Scanning electron microscopy of normal rat brains revealed a regular pattern of blood vessels. In the normal cortex, radially arranged penetrating arterioles and venules, were interconnected by an extensive microvascular network. The figure shows images 3 days after rat middle cerebral artery occlusion, the first vascular budding was visible at many sites. Angiogenesis was also observed in larger microvessels (50–150 μm). The smallest microvessels (4–10 μm) formed connections with the surrounding proliferating vessels
The formation of new blood vessels involves a highly ordered cascade of events that is regulated all or in part by angiogenic factors as well as many mediators and molecules of the innate and adaptive immune systems, in a response of leucocytes in chronic inflammation [2]. Hypoxia plays a role in angiogenesis through stimulus promotion with the growth factors (e.g., hypoxia-inducible transcription factor-1 (HIF-1) and vascular endothelial growth factor (VEGF)). The effect of angiogenesis is to restore the circulation reduced by the ischemic event [3]. Therapies to promote post-ischemic angiogenesis are critically important for the treatment of ischemic stroke [6].
According to Yin et al., several studies in patients with stroke demonstrated that angiogenesis occurs within 3–4 days after stroke and in post-mortem examinations. The brain tissues revealed that patients that suffered from stroke had increased levels of cerebral microvessels in the penumbral areas [6]. However, in some cases, angiogenesis can cause abnormal proliferation of structurally weak and leaky blood vessels leading to undesirable complications of the inflammatory processes [4]. Hypoxia also contributes to the abnormal process when immature neovessels are deprived of oxygen, and develop weak structures with smooth muscle cells [4]. Figure 1 shows Scanning electron micrographs of vascular casts show marked sprouting and anastomoses of two newly formed capillaries within days following blockage of blood vessel in the cerebral grey matter adjacent to ischemic infarction in a rat brain.
Understanding the differences within the extracellular matrix microenvironment that accounts for patent and nonpatent neovessel regeneration has important therapeutic implications.
2 Laser Capture Microdissection (LCM)
Laser capture microdissection (LCM) is a new, easy-to-use technique recently developed to harvest specific regions or cells of interest from complex, heterogeneous tissue samples that contain a mixture of cell populations at molecular level. Prior to the advent of this technology, comparison of sampling involved heterogeneous comparisons between core biopsies usually separated by scalpel which were unable to take into account absolute cellular content and type; hence, the ultimate detail gleaned from global or targeted gene analysis assays was flawed.
The LCM system is made up of an inverted microscope, an infrared laser and a laser control unit, a joy-stick controllable stage, a charge-coupled device (CCD) camera, and a monitor. It is based on the adherence of selected cells and focally melting the captured pathologic lesions by triggering of a quite low energy infrared laser pulse without destroying the unselected tissue area. This causes melting at a precise area of a thermoplastic membrane located between the laser and the sample. The melted membrane forms a composite with the selected tissue area, which can be removed easily by simply lifting the membrane.
The membrane rapidly resolidifies and forms a composite with the targeted cells. The adherence of these cells to the membrane allows for their subsequent removal from the rest of the tissue. LCM can also be used to extract DNA, RNA and proteins from the targeted tissue fragment. Most of the energy from the laser is absorbed by the membrane, so there is minimal damage to the tissue [7].
In circumstances of disease within a tissue, the abnormal cells may be surrounded by many other tissue elements, LCM can be applied to the cells of interest only at the precise level. Using LCM to isolate these cells allows for a sufficient number to be collected and to be molecularly analyzed without interruption of the other cell populations affecting results.
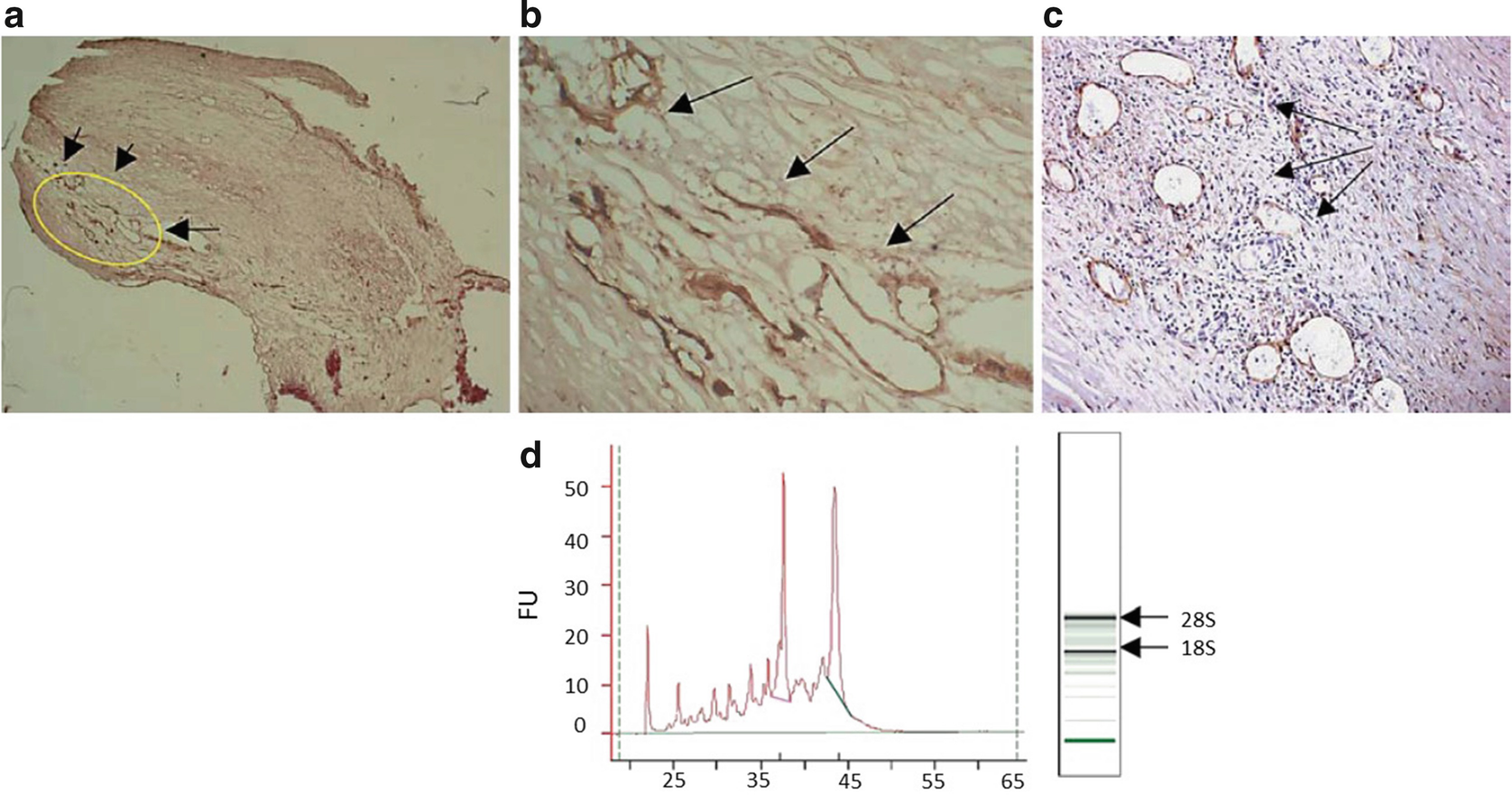
Immunostaining, identification, and separation of angiogenic microvessels. (a) Low-power photomicrograph showing an area of neointima with a high concentration of CD105-positive blood vessels [taken from endarterectomy specimen 305 (age 53 and male with a complex, ulcerated plaque; online version: yellow insert); arrows; ×10]. (b) Higher power of the same sample showing a clearly labelled, compact group of blood vessels (arrows; ×100). (c) Example of CD105-positive immature and irregularly shaped vessels chosen as positive sample areas. (d) Bioanalysis of laser-cut areas was carried out using an Agilent Technologies 2100 analyzer and only samples without evidence of RNA degradation and RIN ≥ 6.0 were chosen for preamplification. Arrows and peaks show 18S and 28S ribosomal RNA. Figure taken from Slevin et al. (J Vasc Res. 2010;47(4):323–335) with agreement of Karger Publishers
An example is shown in Fig. 2, where angiogenic microvessels in close proximity to inactive ones/areas without vessels are shown in a human heterogeneous carotid plaque (a and magnified in b). Also shown is a cluster of CD105-active-positive microvessels and a demonstration in one section of a region containing both active and quiescent vessels (c).
LCM is significantly faster, more precise, and more versatile compared to previous microdissection methods [7]. Because LCM does not destroy the adjacent residual tissue, it allows multiple different tissue components to be taken from the same tissue lesion and compared using a battery of genetic, epigenetic, and even protein profiling systems. Our colleagues and ourselves have used LCM for examining gene expression patterns in heterogeneous plaque microenvironments in carotid and coronary arteries, for investigation of neuronal health and function following ischemic stroke, and also, comparing neurodegenerative (dementia) regions versus normal looking areas of the brain. All appropriately prepared histological cryo-tissue could theoretically be used in other disease processes for example in targeting the moment a cancer cell undergoes epithelial–mesenchymal transition and the capability to metastasize.
3 Detailed Methodology1
3.1 List of Consumables and Equipment
Tissue of interest |
ddH2O (DEPC treated) |
Ethanol 70% and 100% (RNase Free) |
RNase Zap decontamination solution (Fisher) |
Cryostat mounting medium (OCT) |
Polyethylene naphthalate (PEN) Membrane Frame Slides (fisher) |
Superfrost Plus slides |
Microcentrifuge tubes (RNase free) |
RNase Zap decontamination solution (Fisher) |
ABC elite kit (Vector) |
DAB substrate kit (Vector) |
CD105 Antibody |
CD31 Antibody |
Vector Hematoxylin QS (Vector) |
Robot microbeam laser microscope |
Total RNA Purification Kit (Norgen 48300) |
β-Mercaptoethanol |
Norgen Total RNA purification kit (reference) |
Qubit Fluorometer (Life Technologies) |
Qubit RNA HS Assay Kit (Fisher) |
Bioanalyzer (Agilent) |
Agilent RNA 6000 Pico Kit (Agilent) |
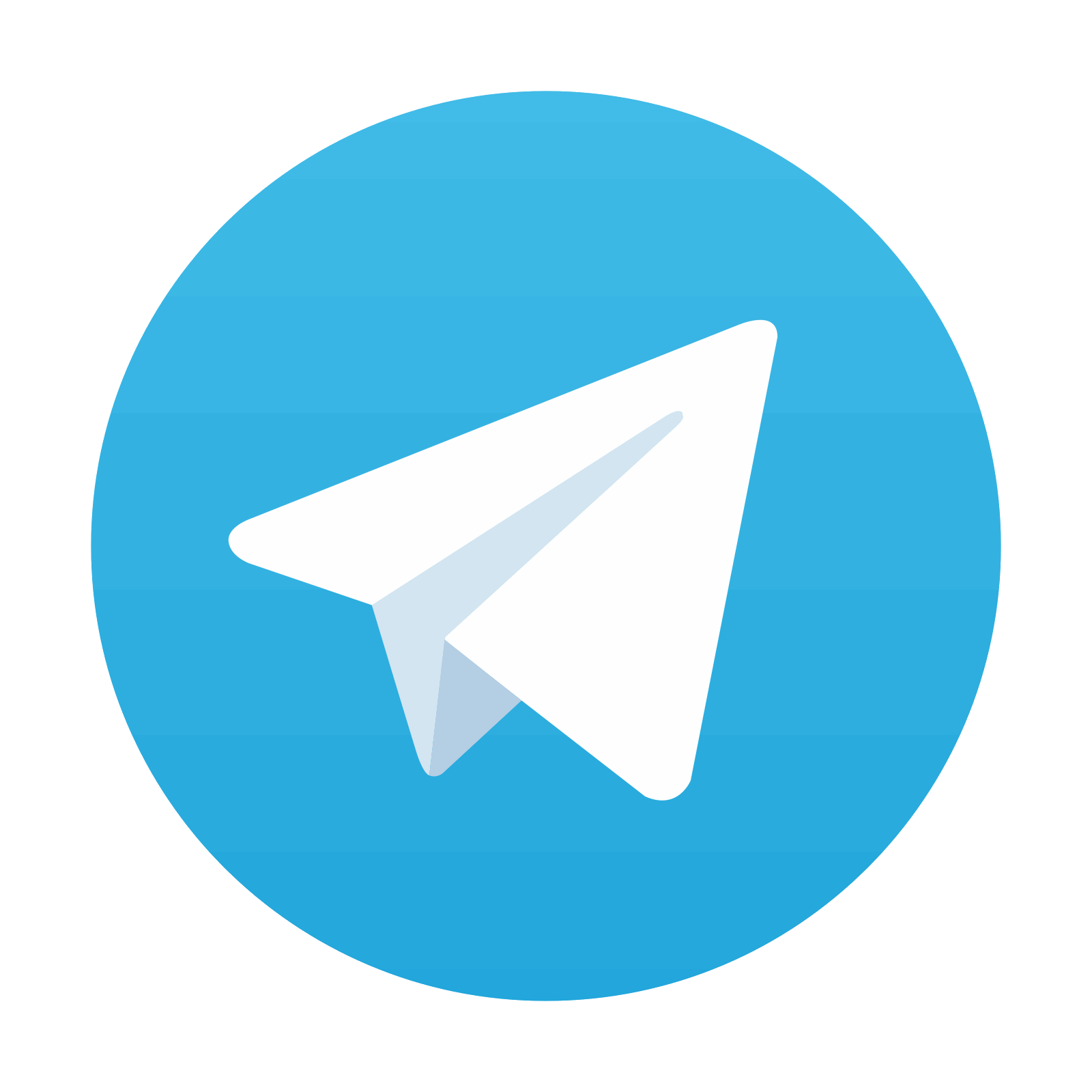
Stay updated, free articles. Join our Telegram channel

Full access? Get Clinical Tree
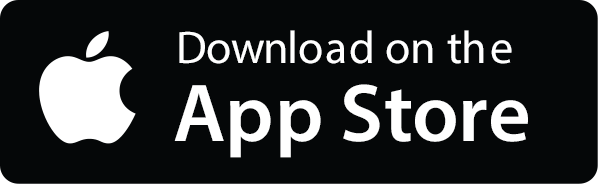
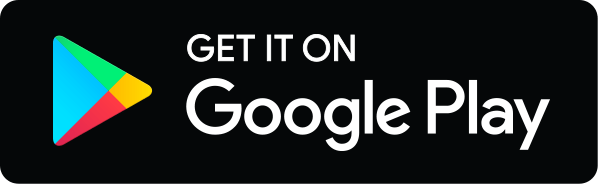