalographic (EEG) recording techniques, numerous animal studies have reported changes in, or memory-related associations with, a series of postlearning sleep parameters, including sleep stage duration or proportion relative to total sleep time, density of rapid eye movements, power increase in selected EEG frequency ranges, changes in density and duration of phasic events (e.g., spindles, ponto-geniculo-occipital waves), and reexpression of learning-specific neural patterns (for reviews, see references 3, 4, 6, 33, 34).
Likewise, in humans, numerous EEG studies have evidenced that both the architecture of sleep and distinctive features of sleep stages can be affected by prior learning experience. For example, postlearning sleep modifications have been shown when looking at absolute or proportional (i.e., relative to total sleep time) increases in the duration of REM sleep,6,20,35–37 stage 2 sleep38 and SWS20 episodes. Other studies have reported increased density of rapid eye movements39,40 and stage 2 spindle activity in the sigma frequency band,41–50 as well as increases in REM sleep theta power.51 Many found statistical correlations between quantitative parameters of sleep and overnight performance improvements20,37,41–44,48–50 or levels of performance at the end of learning,45 suggesting a close link between changes in sleep physiology and memory consolidation. In support of the double-step hypothesis described earlier, other studies evidenced relationships between performance changes and the organization of NREM/REM sleep cycles.19,52 Hence, these investigations, mostly based on noninvasive electrophysiologic techniques, have consistently demonstrated that physiology of sleep is influenced by prior learning during the day. Additionally, waking experience was shown to influence the content of hypnagogic hallucinations53 and of dreams collected on awakening from sleep stages54,55 on the postexposure night, although delayed incorporations of up to weeks have also been reported often.55
Noninvasive neuroimaging studies, especially using positron emission tomography (PET) measurements, with their better spatial resolution and whole brain coverage, have been used to look more precisely at the neural correlates of postlearning modifications during sleep. They have shown that neural activity occurring during waking task practice in learning-dedicated cerebral structures can be reexpressed or continued during both REM56,57 and NREM58 stages of sleep, as well as during posttraining wakefulness.21 These data were in close agreement with intracerebral recording studies in animals, which demonstrated neuronal reactivation during sleep.59,60 The animal data suggested reactivation of coherent memory traces in key cerebral structures during sleep that was deemed to contribute to or reflect the result of the memory consolidation process. However, these seminal animal studies did not experimentally establish the behavioral relevance of reactivations in neuronal ensembles, as they never sought to show a relationship with subsequent behavioral modifications. A significant contribution of human neuroimaging data was to show that experience-dependent reactivations of local—that is, hippocampal—activity during SWS are correlated to overnight gains in memory performance after spatial navigation.58 On the other hand, levels of implicit procedural learning achieved before sleep correlated with the amplitude of reactivation in cortical areas during REM sleep,57 at which time connectivity patterns between learning-related areas were additionally reinforced.61 Taken together, human reactivation studies have suggested the neuronal replay of previous experience during sleep, and that posttraining sleep activity in brain areas involved during the learning episode represents a neural signature of memory-related cognitive processes. Another (but not exclusive) hypothesis is that learning in the awake state induces local synaptic changes that themselves induce local changes in slow-wave activity (SWA), the main marker of sleep homeostasis, and that these changes are ultimately beneficial to imprint novel memories.62 In support of this view, high-density electrophysiologic recordings have demonstrated local increases in SWA in learning-related areas during posttraining NREM sleep, correlating with overnight performance improvements.63
Within-Sleep Stimulations
In line with animal studies,33 preliminary data have indicated the possibility of heart rate conditioning in man during NREM sleep,64 suggesting plasticity during sleep. On the other hand, no learning effect was found for lists of paired-associate words presented during either REM or stage 2 sleep and tested immediately afterward.65 Although these results suggest a limitation in learning capacity during sleep, there is consistent evidence that sleeping subjects remain able to detect and discriminate external sensory events as indexed by event-related auditory potentials (ERPs).66,67 Likewise, nociceptive stimulations during sleep persistently elicit evoked responses.68 ERP and fMRI data also indicated higher sensitivity to external stimuli during tonic than phasic periods of REM sleep.69,70 Higher-level processing of externally presented events was also found during sleep, as auditory ERPs are elicited after hearing one’s own name both while sleeping and awake.71 Nonetheless, brain responsiveness during sleep is not independent of prior waking experience. Learning semantic associations72 or discriminating auditory patterns67 during wakefulness leads to changes in evoked response potentials during sleep,72 even up to 2 days later.67 These results support the idea that external events can be processed during certain periods of sleep, and that stimulus significance affects this processing.
Further demonstrating an active role for sleep in memory consolidation processes, several studies have established that stimulations in the posttraining sleep period may enhance performance as compared with a standard, unmodified posttraining night.73–77 Presentation of nonawakening auditory stimulations during REM sleep after Morse code learning73 or re-presentation during REM sleep of sounds heard in background while learning a complex logic task74 increased overnight memory. This effect was present only when auditory stimulations were displayed in coincidence with the bursts of rapid eye movements that reflect phasic ponto-geniculo-occipital (PGO) activity in man. Likewise, presentation during SWS of odors that were used as contextual cues during the learning episode triggered hippocampal responses and improved overnight retention of declarative memories.77 Transcranial direct-current stimulation that modulates excitability in cortical areas improved declarative memory when applied during SWS,76 especially when application of oscillating potentials at about 0.75 Hz induced slow oscillation-like potential fields that mimic the slow oscillations of deep NREM sleep.75 Finally, artificially maintaining high levels of cortisol feedback and cholinergic tone during SWS impairs hippocampus-dependent declarative memory formation,78–80 suggesting that the natural shift in central nervous system cholinergic tone from high levels during acquisition-related wakefulness periods to minimal levels during SWS optimizes declarative memory consolidation.81 On the other hand, preventing natural increases in cortisol during REM sleep periods appears to enhance amygdala-dependent emotional memory.80 These studies have demonstrated beneficial (or detrimental) effects of various stimulations and manipulations in the posttraining sleep periods on overnight gains in performance, and therefore have provided interesting evidences that sleep does not play a merely passive role in memory processing by protecting novel memories from interference. Rather, they support the hypothesis that sleep acts in a complex manner in providing optimal conditions for the consolidation of novel memories in the nervous system.
Sleep and Declarative Memory
Semantic Memory
Few studies have looked at the role of sleep for consolidation of semantic information, although ERP studies have demonstrated that semantic processing of externally presented stimuli is possible during REM and stage 2 sleep, but not during SWS.66,71,72 Also, it has been shown that the semantic priming (i.e., the facilitatory processing effect resulting from prior presentation of semantically related material) qualitatively differs upon awakening from stage 2 and REM sleep.82 Despite evidence for residual semantic processing, attempts to create novel semantic associations using direct auditory stimulation during sleep have been unsuccessful.65 Other evidence for a role of sleep in processing newly acquired semantic memories are not fully conclusive because the tasks that have been used comprise other cognitive components that may obscure the interpretation.2 For example, improvement in a French immersion course over 6 weeks was correlated with REM sleep increases,36 but learning a novel language is a task that involves episodic memory processes as well as the creation of novel semantic associations. Likewise, learning Morse code, which was found to be associated with increases in REM sleep parameters,37 may be viewed as a specific form of cognitive procedural learning of novel associations. Also, it is believed that transfer of novel information from hippocampus-dependent episodic memory stores to neocortical, semantic, decontextualized memory representations is a gradual process that may take years to complete.8 Therefore, protocols in which initial encoding, posttraining sleep periods and retrieval are temporally close are probably not best suited to segregate the semantic component of memory from other constituents.
A few neuroimaging studies have investigated the cerebral correlates of declarative memory retrieval after extended periods of time (up to 6 months) using paired-associate list of words31 or pictures of landscapes.83 Although these experiments were not specifically designed to probe whether the memorized material was semanticized at the time of retesting, neuroimaging results clearly indicated a transfer from activity in hippocampal locations, observed early after learning, toward activity in medial prefrontal cortical (mPFC) sites recorded 6 months later during memory retrieval.31,83 Furthermore, total sleep deprivation on the postlearning night hindered this gradual process of consolidation. Six months after learning verbal material, memory retrieval more strongly activated the mPFC sites when initial encoding was followed by a night of sleep than by sleep deprivation, suggesting that sleep leads to long-lasting changes in the representation of memories at the neuroanatomic level.31 These results may be consistent with the hypothesis that sleep exerts an effect on the gradual semanticization of the learned material.
Episodic Memory
The effect of sleep on episodic memory has been extensively studied using a series of declarative memory paradigms encompassing learning of verbal material such as lists of words or paired-associate words15,31,41,42,50,51,75,76,78,79,84–89 and sentences or prose passages,90 and explicit encoding of landscapes,83 objects’ locations,77 faces,91 visuospatial memory,16,48 and navigation in virtual30,32,58,92 or natural93 environments.
Among these, most studies using partial behavioral15,16,31,85 or pharmacologic79 sleep deprivation have consistently found that SWS, or at least the first half of the night of sleep (which is rich in SWS), is beneficial for the consolidation of novel declarative memories. Consolidation of declarative learning was also linked to increased spindle activity during posttraining stage 2 sleep,41,42,48–50 as well as to the alteration of SWS94 and spindles95 in schizophrenia. Spindles are strong candidates to subtend memory consolidation processes during sleep because they are thought to support neural plasticity.96 As well, declarative learning abilities have been linked with increased spindle activity during stage 2 sleep44,97 and periodic arousal fluctuations during non-REM sleep98 in healthy subjects. On the other hand, declarative memory deficits are associated with decreased sleep spindle activity in patients with Alzheimer’s disease,99 and NREM sleep duration and number of cycles in patients with chronic nonrestorative sleep.100 However, others reported that overnight performance gains on declarative verbal memory depend primarily on preserved organization of sleep cycles—that is, sleep continuity—rather than on the integrity of a specific sleep stage per se.19 These studies may stand in contrast with much older ones that showed impairment in recall of sentences and short stories101 or lists of words102 after selective REM sleep deprivation. Also, a more recent study found that REM sleep deprivation specifically impaired recall of spatial and temporal features of memories, as well as the subject’s confidence in his own remembering,89 these parameters being considered as genuine components of episodic memory, as opposed to general recall, which may partially rely on semantic, decontextualized memories.2 Accordingly, it has been reported that consolidation during sleep enhances explicit recollection in recognition memory103 and strengthens the original temporal sequence structure for lists of triplet words.104
Emotion in Episodic Memory
The role of emotional variables in sleep-dependent processes has been a focus of recent interest.80,90,105–108 Emotion can be seen as an important contextual cue in retention of episodic memories, although it is not necessarily processed explicitly. Nonetheless, emotional material was found to be better recalled after REM than after NREM sleep90 and to be altered after sleep deprivation,105 although more for the emotional content than the context of the information.108 However, others have found emotional memories to be better preserved, or at least less disrupted, than neutral memories after total sleep deprivation.80,106 These latter results suggest that consolidation of emotional memories also occurs efficiently during awake periods, an effect that may be explained by the important ecologic value of rapidly acquiring emotional stimulus–response associations. Similarly, it was found that sleep deprivation does not alter behavioral performance in an ecologically valid virtual navigation task.30,32 Importantly, a lack of behavioral effect does not guarantee that sleep was without any consequence on memory consolidation processes, as, in the case of both navigation and emotional memories, underlying patterns of brain activity at retrieval were effectively altered by sleep deprivation on the posttraining night.30,32,106
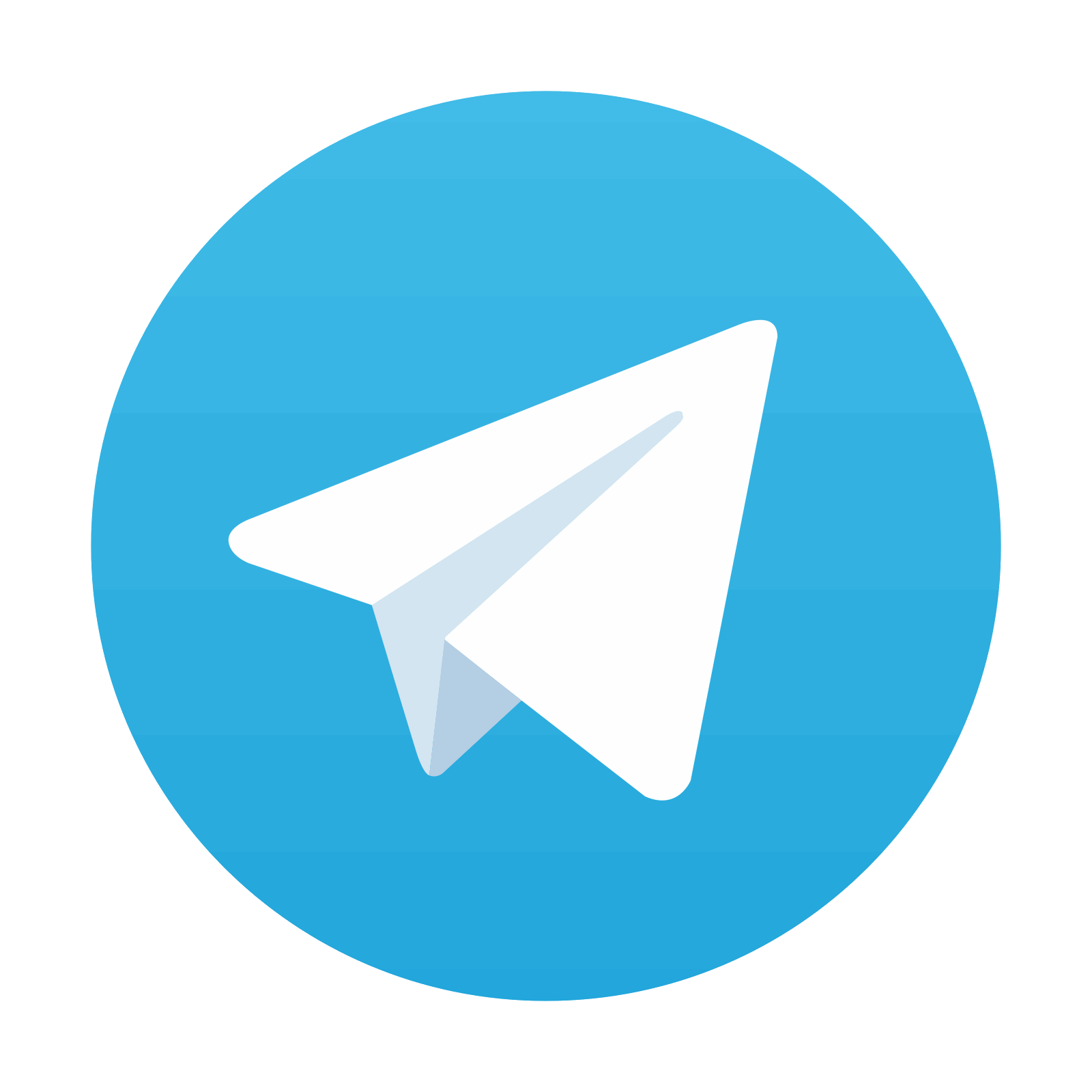
Stay updated, free articles. Join our Telegram channel

Full access? Get Clinical Tree
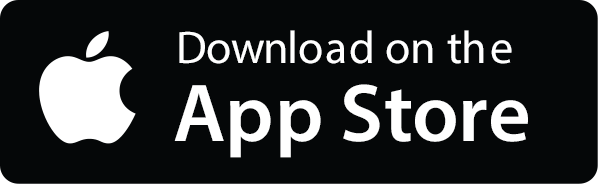
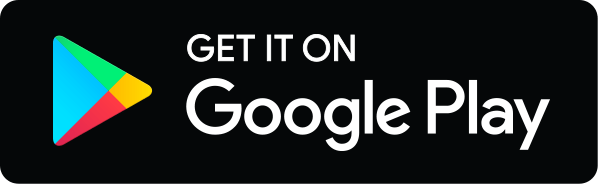