Mitochondrial Diseases
Michio Hirano
Wolfram S. Kunz
Salvatore DiMauro
Introduction
Since the initial discovery of mitochondrial DNA mutations in 1988, our understanding of the mitochondrial encephalomyopathies has advanced at an astoundingly rapid pace. Numerous scientific and clinical reports have documented the discoveries, while review articles19,23 and even several books20,104 have summarized much of this progress. The purpose of this chapter is not to describe the mitochondrial encephalomyopathies comprehensively, but rather, to illustrate some of the fundamental clinical and scientific themes in these diverse disorders that often manifest epilepsy.
Mitochondria are vital organelles, because they (a) produce energy in the form of adenosine triphosphate (ATP) through oxidative-phosphorylation of carbohydrates, fats, and amino acids,57,70,128 (b) are essential for intracellular calcium homeostasis,28 (c) contribute to the synthesis of important metabolic precursors [e.g., uridine,54 amino acids, and iron-sulfur (Fe-S) clusters34], and (d) finally, participate in cell death pathways.39 Much has been learned about the functions of these organelles, and defects of specific processes provide an elegant and rational biochemical classification system of the mitochondrial diseases (Table 1). A prime example of a specific biochemical defect of mitochondria is carnitine palmityl transferase (CPT) II deficiency.21 Transport of fatty acids through the mitochondrial membranes is impaired by specific mutations in CPT II. By contrast, mitochondrial respiratory chain disorders often do not conform to single-enzyme defects because multiple enzymes can be affected; therefore, this biochemical classification scheme has limitations.
Molecular genetics provides an alternative perspective of mitochondrial diseases. One can gain a better understanding of mitochondrial disorders by considering the several unique genetic characteristics of mitochondria. Mitochondria are unique mammalian organelles because they possess their own genetic material, mitochondrial DNA (mtDNA), which is a small (16.5 kilobases) circular molecule encoding 13 polypeptides, 22 transfer RNAs (tRNA), and two ribosomal RNAs (rRNA).4 The mtDNA-encoded polypeptides are vital subunits of the respiratory chain. In addition, more than 1,000 mitochondrial proteins are encoded in the nuclear DNA (nDNA). Because mitochondria are the products of two genomes, defects in either genome can cause mitochondrial dysfunction. To date, most of the respiratory chain defects that have been characterized at the molecular genetic level are due to mtDNA mutations.19,20,23
An important principle of mtDNA genetics is heteroplasmy. Each mitochondrion contains two to ten copies of mtDNA and, in turn, each cell contains multiple mitochondria; therefore, there are hundreds to thousands of mtDNA molecules in each cell. Alterations of mtDNA may be present in some of the mtDNAs (heteroplasmy) or in all of the molecules (homoplasmy). As a consequence of heteroplasmy, the proportion of a deleterious mtDNA mutation can vary widely. An individual who harbors a large proportion of mutant mtDNA will be more severely afflicted by the mitochondrial dysfunction than a person with a low percentage of the same mutation. As a consequence, a given mtDNA mutation can produce a diverse spectrum of clinical severity among patients.
A second factor that can influence the expression of a mtDNA mutation in a person is the tissue distribution of that mutation. The best example of tissue distribution variation is offered by large-scale mtDNA deletions. Infants with a high proportion of deleted mtDNA in their blood can develop Pearson syndrome, a sideroblastic anemia often accompanied by exocrine pancreatic dysfunction.68,92 Presumably, these infants have a high proportion of deleted mtDNA in the bone marrow stem cells. Some children survive the anemia with blood transfusions and subsequently recover, because the stem cells with a high proportion of deleted mtDNA are eliminated through a negative selection bias. Later in life, however, those children may develop the multisystem mitochondrial disorder Kearns-Sayre syndrome (KSS), characterized by ophthalmoplegia, pigmentary retinopathy, and cardiac conduction block.53,93 Thus, variable tissue distribution broadens the clinical spectrum of pathogenic mtDNA mutations.
The third factor that determines clinical expression of a mtDNA mutation is tissue threshold effect. Cells with high metabolic activities are more severely affected by relatively lower levels of mtDNA mutations; therefore, these disorders tend to affect disproportionately the brain and muscles (encephalomyopathies).
A fourth unusual characteristic of mtDNA is maternal inheritance. During the formation of the zygote, the mtDNA is derived exclusively from the oocyte. Thus, mtDNA is transmitted vertically in a nonmendelian fashion from the mother to both male and female progeny. A single exception to this rule has been described; a man with exercise intolerance and two base-pair deletions in the mitochondrial-encoded gene for subunit 2 of complex I (nicotinamide adenine dinucleotide [NAD] dehydrogenase 2 [ND2]) showed paternal inheritance of mtDNA in skeletal muscle.56,108 Because paternal inheritance of mtDNA appears to be exceedingly rare,29,109,122 maternal inheritance is important to recognize in determining whether a family is likely to harbor a mtDNA mutation. A caveat to this principle is the fact that maternal relatives who have lower percentages of a mtDNA mutation may have fewer symptoms (oligosymptomatic) than the proband, or they may even be asymptomatic. Therefore, in taking the family history, it is important to ask about the presence of subtle symptoms or signs in maternally related family members who might be oligosymptomatic.
Table 1 Biochemical classification of mitochondrial encephalomyopathies | ||
---|---|---|
|
These peculiar features of “mitochondrial genetics” contribute to the clinical complexity of human mitochondrial
disorders. Variable heteroplasmy of mtDNA mutations produces an extensive range of disease severity, whereas tissue distribution and tissue threshold of mtDNA mutations explain the frequent but variable involvement of multiple organ systems.
disorders. Variable heteroplasmy of mtDNA mutations produces an extensive range of disease severity, whereas tissue distribution and tissue threshold of mtDNA mutations explain the frequent but variable involvement of multiple organ systems.
In addition to mtDNA mutations, nuclear DNA (nDNA) defects can also cause mitochondrial dysfunction. In fact, nDNA encodes most of the components of the respiratory chain and, since 1995, many nDNA mutations associated with defects in oxidative-phosphorylation have been identified.19,20 In this chapter, we review the major mitochondrial encephalomyopathy syndromes associated with seizures. We will not quote or summarize the vast literature on mitochondrial encephalomyopathies; rather, we will try to convey some of the principles of evaluation, diagnosis, and treatment of these disorders with emphasis on epilepsy.
Clinical Description
The mitochondrial encephalomyopathies comprise a heterogenous group of multisystem disorders that typically affect infants, children, or young adults. Among these diverse conditions, specific clinical syndromes were recognized. Because clinicians are confronted with patients, the clinical classification of the mitochondrial disorders is of pragmatic significance in guiding the diagnostic evaluation, determining prognosis, and in directing therapy. The discovery of distinct mtDNA and nDNA mutations has demonstrated that, in general, clinical phenotypes have specific genotypes; however, some patients do not fit into any clinical syndrome, or they have an atypical presentation for a particular mutation.
Table 2 Seizure incidence in specific mitochondrial encephalomyopathy syndromes | ||||||||||||||||||||
---|---|---|---|---|---|---|---|---|---|---|---|---|---|---|---|---|---|---|---|---|
|
Common to all mitochondrial disorders with prominent epilepsy is involvement of the central nervous system (CNS), particularly the cortex. Imaging techniques have confirmed that gray matter involvement is an early feature of myoclonus epilepsy with ragged-red fibers (MERRF) and of mitochondrial encephalomyopathy, lactic acidosis, and stroke-like episodes (MELAS); white matter abnormalities are also seen at later stages, but usually not in isolation.15 Maternal inheritance is pathognomonic of mtDNA point mutations, whereas patients with single large-scale rearrangements tend to be sporadic. In addition, phenotypic variability in families is the general rule rather than the exception with pathogenic mtDNA point mutations.
Mitochondrial Encephalomyopathies Associated with mtDNA Mutations
Kearns-Sayre Syndrome
Rowland and colleagues defined Kearns-Sayre syndrome (KSS) by the obligate triad of ophthalmoplegia, pigmentary retinopathy, and onset before age 20, with at least one of the following additional features: Cardiac conduction block, ataxia, and cerebrospinal fluid (CSF) levels of greater than 100 mg/dL.93 The existence of KSS as a clinical entity is supported by the fact that more than 150 patients with these characteristics have been reported.43 About 90% of the KSS patients have single large-scale rearrangements of the mtDNA, either deletions, duplications, or both.72,85 Typically, KSS patients are sporadic, because the mtDNA rearrangements seem to originate in oogenesis or early zygote formation. Despite the clear evidence of encephalopathy (dementia, basal ganglia calcifications, and spongy changes of the brain white matter), seizures are uncommon in KSS (5/156 patients) (Table 2).43
Myoclonus Epilepsy with Ragged-Red Fibers Syndrome
In contrast to KSS, MERRF includes epilepsy as a defining clinical feature. In addition, these patients have myoclonus, ataxia, and ragged-red fibers in the muscle biopsy.32 Other common clinical manifestations associated with MERRF are hearing loss, dementia, peripheral neuropathy, short stature, exercise intolerance, lipomas, and lactic acidosis.118 The majority of MERRF patients have a history of affected maternally related family members, although not all have the full syndrome.
In 1990, Shoffner et al. identified a mtDNA A-to-G transition mutation at nucleotide (nt) 8344 of the tRNALys gene113; that mutation has been found in about 90% of MERRF patients tested.118 This mutation was the first molecular genetic defect to be associated with a hereditary epilepsy syndrome. A second mutation in the same gene at nt-8356 was identified in a pedigree with typical MERRF117 and in another family with overlap features of MERRF and MELAS.134 In addition, a mutation at nt-611 in the tRNAPhe gene has
been also associated with MERRF.63 Within families with a MERRF proband, oligosymptomatic and asymptomatic members harbor the same mtDNA mutation, but the phenotype is presumably attenuated by heteroplasmy and tissue distribution of the mtDNA mutation.118
been also associated with MERRF.63 Within families with a MERRF proband, oligosymptomatic and asymptomatic members harbor the same mtDNA mutation, but the phenotype is presumably attenuated by heteroplasmy and tissue distribution of the mtDNA mutation.118
Table 3 MtDNA mutations associated with epilepsy | |||||||||||||||||||||||||||||||||||||||||||||||||||||||||||||||||||||||||||||||||||||||||||||||||||||||||||||||||||||||||||||||||||||||||||||||||||||||||||||||||
---|---|---|---|---|---|---|---|---|---|---|---|---|---|---|---|---|---|---|---|---|---|---|---|---|---|---|---|---|---|---|---|---|---|---|---|---|---|---|---|---|---|---|---|---|---|---|---|---|---|---|---|---|---|---|---|---|---|---|---|---|---|---|---|---|---|---|---|---|---|---|---|---|---|---|---|---|---|---|---|---|---|---|---|---|---|---|---|---|---|---|---|---|---|---|---|---|---|---|---|---|---|---|---|---|---|---|---|---|---|---|---|---|---|---|---|---|---|---|---|---|---|---|---|---|---|---|---|---|---|---|---|---|---|---|---|---|---|---|---|---|---|---|---|---|---|---|---|---|---|---|---|---|---|---|---|---|---|---|---|---|---|
|
Table 4 Mitochondrial encephalomyopathies with epilepsy due to nuclear DNA mutations | ||||||||||||||||||||||||||||||||||||||||||||||||||||||||||
---|---|---|---|---|---|---|---|---|---|---|---|---|---|---|---|---|---|---|---|---|---|---|---|---|---|---|---|---|---|---|---|---|---|---|---|---|---|---|---|---|---|---|---|---|---|---|---|---|---|---|---|---|---|---|---|---|---|---|
|
Mitochondrial Encephalomyopathy, Lactic Acidosis, and Stroke-like Episodes Syndrome
MELAS is another maternally inherited disorder whose defining clinical features include (a) stroke-like episodes at a young age (typically before age 40); (b) encephalopathy manifest as seizures, dementia, or both; and (c) mitochondrial dysfunction with lactic acidosis, ragged-red fibers, or both.44,82 Other commonly encountered manifestations include normal early development, migraine headaches, myopathic weakness, exercise intolerance, myoclonus, ataxia, short stature, and hearing loss.44,82 It is uncommon for more than one family member to have the full MELAS syndrome; in most pedigrees, there is only one MELAS patient with oligosymptomatic or asymptomatic relatives in the maternal lineage.
Other Mitochondrial Encephalomyopathies with Epilepsy Caused by mtDNA Point Mutations
In addition to the three aforementioned common and well-defined phenotypes of mitochondrial diseases, several other epilepsy syndromes have been associated with point mutations of mtDNA (Table 3). These mutations reside predominantly in mitochondrial tRNA genes.50,64,78,86,99,106,114,115,125 Although less common, mutations in polypeptide-coding mitochondrial genes have also been reported in patients with epilepsy.11,13,17,65,75,124,130 Some of these mutations are associated with multisystem disorders such as maternally inherited Leigh syndrome (MILS),17,115 neuropathy ataxia retinitis pigmentosa (NARP),13 or Leber hereditary optic neuropathy
(LHON).11 In addition to epilepsy, other common manifestations include dementia, cardiomyopathy (typically hypertrophic) skeletal myopathy, and hearing loss.99,120
(LHON).11 In addition to epilepsy, other common manifestations include dementia, cardiomyopathy (typically hypertrophic) skeletal myopathy, and hearing loss.99,120
Mitochondrial Encephalomyopathies Associated with Nuclear Mutations
Leigh Syndrome
Subacute necrotizing encephalomyelopathy was originally described in 1951 by Dr. Denis Leigh, who reported a 6.5-month-old infant presenting with developmental regression that progressed quickly and led to death 6 weeks later.61 At autopsy, Dr. Leigh observed multiple symmetric foci of spongy degeneration with microvascular proliferation in the brainstem tegmentum, thalami, cerebellum, posterior columns of the spinal cord, and optic nerves. He astutely noted that the neuropathologic alterations resembled those of Wernicke syndrome but spared the mamillary bodies, a consistent feature that distinguishes the two disorders.
The clinical presentations of Leigh syndrome (LS) are heterogeneous, due to variations in age-at-onset, rates of progression, frequency of epilepsy, and presence or absence of pigmentary retinopathy. The onset is often acute and may coincide with a febrile illness or may follow a seizure. Most LS patients present in infancy with psychomotor regression, whereas some present in childhood or adolescence. Adult-onset LS is uncommon. In infants with LS, in addition to developmental regression, generalized hypotonia, feeding problems, progressive vision loss due to optic neuropathy or pigmentary retinopathy, progressive external ophthalmoplegia, hearing loss, nystagmus, ataxia, and seizures are typical manifestations. In addition, failure to thrive, dysarthria, vomiting, and diarrhea are common manifestations. Respiratory dysfunction is often prominent and frequently causes death. Most infantile-onset LS patients die before age 2 years. In older infants or young children, LS may begin with ataxia, dystonia, or intellectual decline.
The clinical diagnosis of LS is usually made by brain magnetic resonance imaging (MRI) scans, which reveal increased signals in the basal ganglia and brainstem on T2-weighted or FLAIR images. The lesions are typically symmetric and commonly affect the putamen, globus pallidus, caudate, thalami, substantia nigra, inferior olivary nuclei, periaqueductal gray matter, and brainstem tegmentum. Magnetic resonance spectroscopy (MRS) scans reveal decreased N-acetylaspartate and increased lactate in the affected brain regions.
The causes of LS are biochemically heterogeneous and, to an even greater extent, genetically diverse (Table 4). LS has been associated with defects of pyruvate carboxylase, pyruvate dehydrogenase complex (PDHC), and mitochondrial respiratory chain complexes I, II, IV, and V.10,98,126,129,135 The inheritance pattern depends on the specific mutated gene, but may
be autosomal recessive, X-linked recessive, or, as previously mentioned, maternally inherited.
be autosomal recessive, X-linked recessive, or, as previously mentioned, maternally inherited.
Alpers Syndrome
Alpers or Alpers-Huttenlocher syndrome was reported in 1931 by Bernard Alpers, who described a previously healthy 3-month-old girl, who developed intractable seizures, blindness, and became stuporous before dying at age 4 months.3 Neuropathology revealed widespread degeneration of the cortex and basal ganglia. Huttenlocher et al. described the pathologic findings in liver, which included microvesicular steatosis, proliferation of bile ducts, and cirrhosis.48 Clinically, Alpers syndrome is characterized by autosomal recessive inheritance, normal early development, episodic neurodegeneration with psychomotor regression, seizures that become intractable, and hepatopathy. The occipital lobe is frequently affected, leading to cortical blindness. Onset is usually in infancy or childhood, but can be as late as 25 years. In patients with Alpers disease, valproic acid should be avoided because this drug can precipitate fulminant hepatopathy.
Ultrastructurally abnormal mitochondria in cerebral neurons suggested that the disease might be a mitochondrial encephalomyopathy97; this notion was confirmed by the identification of mtDNA depletion in association with pathogenic mutations in the gene encoding the catalytic subunit of mitochondrial DNA polymerase-γ (POLG1).77
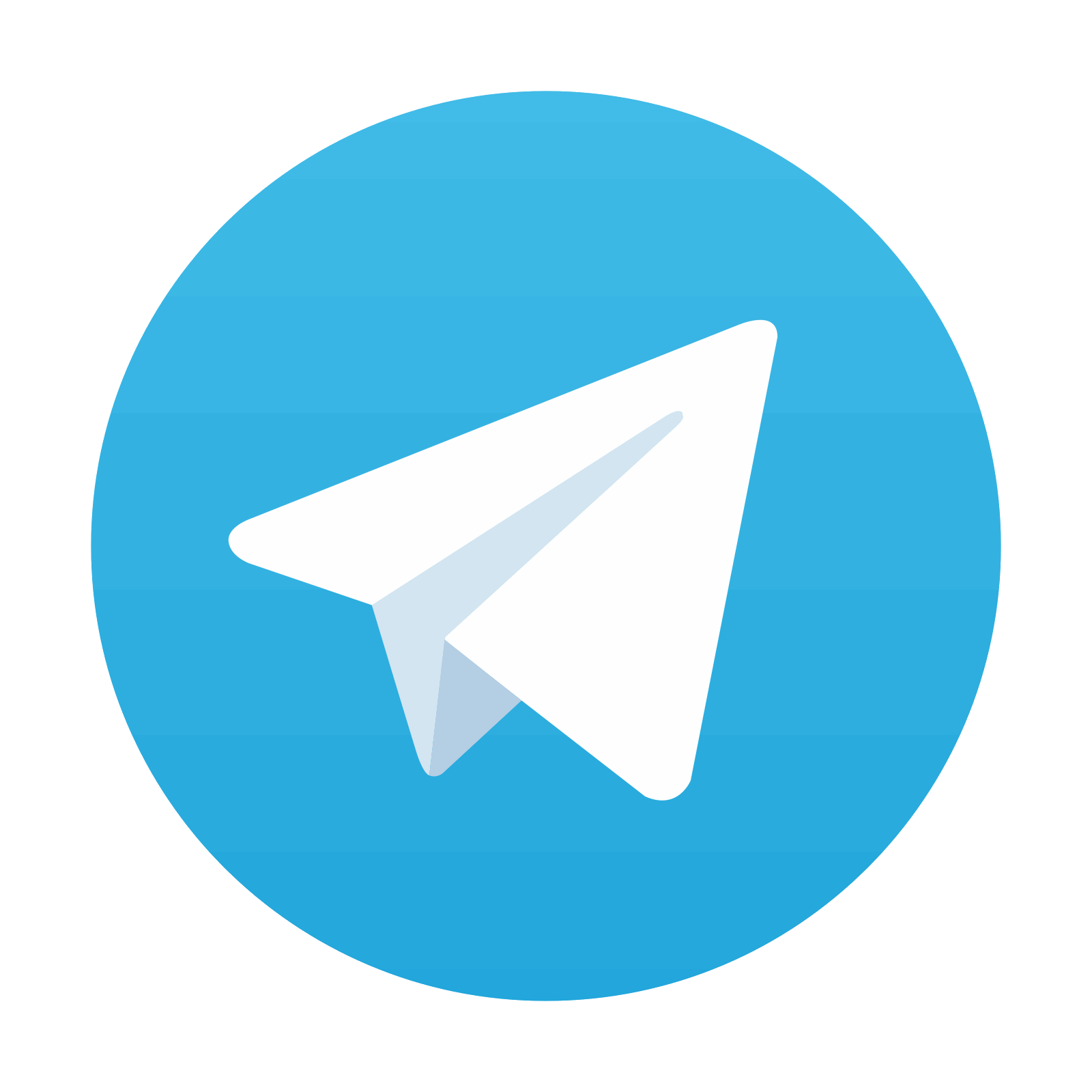
Stay updated, free articles. Join our Telegram channel

Full access? Get Clinical Tree
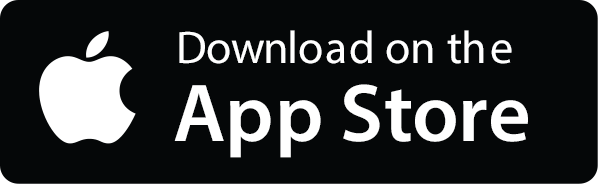
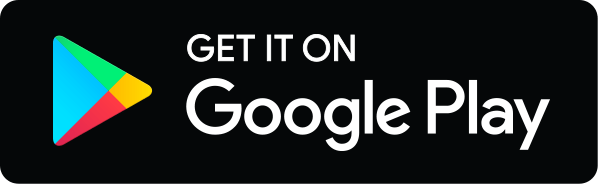
