© Springer International Publishing Switzerland 2015
M. Memet Özek, Giuseppe Cinalli, Wirginia Maixner and Christian Sainte-Rose (eds.)Posterior Fossa Tumors in Children10.1007/978-3-319-11274-9_4444. Molecular Biology and Genetics of Chordomas
(1)
Department of Neurosurgery, Acibadem University, School of Medicine, Istanbul, Turkey
(2)
Division of Pediatric Neurosurgery, Department of Neurosurgery, Acibadem University, School of Medicine, Istanbul, Turkey
44.1 Introduction
Chordomas are extra-axial, low-grade malignancies growing slowly, located anywhere from the clivus to the sacrum on the axial skeleton. Grossly they are unencapsulated, usually lobulated, pink-gray colored, invasive tumors with foci of hemorrhage and calcifications exhibiting epithelial phenotype. Histopathologically they are classified as classic chordomas, chondroid chordomas, and atypical chordomas [1, 2]. Classic chordomas are characterized by the presence of physaliferous cells which are large, vacuolated, mucus-containing cells with hyperchromatic, eccentric nuclei having prominent nucleoli rarely demonstrating atypia and reticulated cytoplasm due to intracellular accumulation of glycosaminoglycans, similar to the cells of notochord. The cytoplasm is eosinophilic and stains positive with the periodic acid Schiff stain. Other cell types are stellate cells and intermediate or transitional cells [3].
Annual incidence of chordomas is 0.08 per 100,000 population, more common in males (1.0) than females (0.6), and rare in patients under 40 years and blacks. Within the axial skeleton, 2 % are cranial, 32.8 % spinal, and 29.2 % sacral. Younger age and female sex are associated with a higher likelihood of cranial presentation. While the biological activity of chordomas varies considerably, more aggressive behavior is usually seen in the younger age group [4].
Chordomas are extremely rare tumors in children which are supposed to arise from the remnants of the embryonic notochord (from the Greek noton or notos, meaning back, + Latin chorda, meaning cord) originating from the ectoderm during the third week of embryogenesis, influencing the development of perinotochordial mesenchymal elements, and serving as the axial skeleton of the embryo until the formation of other vertebral elements. The notochord which expresses type X collagen is replaced by bone, but between the vertebrae, the notochord which does not express type X collagen expands and forms the nucleus pulposus. The notochord is most likely a primitive relative of cartilage and shares many characteristics with it like aggrecan, Sox9, calmodulin, and type II and IX collagen expressions [5].
Benign forms of chordal ectopias are called ecchordosis physaliphora and commonly encountered in the cranial base and axial skeleton of adults. In autopsy and radiologic studies, its incidence is 0.5–2 %. In a report of 100 autopsies, microscopic notochordal intraosseus remnants are found in 11 % of the clivus and 19 % of spinal vertebrae [6]. The supposed origin of ecchordosis physaliphora from the notochord is supported by electron microscopy and immunohistochemical studies. Some authors have proposed a chordoma tumorigenesis from these benign remnants, while others hypothesize a de novo origin. The hypothesis of chordomas originating from ecchordosis physaliphora is supported by similar morphology, similar immunophenotype, and similar localization [7, 8].
There are both genetic and immunohistochemical studies which find brachyury, a T-box transcription factor gene product, expression in embryonal notochord, and chordomas. Brachyury expression is required for the specification of mesodermal identity, representing one of the key genes regulating notochord formation and the differentiation of the notochord to nucleus pulposus, while it is absent in the nucleus pulposus. The brachyury protein is a useful marker for chordomas in cases where diagnosis and differentiation is difficult, increasing the sensitivity and specificity of the diagnosis. Expression of this protein specifically in the notochord and the chordomas is considered to be an evidence of the notochordal origin of chordomas.
The studies on the molecular biology and genetics of chordomas are increasing although most of the series have limited numbers, and there is not a specific study regarding pediatric age group chordomas. The studies show polyclonal origin of chordomas [9], and the genome-wide studies using comprehensive array techniques are still of limited number.
44.2 Familial Chordomas
First data regarding the genetics of chordomas came from familial chordoma cases. Foote et al. reported sacrococcygeal chordomas in middle-aged siblings [10], followed by few other familial chordoma reports [11–20]. Although congenital chordomas have been reported [21, 22], the youngest patient with a chordoma family history was a 3-year-old child [23].
Although familial chordomas were reported, cytogenetic or detailed genetic studies came later. Four affected patients in two generations were reported with a probable autosomal dominant inheritance pattern with complete penetrance but without cytogenetic abnormality [12], and the first cytogenetic study of familial chordomas (father and two daughters) found chromosomal hypo- or nearly diploidy and had a pronounced karyotypic heterogeneity with clonal and non-clonal rearrangements affecting several chromosomes, and a dic(1;9)(p36.1;p21) was found by fluorescence in situ hybridization (FISH) technique, and especially chromosome 1p appeared to be involved in unbalanced translocations with different chromosomes, leading to variable losses of 1p [13]. This family and six other sporadic tumors were studied later by combined use of loss of heterozygosity (LOH) and haplotype analyses, and they have found a susceptibility region on 1p36 related with a tumor suppressor gene [14]. In 2001, Kelley et al. have linked chordomas to chromosome 7q33 by performing linkage analysis using microsatellite markers in an extended pedigree of the family reported by Stepanek and other two unrelated families in a total of 16 chordoma patients [12, 15]. They have corroborated their results by using chromosome 7 and 1p36 single nucleotide polymorphisms (SNPs) in these families but could not detect the same changes in another fourth family in a study in 2005 which was studied previously by LOH and linkage analysis [16]. In another study, 7q LOH in one familial chordoma case was reported [18]. Yang et al. reviewed previously studied four chordoma families by using high-resolution array-comparative genomic hybridization (CGH) and identified a unique duplication of a region on 6q27 which contains only the T gene (brachyury) [20].
44.3 Genome-Wide Studies
Genome-wide studies in chordomas have shown many diverse chromosome aberrations, while most of them were hypodiploid or near diploid with both structural and numerical abnormalities. Karyotype analyses are comparable between chordoid and classic chordomas, and aneuploidy is rare in both types of chordomas. Those which have abnormal karyotype have higher recurrence rates.
In a study using CGH and FISH, the most common DNA copy number alterations in 16 chordomas were losses on chromosomal arms 3p (50 %) and 1p (44 %) suggesting tumor suppressor genes or mismatch repair genes (located at 1p31 and 3p14), and the most common gains involved 7q (69 %), 20 (50 %), 5q (38 %), and 12q (38 %) suggesting oncogenes (located in 7q36) might be involved in chordoma genesis [24].
Hallor et al. have published the first array-based genome study of chordomas in 30 tumor samples from 26 patients. All of those tumors were located in the spinal axis, and the median age of patients was 60 years. They have found multiple chromosome imbalances, and those which showed no karyotype anomaly had genomic imbalances upon aCGH analysis, especially CDKN2A, and CDKN2B deletions (9p21) have been found meaningful and expected larger deletions in that region with higher-resolution arrays [25]. In another study, chordomas highly expressed genes like type II collagen, aggrecan, SOX 9, and CD-RAP which are commonly found in the cartilage. They also found components of the activator protein 1 (AP-1) transcription factor to be highly expressed in chordomas in comparison to nucleus pulposus and articular cartilage tissue. The same pattern of expression was seen with FOSB, c-Fos, and c-Jun. Deregulation of AP-1 transcription factor is thought to be sufficient for tumorigenesis, and AP-1 is considered critical in the function of dominant oncogenes and plays a role in the invasiveness of the chordomas. Several genes are known to be regulated by AP-1 like EGFR, MMP3, and MMP9 [26]. Genes like T brachyury; CD24; keratins 8, 13, 15, 18, and 19; and discoidin domain receptor 1 were found to be highly expressed in concordance with the findings of Vujovic et al. They found that T brachyury was significantly expressed in chordomas but nucleus pulposus in concordance with other studies [27]. The fibroblastic growth factor receptor-RAS/RAF/MEK/ERK-ETS2/brachyury signalling pathway was later studied, but no mutation could be shown to be related with pathogenesis [28]. Diaz et al. have done high-resolution whole-genome analysis of skull base chordomas and have found a deletion at 9p involving CDKN2A, CDKN2B, and MTAP but at a much lower rate (22 %) than previously reported for sacral chordomas. At a similar frequency (21 %), they found aneuploidy of chromosome 3. Tissue microarray immunohistochemistry demonstrated absent or reduced fragile histidine triad (FHIT) protein expression in 98 % of sacral chordomas and 67 % of skull base chordomas suggesting that chromosome 3 aneuploidy and epigenetic regulation of FHIT contribute to loss of the FHIT tumor suppressor in chordomas [29].
44.4 Telomere Maintenance
Butler et al. studied five lumbosacral chordomas and found increased telomere length in four of them and increased telomerase activity in one of two of those tumors studied for telomerase activity [30], and in a later study, they found telomere elongation in four chordomas and no consistent chromosomal alterations [31]. In another study, Pallini et al. found correlation between human telomerase reverse transcriptase (TERT)-mRNA expression and shorter recurrence-free survival in 26 skull base chordomas. Most of the hTERT-positive tumors were also positive for p53 mutations, suggesting that telomerase dysfunction is combined with abnormal p53 function to initiate the unrestrained clonal expansion of the tumor cells [32]. In another study, low telomerase activity was found, but the study had only one chordoma case [33], while in another study, none of the three chordomas had telomerase activity [34]. Ricci et al. have established a tumor cell line from three chordomas which were aggressive, recurrent tumors; all of them had overexpressed telomerase activity and expressed hTERT immunohistochemically [35].
44.5 The Rb Gene, P53, and Cell Cycle Control
The Rb gene is one of the well-characterized tumor suppressor genes, and its inactivation has been shown to be associated with a number of cancers. The Rb gene is located on chromosome 13q14. The Rb gene plays a pivotal role in regulating the cell cycle, specifically control of the G1-S transition via its interaction with E2F transition complex and also can induce apoptosis through a pathway that involves p53 through a pathway involving P14 and MDM2 and p21.
LOH in the Rb gene has been demonstrated in two of seven chordomas at genetic level [36]. Alterations of pRb proteins have also been investigated in relation to MIB-1 labeling index, but no significant relation have been found [37].
Bergh, Kilgore, Naka, and Pallini found p53 overexpression in 0, 27 %, 30 %, and 40 % of the chordoma cases in their cohorts, respectively [32, 37–39]. In a comprehensive analysis, Naka et al. showed that high p53 levels in 30 % of their cases correlated well with increased mitotic index and decreased patient survival. In a significant proportion of human cancers, increased p53 levels result from mutations in the p53 gene. However, the authors found no mutations in the p53 gene in cases with increased p53 levels, possibly indicating an alternative mechanism for p53 protein accumulation. Mdm2 overexpression is a common mechanism of p53 inactivation in sarcomas, but not in chordomas [37]. Toguchida et al. could find no p53 mutations in three chordoma cases [40].
44.6 1p36 Locus
Genetic changes mapping to 1p36 have been found by Miozzo et al. in a family which was previously reported by Dalpra and six sporadic chordomas from five patients, and the additional LOH data performed on those sporadic tumors defined an SRO (the smallest region of overlapping loss) from 1p36.31 to 1p36.13 probably carrying a tumor suppressor gene [14]. Riva et al. performed a linkage analysis in 27 sporadic chordoma cases and mapped a defect to 1p36.13, common to 85 % of the cases. By performing RT-PCR analysis on candidate genes in this region, the authors suggested that caspase 9, ephrin 2A, and DVL1 genes may play a role in chordoma tumor suppression [41]. Yang et al. have studied a chordoma family which was studied before by using combined LOH and linkage analysis and reported to be linked to 1p36, by using SNP and microsatellite (STR) markers for 1p36, but they could not corroborate the same result [13, 16]. In another study using interphase FISH technique which included 7 primary and 11 recurrent chordomas from seven patients, 1p36 deletion was observed in 28.5 % of primary chordomas and 30 % of recurrent tumors, while 1p36 amplification was present in 14.2 % of primary chordomas and 60 % of recurrent chordomas [42]. Defects in 1p may be an early change in chordoma oncogenesis. Longoni et al. studied 1p36 expression in 16 skull base chordomas from 15 patients. They performed LOH analysis using 33 microsatellite markers mapped at 1p36.33–1p36.12 region, five microsatellite markers mapped at 1p, and six microsatellites mapped at 1q; 75 % of chordomas had 1p36 LOH. They have also studied eight apoptotic genes located in 1p36; superfamily of four tumor necrosis factor receptors, TNFRSF1B, TNFRSF8, TNFRSF9, and TNFRSF14, involved in cell death or survival signaling; CASP9, involved in the activation cascade of caspases and responsible for apoptosis execution; DFFA and DFFB, effectors of DNA fragmentation after apoptotic stimuli; and TP73, encoding a member of the p53 family. They studied the presence/absence of the transcripts of the above genes in the 15 chordoma samples by RT-PCR and compared the expression profiles of those with that of adult nucleus pulposus. In order to define an expression profile specific for nucleus pulposus for this set of genes, they also investigated other normal tissues: lymphocytes, placenta, fetal brain, fetal heart, fetal aorta, fetal skeletal muscle, and fetal and adult liver. Most of the studied genes were expressed evenly in nucleus pulposus compared to the other tissues. A notable exception was TP73, which was peculiarly not expressed in nucleus pulposus, whereas TNFRSF9 was expressed only in nucleus pulposus and lymphocytes. A third gene that did not show a similar expression profile in normal tissues was TNFRSF8 which was not detected in nucleus pulposus. They found widespread deregulation of TNFRSF8, TNFRSF9, and TNFRSF14 in the chordoma samples [43].
Considering all the selected genes, tumor samples showed variable expression profiles, none of which matched that of nucleus pulposus. The TNFRSF14 gene was differently expressed in six samples in comparison with nucleus pulposus; TP73 was differently expressed in two tumors, DFFB in four, TNFRSF9 in eight, DFFA in one, TNFRSF8 in seven, TNFRSF1B in three, and CASP9 in two chordomas. None of the analyzed samples showed the same expression profile observed in the reference tissue for all of the tested genes.
They then grouped the patients according to LOH status at 1p36 and observed that all events (recurrence/death) clustered in the group of 1p36 LOH-positive patients. They also grouped patients according to expression/non-expression for each of the mentioned genes. They observed that all events (recurrence or death) clustered in the group of TNFRSF8 expression-negative patients. They found no correlations between expression/non-expression of the other studied genes and clinical outcome [43].
44.7 Tuberous Sclerosis Complex, PTEN, and Akt/mTOR Pathway
There are case reports of chordomas in patients with the tuberous sclerosis complex. Two genes have been identified in tuberous sclerosis: TSC1 and TSC2. The TSC1 gene on chromosome 9q34 encodes a protein known as hamartin (from hamartoma); the TSC2 gene on chromosome 16p13.3 encodes for a protein called tuberin. TSC1/TSC2 complex inhibits the mammalian target of rapamycin (mTOR) signaling pathway, therefore suppressing cell growth. The mTOR pathway is a growth-promoting pathway and plays an essential role in a wide array of cellular processes including translation, transcription, trafficking, and autophagy. In two tuberous sclerosis complex patients, one with a germ-line TSC2 and the other with a germ-line TSC1 mutation having sacrococcygeal chordomas, Lee-Jones et al. showed somatic inactivation of the other wild-type allele by LOH and immunohistochemistry, but a causal relationship was not proven [44].
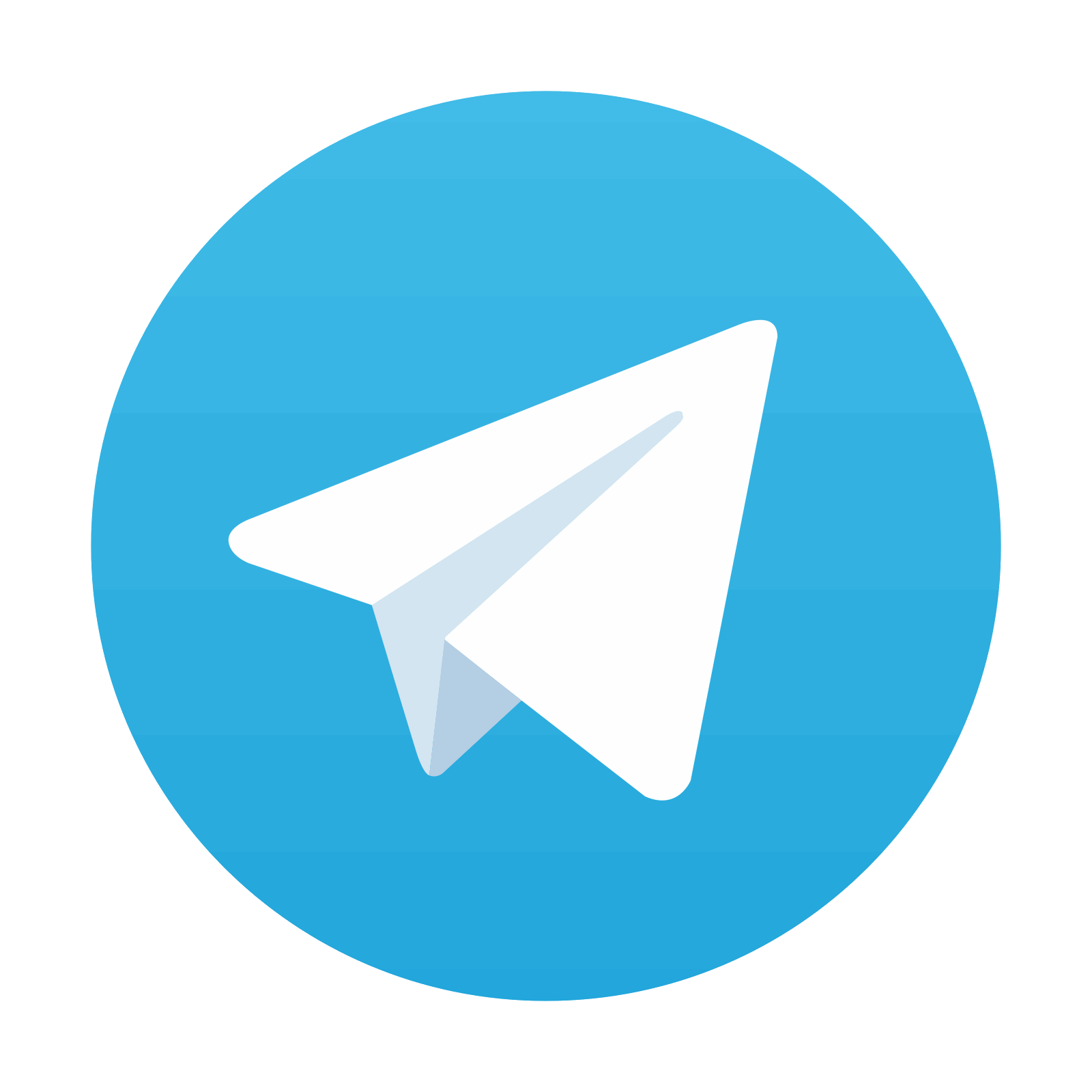
Stay updated, free articles. Join our Telegram channel

Full access? Get Clinical Tree
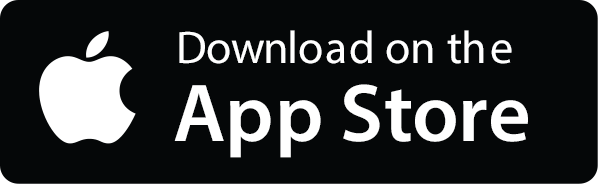
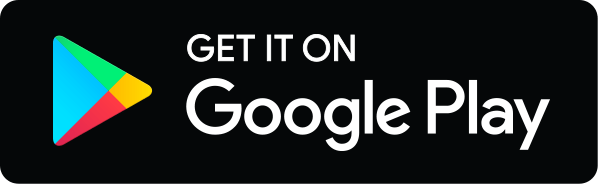