Fig. 33.1
Schematic representation of different mechanisms thought to be implicated in the pathogenesis of autism
Metabolic Insights in ASD
In 1966, Dohan postulated that schizophrenia is the cause of an overload of peptides derived from dietary gluten [14]. This hypothesis was later extended to include autism [15, 16].
The normal dietary protein digestion entails their breakdown to smaller molecules until reaching the basic unit (amino acid). Therefore, an incomplete protein breakdown generates bigger structural compounds, namely peptides, composed by 2–8 amino acids. Indeed, increased urinary β-casomorphin and gliadorphin peptides have been reported in ASD patients as a result of an incomplete digestion of casein and gluten respectively [6]. Both of these peptides show structural similarities in fragment tyrosine–proline (tyr–pro) in position 1–2 and proline (pro) amino acid in positions 4 and 6 (Fig. 33.2).


Fig. 33.2
Amino acid sequence for β-casomorphin-7 and gliadorphin-7 peptides. Amino acids: tyrosine (tyr), proline (pro), phenylalanine (phe), glycine (gly), isoleucine (ile), glutamine (gln)
Altered intestinal permeability has been implicated in ASD patients, and is thought to be the link between the gut and brain in autism pathogenesis [17–19]. Supporting this hypothesis, gastrointestinal disorders, such as diarrhea, chronic constipation, abdominal pain, ulcerative colitis, and others, have been reported in ASD patients [20–23].
ASD patients are four times more likely to suffer from gastrointestinal problems than the rest of the population [24]. This is a fact known since the first clinical description of autism in the 1940s [25]. Moreover, there is evidence of a strong correlation between gastrointestinal symptoms and autism severity that could be explained by three different mechanisms: (1) an increased permeability of the intestinal mucosa not related to inflammatory processes, such as tight junction defects or alterations in the intestinal flora composition (gut microbiome). (2) a possible second mechanism involving inadequate or deficient enzymes for either intestinal or blood breakdown of β-casomorphins or gliadorphins which allows exorphins in large amounts to gain access to the blood and circulate until taken up by the brain, (3) a possible third mechanism involving an inflammatory process causing increased intestinal wall permeability [6, 17, 18, 26] (Fig. 33.3).


Fig. 33.3
Passage of opioid peptides through gut, blood, and central nervous system (CNS). (a) Normal gut–brain transport of peptides. In healthy subjects, with no major problems with intestinal wall permeability, a portion of peptides (green image) normally crosses from the intestine into the bloodstream. A portion of these peptides bloodstream will cross through the blood–brain barrier (BBB), accessing the brain. (b) Excessive peptide production in the gut. Subjects with abnormal intestinal conditions (gut pH alterations or enzyme system deficiencies) may show increased intestinal peptide levels that will go through the bloodstream and later the BBB reaching the brain. (c) Excessive permeability of the intestinal membrane. Subjects with normal gut peptide levels with a flaw in intestinal membrane permeability. This situation allows greater amounts of biologically active peptides to enter the brain through the bloodstream
Both peptides, β-casomorphin and gliadorphin, induce direct “opioid activity” [27, 28]. The “opioid excess” theory suggests that ASD symptoms are a consequence of the action of exogenous peptides affecting neurotransmission within the central nervous system (CNS) [15, 29–32].
In the absence of an established pathogenesis for autism, some authors have proposed explanations and treatments based on diet and nutrients. Reports on the use of a gluten-and casein-free diet have shown improvement of ASD symptoms, such as social isolation, and overall ability to communicate and interact [33]. Moreover, Cade et al. showed that a gluten- and casein-free diet was accompanied by improvement in 81% of autistic children within 3 months in most of the behavior categories [6]. Other researchers have shown similar results [34–39].
Genetic Insights in ASD
Direct Brain Impacts
Genetic heterogeneity and gene–environment interactions have been found in patients with ASD. Many ASD risk genes encode regulatory proteins of the glutamatergic system involved in the synaptic process and excitatory/inhibitory brain networks, namely: NRXN1, PTEN, SHANK3, UBE3a, NF1, NLGN3/4, CNTNAP2, SYNGAP1, and FMR1 [40–48]. Mutations in these genes during CNS development trigger disruptions in excitatory/inhibitory brain circuits [45, 47, 48].
Actually, before synaptogenesis and brain network formation, critical developmental events such as neurogenesis, migration, cellular differentiation, and polarization take place [49, 50]. Therefore, alterations in genes involved in these early stages of CNS development may contribute to a serious damage in brain function, particularly in relation to genes responsible for posterior synaptic function [47, 51].
The International Molecular Genetic Study of Autism Consortium (IMGSAC) was the first to identify a genome linkage with the autism susceptibility locus1 (AUTS1) on the chromosome 7q region [52, 53]. Moreover, evidence of genetic association with autism has been reported for genes located in three different regions of chromosome 7q; 7q21 (RELN, SERPINE1), 7q31 (MET), and 7q35–36 (CNTNAP2, EN2). Each of these chromosome 7q genes, and possibly others, may contribute to autism risk [43, 54–56].
The human gene MET (proto-oncogene hepatocyte growth factor receptor, OMIM 164860) that is located on chromosome 7q31.2 and covers approximately 126 kb, encodes a high-affinity transmembrane receptor tyrosine kinase that binds to the hepatocyte growth factor (HGF) [57, 58]. Protein receptor tyrosine kinases (RTKs) are cell-membrane receptors that participate in key stages of CNS development, such as neurogenesis, neuronal differentiation, migration, connectivity, and plasticity [59].
Different studies have reported this gene as a risk factor for ASD based on genetic variants overrepresented in individuals with ASD compared with control populations [60–64].
Furthermore, the expression of the MET receptor and HGF protein was reduced in post-mortem brains of individuals with ASD [65]. MET and HGF have been involved in neuronal development and maturation of functional circuits, particularly in cerebral cortex, cerebellum, and hippocampus [59, 66–68]. With distinct spatial and temporal profiles, these brain regions may be altered in autism [69, 70]. Cellular signaling through the MET receptor contributes to neuronal migration and synaptogenesis, among other developmental processes. Therefore, alterations in MET/HGF would stimulate disrupted behavioral and cognitive functions in ASD [59].
Interestingly, MET also contributes to gastrointestinal and immunological functions [71–74]. Alterations in both systems frequently co-occur in ASD patients [18, 19, 22, 60].
Several studies have found a relationship between MET promoter variants and the risk for developing ASD. Furthermore, functional imaging and animal studies tie alterations in MET gene to morphological and functional disruptions in brain regions related with ASD [59, 75].
MET gene polymorphisms “rs1858830” and “rs38845” have frequently been found in individuals with ASD in Europe and USA, and may contribute to ASD susceptibility [63, 76]. MET gene promoter “rs1858830” is a common guanine (G) to cytosine (C) single nucleotide polymorphism, with significant association in ASD families reported in Italian and USA cohorts. The “C allele” is more common in individuals with ASD than in the general population [59]. Expression studies have shown that “C allele” results in decreased MET promoter activity, thus reducing transcription of the MET gene as well as the specific transcription factor complexes binding “SP1” [60]. The functional ASD risk variant rs1858830 (“C allele”), which reduces MET protein expression, specifically impacts on the network of connections of different areas of the brain involved in social behavior, including recognizing emotions shown on people’s faces [75]. Moreover, rs1858830 has shown diminished functional and structural connectivity in temporo-parietal lobes brain areas that present a large MET expression, which leads to decreased MET protein in the brains of individuals with ASD [59, 75].
Sousa et al. described an association of another polymorphism located in intron 1 of MET gene “rs38845” with a potential risk of autism susceptibility [63]. These authors suggested that rs38845 “A allele” may regulate gene expression interfering in the transcription of MET gene by a transcriptional activator “IRF1”. Thereby, IRF1 would bind to MET promoter, thus altering MET/HGF signaling in ASD patients [63].
Gastrointestinal Impacts
As stated before, high rates of gastrointestinal conditions in individuals with ASD have been reported in several studies [77, 78]. MET gene polymorphism rs1858830 “C allele” has been associated with ASD patients with gastrointestinal dysfunctions. Moreover, the transmission of the “C allele” might be enhanced in families with co-occurring ASD and gastrointestinal conditions [60].
MET signaling system alteration can lead to brain and gastrointestinal dysfunctions, and would explain the pathophysiology of ASD and GI comorbidities [60].
Immune Dysregulation and Neuroinflammation in ASD
There is strong evidence of an association between immune dysfunction with ASD development and behavioral symptoms severity, because there are critical interactions between CNS and the immune system [79, 80]. Patients with ASD often exhibit alterations in cytokine levels, lymphocytes T helper type 2 (Th2), abnormal immune cell function, mast cell activation, and the presence of autoantibodies, supporting the association between chronic inflammation and immune dysregulation [7, 79].
General alterations of immune mechanisms trigger chronic inflammation and immune dysregulation in CNS, leading to a neuroinflammatory response [79–81].
Several in-vivo and postmortem studies have found chronic inflammatory processes in multiple areas of the brain, highlighting the correlation between immune dysfunction with behavioral and cognitive impairments in ASD patients [8, 82, 83]. Moreover, some studies have shown immune system dysregulation during a critical period of development in children with ASD [79]. These findings suggested that inflammation plays an important role in the pathogenesis of ASD, and lead to research to elucidate innate immunity pathways associated with neuronal activity in ASD [82, 84].
Association Between Allergies and ASD
ASD shares with sufferers from IgE and non-IgE-mediated allergic reactions some neuro-psychiatric symptoms and mood disorders, such as anxiety, hyperactivity, irritability, tics, sleep disturbance, incoordination, and learning disabilities that ameliorate after anti-allergic treatments. Allergic diseases are associated with pain and discomfort that exacerbate behavioral symptoms in ASD patients [90, 91].
Idiopathic ASD patients frequently show increased mast cell activation in many body organs, or “mastocytosis” [92]. Mast cell stimulation may be triggered by stress, environment, immune reaction, and toxics impacting on brain areas associated with behavior and language [93]. Mast cells release pro-inflammatory molecules and histamine that stimulate the hypothalamus–pituitary–adrenal and sympathetic axes, influencing behavior and cognition [94–96].
Non-celiac Food Sensitivity and ASD
Non-celiac gluten sensitivity (NCGS) is a heterogeneous condition regarded as a distinct clinical entity, unrelated to celiac disease, and whose symptoms are triggered by gluten ingestion in the absence of celiac-specific antibodies and of classical celiac villous atrophy [98, 99]. NCGS is an immune reaction against gluten in subjects not affected with celiac disease (CD) or wheat allergy (WA). It does not involve autoimmune mechanisms. In fact, this entity is characterized by intestinal and extra-intestinal symptoms triggered by the ingestion of gluten-containing food [99, 100].
There is strong evidence of comorbidity in ASD with intestinal pathology, and susceptibility to suffer from allergies [101, 102]. IgA antibodies to gluten and casein were found in ASD patients, suggesting a possible mechanism for the increased intestinal permeability and for some of the symptoms in ASD patients. The presence of IgG antibodies to gluten and casein is another indication that the foreign peptides reach the blood stream intact and in sufficient amounts to stimulate a vigorous IgG response, and are therefore available for uptake by the brain [6, 103]. Several studies have described clinical, serological, and histological characteristics with eosinophil infiltration in the duodenal and colon mucosa in NCGS [103]. Prominent mucosal eosinophil infiltration and increased prevalence of blood eosinophilia have been found in a high percentage of children with ASD. These features are improved in children following a gluten-free diet [104, 105].
Identification and specific medical treatment of GI pathologies in patients with ASD could diminish discomfort, thereby improving behavior problems [105].
Neuroanatomy and Brain Chemistry in ASD
Magnetic resonance images and brain scan studies in ASD patients have shown alterations in brain anatomy and white matter connections in the frontal lobe, the arcuate bundle, temporal cortices, amygdala, and the anterior cingulate cortex. Moreover, post-mortem studies of ASD patient’s brains have shown abnormal number of Purkinje cells in cerebellum, as well as dendritic arborization in hippocampus [106]. These brain regions are involved in language and social interaction skills, attention control, empathy, motivation, emotions, error-monitoring, pain perception, behavioral adaptation to a changing environment, and consciousness dissociation. All these facts correlate with specific aspects of ASD symptoms [107–110].
Opioid-active peptides have shown high affinity for the same brain tissues as other related compounds, such as endorphins or morphine [110, 111]. Thus, opioid peptides may cause excitatory and inhibitory actions in CNS, modulating presynaptic release of neurotransmitters. This is associated with the excitatory/inhibitory imbalance hypothesis (neuronal homeostasis) in ASD [112]. These peptides are involved directly or indirectly, firing neural action potentials, and/or releasing intracellular calcium [113–116]. Opioid peptides can inhibit glutamatergic (excitatory) signaling at spinal and supraspinal level, or disinhibit GABAergic signaling (inhibition) [116]. Opioid-receptor binding is involved in cellular proliferation, migration, and differentiation during CNS development [117, 118].
There are three main classes of opioid receptors—mu, delta, and kappa—which belong to the G protein-coupled family. These opioid receptors exhibit different functions and binding characteristics. A specific opioid peptide is able to bind with more than one type of receptor [119, 120]. The binding of opioid peptides to these receptors leads to analgesia and euphoric response [119].
Beta casomorphine-7 (BCM-7) interacts with opioid receptors and affects brain regions including the nucleus accumbens, caudate–putamen, ventral tegmental and median raphe nucleus, and orbitofrontal, prefrontal, parietal, temporal, occipital, and entorhinal cortex. Most of these brain areas have been found to be altered in ASD. Some of these brain regions are integrated by dopaminergic, serotoninergic, and GABA-ergic systems, suggesting that BCM-7 may interfere with all of these pathways. BCM-7 may disturb cortical association or functional connections, and could be implicated in emotions and motivated behavior, social adaptation, hallucinations, and delusions, typical manifestations of patients with ASD [28, 112, 121].
In brief, ASD behavior results from abnormal interactions between the endogenous opioid system and various pathways, together with anatomical alterations in the CNS. Biochemical, molecular, neurophysiological, and neuroimaging studies should provide further insights into the pathogenesis of ASD. Due to etiologic and phenotypic heterogeneity of ASD, individualized diagnosis and prognostic predictions should provide effective personalized therapies in ASD patients [109, 122, 123].
References
1.
Lord C, Risi S, Lambrecht L, Cook Jr EH, Leventhal BL, DiLavore PC, Pickles A, Rutter M. The autism diagnostic observation schedule-generic: a standard measure of social and communication deficits associated with the spectrum of autism. J Autism Dev Disord. 2000;30(3):205–23.PubMed
2.
Christensen DL, Baio J, Van Naarden BK, Bilder D, Charles J, Constantino JN, Daniels J, Durkin MS, Fitzgerald RT, Kurzius-Spencer M, Lee LC, Pettygrove S, Robinson C, Schulz E, Wells C, Wingate MS, Zahorodny W, Yeargin-Allsopp M. Centers for disease control and prevention (CDC). Prevalence and characteristics of autism spectrum disorder among children aged 8 years-Autism and Developmental isabilities onitoring Network, 11 sites, United States, 2012. MMWR Surveill Summ. 2016;65(3):1–23.PubMed
3.
Volkmar F, Chawarska K, Klin A. Autism in infancy and early childhood. Annu Rev Psychol. 2005;56:315–36.PubMed
4.
Bailey A, Le Couteur A, Gottesman I, Bolton P, Simonoff E, Yuzda E, Rutter M. Autism as a strongly genetic disorder: evidence from a British twin study. Psychol Med. 1995;25:63–77.PubMed
5.
Grafodatskaya D, Chung B, Szatmari P, Weksberg R. Autism spectrum disorders and epigenetics. J Am Acad Child Adolesc Psychiatry. 2010;49:794–809.PubMed
6.
Cade R, Private FM, Rowland N, Sun Z, Zele V, Wagemaker H, Edelstein C. Autism and schizophrenia: intestinal disorders. Nutr Neurosci. 2000;3:57–72.PubMed
7.
Molloy CA, Morrow AL, Meinzen-Derr J, Schleifer K, Dienger K, Manning-Courtney P, Altaye M, Wills-Karp M. Elevated cytokine levels in children with autism spectrum disorder. J Neuroimmunol. 2006;172(1):198–205.PubMed
8.
Morgan JT, Chana G, Pardo CA, Achim C, Semendeferi K, Buckwalter J, Courchesne E, Everall IP. Microglial activation and increased microglial density observed in the dorsolateral prefrontal cortex in autism. Biol Psychiatry. 2010;68(4):368–76.PubMed
9.
Williams BL, Hornig M, Parekh T, Lipkin WI. Application of novel PCR based methods for detection, quantitation, and phylogenetic characterization of Sutterella species in intestinal biopsy samples from children with autism and gastrointestinal disturbances. MBio. 2012;3(1):e00261–11.PubMedPubMedCentral
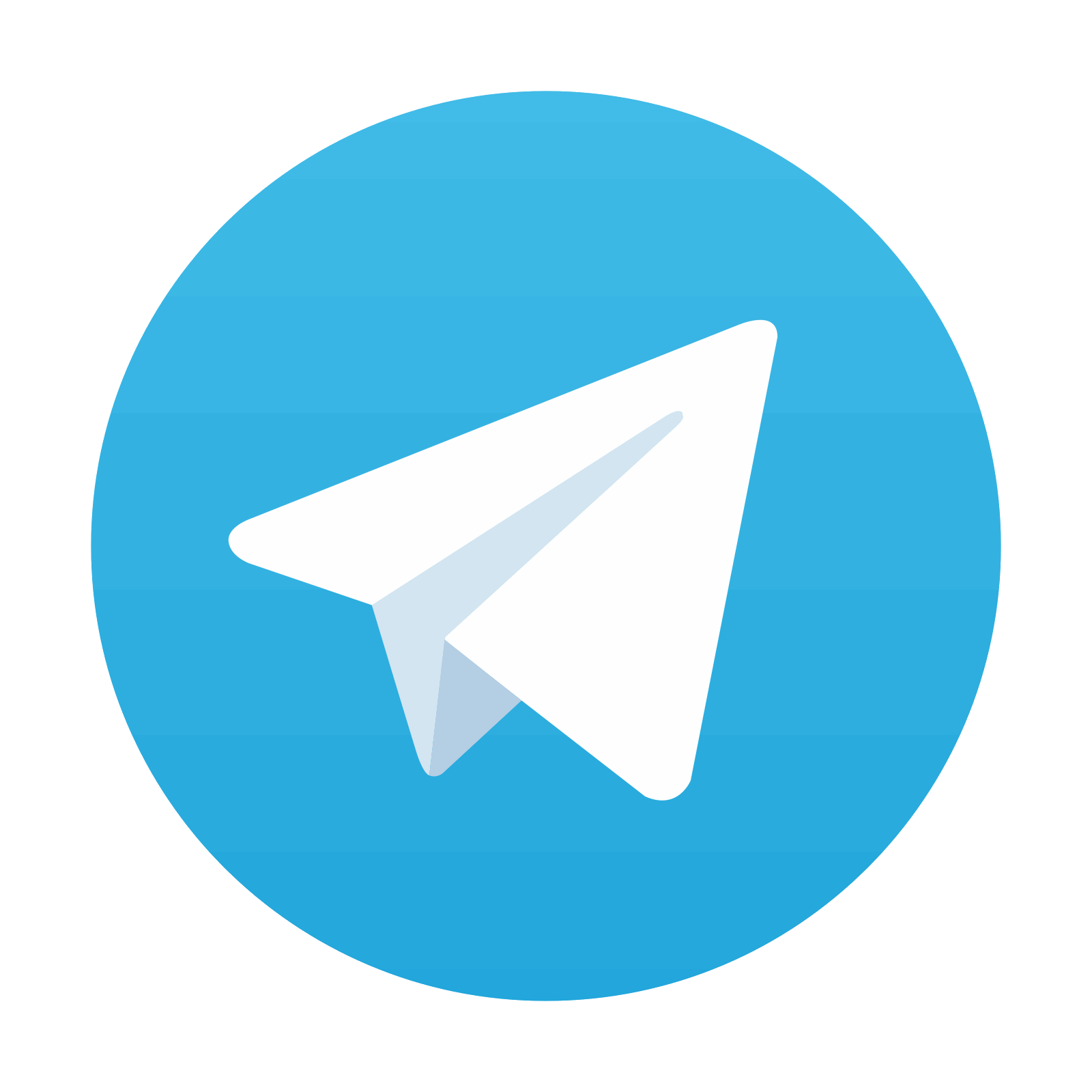
Stay updated, free articles. Join our Telegram channel

Full access? Get Clinical Tree
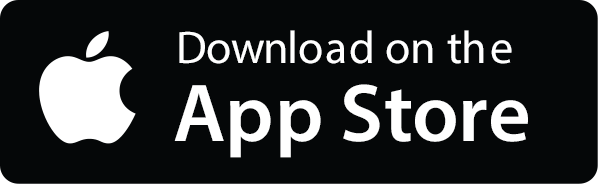
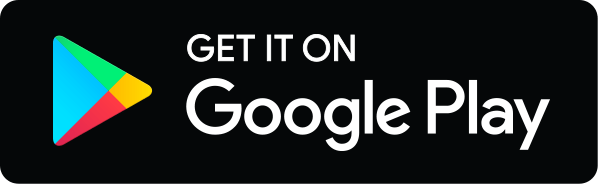