Fig. 4.1
In NETs, the study of inherited syndromes allowed the identification of the common deregulation of genes involved in the mTOR pathway in these tumors. As a master regulator of different cell functions, mTOR activation is subjected to tight and coordinated regulations through diverse positive and feedback regulatory loops. The more recent “next-generation sequencing” effort further identified high prevalence of ATRX/DAXX and PTEN mutations in pancreatic sporadic tumors. The upregulation of miR-21 also represents a major driver alteration in inherited and sporadic NETs
The most common NET-related inherited syndrome is the multiple endocrine neoplasia type I (MEN1; OMIM 131100). MEN1 is an autosomal dominant cancer susceptibility syndrome in which there is a greatly elevated risk of a variety of endocrine tumors, including pituitary tumors, parathyroid tumors, and pancreatic NETs (pNETs). By the clinical point of view, MEN1 patients typically harbor multiple small (<0.5 cm) pancreatic neuroendocrine microadenomas, which are thought to be precursor lesions to pNETs. These data support an early involvement of the MEN1 tumor suppressor gene in pNET tumorigenesis [6–8].
Menin, the protein encoded by the MEN1 gene, is a ubiquitously expressed nuclear protein that interacts with a SET1-like histone methyltransferase (HMT) complex containing the MLL (KMT2A) protein which functions to methylate lysine 4 of histone 3 (H3K4), an epigenetic modification associated with actively transcribed genes [9]. In normal pancreatic islet cells, menin appears to negatively regulate islet cell growth by targeting this HMT complex to the gene promoters of two key negative regulators of the cell cycle: p18 (CDKN2C) and p27 (CDKN1B), thus fostering their expression. Menin may exert additional effects on the cell cycle via the PI3K/Akt/mTOR signaling pathway by inhibiting the activity of the key serine-threonine kinase Akt1 [10].
In addition to its role on cell proliferation regulation, menin is also involved in the response to DNA damage. In fact, menin activates the transcription of several genes whose protein products are involved in the homologous recombination pathway of DNA repair (e.g., BRCA1 and RAD51) [11]. As a result, depletion of menin leads to increased use of nonhomologous end joining DNA repair activity, a more error-prone repair pathway [12].
Taken together, the above results suggest that loss of menin causes deregulation of normal cell growth control in conjunction with increased genomic instability, an ideal setting for the initiation of malignant transformation. MEN1 mutations or chromosomal deletions involving the MEN1 locus at 11q13 locus have been observed in up to 30 % NET sporadic cases [2, 13–21]. To further support these findings, in vivo studies demonstrated that MEN1 deficiency leads to pancreatic islet cell hyperplasia and neuroendocrine tumors [22]. Weak or negative nuclear expression of menin, as assessed by immunostaining, has been reported in 40 % of sporadic pNET cases, while 27 % of cases had MEN1 gene mutations, most of which were inactivating mutations [13]. In lung carcinoids, the presence of MEN1 mutation or loss had shorter overall survival and low MEN1 mRNA levels correlated with distant metastasis and shorter survival [23].
A second autosomal dominant hereditary syndrome is the multiple endocrine neoplasia type 2 (MEN 2). MEN2 is associated with NETs occurring in the thyroid, in the parathyroid, and in the adrenals [24]. The most common tumor among these patients is the medullary thyroid carcinoma (MTC), and overall the 25 % of MTCs are hereditary cases. By the clinical point of view, MEN2 is subclassified in three further inherited syndromes MEN2A, MEN2B, and familial medullary thyroid cancer, which are all caused by mutations of the RET oncogene. RET encodes a receptor tyrosine kinase that normally binds a family of ligands including glial-derived neurotrophic factor and is thought to provide growth and survival signaling via the RAF-MEK-ERK and PI3K/Akt/mTOR pathways [24]. Thus, activating mutations in RET can confer ligand-independent growth and resistance to apoptotic stimuli.
Also the von Hippel-Lindau disease is an autosomal dominant predisposition syndrome featuring an elevated rate of NETs (VHL; OMIM 193300). This syndrome is caused by mutation of the VHL tumor suppressor gene located at 3p25 and features nonfunctional pNETs in 5–17 % of patients [25]. Compared to the MEN1 gene, the VHL gene is infrequently mutated in sporadic tumors. However, the VHL gene may be inactivated by alternative means, such as promoter hypermethylation or deletion, as has been reported for up to 25 % of pNETs [26]. The VHL gene product is involved in the oxygen-regulated degradation of hypoxia-inducible-factor alpha, a transcription factor which regulates gene expression in response to low-oxygen conditions, and tumors harboring VHL gene inactivation display elevated expression of HIF1 alpha target genes [26].
Two other inherited autosomal dominant cancer-predisposition syndromes that develop NETs, albeit infrequently, are neurofibromatosis type I (NF1; OMIM 162200) and tuberous sclerosis (TS; OMIM 191100). NF1 (formerly known as von Recklinghausen disease) is caused by a germline mutation of the NF1 gene at 17q11.2, which encodes the protein neurofibromin. This protein normally functions as a negative regulator of the Ras oncogene and the mTOR signal transduction pathways [27]. Tuberous sclerosis is caused by mutations in one of two different genes: the TSC1 gene located at 9q34 and the TSC2 gene located at 16p13.3, which encode for the proteins hamartin and tuberin, respectively. Despite a rare occurrence of NETs in the frame of a TS syndrome [28], the TSC2 gene has an important role in sporadic pNET pathogenesis. Our group demonstrated that Tsc2 expression is downregulated in 35 % of sporadic pNETs, and, more recently, Jiao and colleagues found the TSC2 gene to be mutated in 8.8 % of sporadic pNETs [17, 29]. As with neurofibromin, tuberin also functions as a negative regulator of the mTOR pathway, thus lending further support for the potential importance of deregulation of this pathway in NETs.
4.3 Next-Generation Sequencing Era: The Discovery of Novel Cancer Pathways
The advent of massively parallel, high-throughput sequencing, also known as “next-generation sequencing” (NGS), has exceptionally revolutionized the field of cancer genetics over the past decade. This technology allows for the identification of tumor-associated genetic alterations with single-nucleotide level precision, in an unbiased manner, across the entire genome or the entire exome, which consists of the protein-coding regions of the genome [30]. The recognition of driver oncogene mutations in specific tumors has provided a compelling rationale for the introduction of novel targeted therapies [4].
The first NET whole-exome study was published by Jiao and colleagues in 2011 on pNETs [17]. An average mutation rate of 16 mutations per tumor was significantly lower than that previously observed for pancreatic ductal adenocarcinoma (i.e., 66 mutations per tumor) [31]. Furthermore, the genes most frequently mutated in pancreatic adenocarcinoma (CDKN2A, KRAS, TP53, TGFBR1, SMAD3, and SMAD4) were either wild type or only rarely mutated (TP53; 5 %) in PanNETs. As anticipated from prior studies, MEN1 was found to be frequently mutated (44 %). In addition to MEN1, genes in the mTOR signaling pathway were also mutated in a significant frequency of cases, with inactivating mutations in TSC2 (8.8 %) and PTEN (7.3 %) observed, plus a single case was found to possess an activating mutation in PIK3CA.
Two novel genes were implicated in NET carcinogenesis: the ATRX (alpha-thalassemia/mental retardation syndrome, X-linked; OMIM 300032; located on Xq21.1) and DAXX (death domain-associated protein; located on 6p21.3) genes. A total of 43 % tumors possessed either an ATRX or DAXX gene mutation (18 % and 25 %, respectively). Intriguingly, mutations in ATRX or DAXX were mutually exclusive, implying that ATRX and DAXX function in a common pathway important in the pathogenesis of pNETs. In 53 % of cases with either ATRX or DAXX mutations, MEN1 was also found to be mutated as well.
Atrx is a large nuclear protein of the SWI/SNF family of chromatin remodeling proteins [32]. Germline mutations in the ATRX gene cause the rare syndrome ATRX, which features alpha-thalassemia plus impaired intellectual development [33]. The types of ATRX gene mutation differ significantly between those causing the ATRX syndrome and those associated with pNETs. Inherited syndromic ATRX mutations are typically hypomorphic missense mutations, whereas sporadic cancer-associated mutations tend to be nonsense mutations or insertions and deletions which lead to a loss of protein expression [17, 33, 34]. This is in keeping with the observation that engineered ATRX loss in the mouse is embryonic lethal. Thus, inherited inactivating mutations in humans are likely also to be lethal and, as such, are not observed. Furthermore, individuals with ATRX syndrome do not appear to have an elevated risk of the types of tumors associated with somatic inactivating ATRX mutations [17].
Daxx is also a nuclear protein involved in the regulation of chromatin structure and gene transcription. Daxx contains a SUMO-recognition motif, responsible for its association with numerous proteins in their SUMO-modified (sumoylated) state, including a number of transcription factors [35, 36]. Of particular note, Daxx binds the histone variant H3.3 and physically interacts with Atrx to form a histone chaperone-chromatin remodeling complex [37]. This complex acts to deposit H3.3 at a number of locations throughout the genome, including the upstream regions of several active genes, as well as both peri-centromeric and telomeric heterochromatin [37, 38].
Heaphy and colleagues demonstrated that mutations in either ATRX or DAXX or loss of protein expression were perfectly correlated with the alternative telomerase-independent telomere maintenance mechanism termed alternative lengthening of telomeres (ALT) [34]. At the cellular level, the ALT mechanism is characterized by abnormally enlarged PML nuclear bodies containing large amounts of telomeric DNA repeats, readily detectable in fixed archival tissue specimens via telomere-specific FISH analysis [39]. Intriguingly, whereas ALT is observed in approximately half of all PanNET cases, to date it has only rarely been observed in other NETs: in 5 of 107 cases of gastrointestinal NETs and 0 of 7 lung carcinoids [34, 40].
In order to evaluate the timing of ATRX and DAXX mutations during pNET tumorigenesis and progression, de Wilde and colleagues assessed Atrx or Daxx nuclear protein expression and ALT status in tissues obtained from a cohort of MEN1 syndrome patients [41]. Loss of either Atrx or Daxx and ALT-positivity were found exclusively in PanNETs and not in any of neuroendocrine microadenomas. In addition, a significant correlation was observed between these abnormalities and higher tumor grade (G2 versus G1), and tumor diameter >3 cm. Overall, these findings suggest that ATRX and DAXX mutations are relatively late events in pNET tumorigenesis.
Of interest, the spectrum of commonly mutated genes in poorly differentiated, high-grade pancreatic neuroendocrine carcinomas (pNECs) differs substantially from those seen in either pancreatic adenocarcinomas or pNETs [42]. KRAS and CDKN2A, which are commonly mutated in adenocarcinomas, and MEN1, ATRX, DAXX, and mTOR pathway genes, frequently mutated in pNETs, are mutated infrequently or not at all in pNECs. On the other hand, mutations in TP53 and RB1 are very common in this group of tumors. TP53 is rarely mutated in well-differentiated pNETs, but its pathway is likewise inactivated by the frequent prevalence of extra gene copies of p53-related genes, such as MDM2 (22 %), MDM4 (30 %), and WIP1 (51 %) [43]. In the same way, 80 % of pNETs show an unchanged RB1 gene but display genetic alterations of other components of the Rb pathway, such as cyclin-dependent protein kinase 4 and 6 and cyclin D [44].
In addition to pNETs, the genomic landscape of small intestinal NETs (SI NET) has recently been investigated [45, 46]. The group led by Matthew Ames [45] performed an integrative analysis of somatic mutations and copy number variations of 48 small intestine NETs. They reported genetic alterations of different members of the PI3K/Akt/mTOR pathway in 30 % of the samples and the loss of SMAD genes in 45 % of samples. Overall, the average per case mutation rate in this study was significantly lower than that of typical carcinomas but comparable to that reported for pNETs [17]. Among these small intestinal NETs, a positive correlation was observed between primary tumors with higher numbers of mutations and the presence of liver metastases. Interestingly, although mutations were identified in several genes previously associated with cancer (e.g., BRAF, EZH2, FANCD2, FGFR2, MEN1, VHL), these mutations were found in only single cases in this series of 48 carcinoid tumors. Notably, many other cancer genes, including those frequently mutated in pNETs, pNECs, and pancreatic adenocarcinomas, were wild type in all of the SI NETs sequenced. Concomitantly, another group identified p27 (CDKN1B) as a putative tumor suppressor in small intestinal NETs [46]. CDKN1B encodes a cyclin-dependent kinase inhibitor p27Kip1, which is a master regulator of the cell cycle and frequently inactivated in many forms of cancer. Importantly this gene is inactivated in MEN1 patients testing negative for MEN1 mutations [47]. While these mutations may be present in a subset of carcinoid patients, they do not account for the vast majority of the neoplastic lesions [45, 46].
The whole-exome sequencing of 17 sporadic MTCs showed that approximately 90 % of tumors had mutually exclusive mutations in RET, HRAS, and KRAS genes, suggesting that RET and RAS are the predominant driver pathways in MTC [24]. Relatively few other mutations and no commonly recurrent driver mutations were observed.
A recent gene copy number analysis, genome/exome, and transcriptome sequencing of pulmonary carcinoids demonstrated frequent mutations in chromatin remodeling genes. Covalent histone modifiers and subunits of the SWI/SNF complex were mutated in 40 % and 22 % of the cases, respectively, with MEN1, PSIP1, and ARID1A being recurrently affected [48]. In contrast to small-cell lung cancer and large-cell neuroendocrine lung tumors, TP53 and RB1 mutations were rare events.
4.4 Epigenetic Changes in NETs
In recent years, thanks to the advent of molecular techniques allowing genome-wide assessment of epigenetic marks, such as chromatin immunoprecipitation sequencing (ChIP-Seq) and genome-wide DNA methylation analysis, it has become readily apparent that epigenetic abnormalities are widespread in cancer
DNA methylation has been studied rather extensively in a variety of NETs [49]. The long-standing observation that menin is an epigenetic regulator being part of a histone methyltransferase complex further supports that epigenetic, rather than genetic, changes may play a central role in NETs.
Using global analysis of the methylation status of LINE-1 and Alu repeat sequences that are widely distributed throughout the genome, it was found that these sequences were hypomethylated in the vast majority of cases, when compared to adjacent normal tissues and, when present, hypomethylation correlated with clinicopathological parameters such as tumor grade [50, 51].
Another phenomenon involving widespread DNA methylation is CIMP, which stands for CpG island methylator phenotype. First described in colorectal cancer, CIMP-positive tumors display simultaneous abnormal methylation of multiple CpG islands, including several associated with known tumor suppressor genes. In a large series of foregut and midgut NETs, CIMP prevalence was 50 % in gastrinomas and 100 % in VIPomas and glucagonomas [52]. CIMP has also been found in over half of poorly differentiated colorectal neuroendocrine carcinomas, where it was also associated with MSI positivity [53, 54].
Among the others, three genes have been demonstrated to be highly methylated in NETs: MGMT, RASSF1A, and CDKN2A.
MGMT is commonly deficient in pNETs (up to 51 % of cases) and, more importantly, its status can be used to predict response to alkylating agents. On the other hand, MGMT is usually expressed in most G1 GEP-NETs, which likely explains much of the differential sensitivity to temozolomide-based therapies between these two tumor types [55, 56].
The RASSF1A tumor suppressor gene, located on 3p21, is rarely mutated in cancer; however, it is frequently silenced via promoter hypermethylation [57]. In pNETs, the RASSF1A promoter is reportedly methylated in 75–83% of lesions, where methylation is correlated with reduced RASSF1A mRNA expression, increased tumor size, and the presence of metastases [55, 58–60]. However, RASSF1A methylation is also observed in a significant fraction of tumor-adjacent normal pancreas tissue, strongly affecting its specificity as potential tumor biomarker [55, 58–60].
The CDKN2A gene codes for the p16 tumor suppressor protein, a member of the Rb cell cycle regulatory pathway that, among other cellular processes, regulates entry into S-phase. This pathway is thought to be disrupted in practically all human cancers, and CDKN2A has been reported to be methylated in 10–58 % of pNETs and up to 44 % of small intestinal carcinoids [44, 55, 61]. Unlike the case of RASSF1A, methylation of the CDKN2A displayed good specificity for tumor tissue versus adjacent normal tissue [44, 55, 61].
4.5 microRNA Deregulation in NETs
In contrast to many other tumor types, very little is known about microRNA (miRNA) expression patterns in NETs. However, a significant number of oncogenic and suppressor miRNAs have been identified so far, further supporting the possible use of specific miRNAs signatures to predict clinical outcome in NETs [62–65].
Of interest, unlike most mRNAs, miRNAs are long-living in vivo and very stable in vitro. This structural solidity is fundamental to miRNAs analysis in FFPE samples, in which they can also be investigated at the level of individual cells by applying in situ hybridization [ISH] techniques [66]. Another important point is the evidence for applicability of miRNAs as noninvasive biomarkers, as they are easily and reproducibly detectable in all body fluids [66].
In our seminal report, we investigated global microRNA expression signatures of normal pancreas, Langerhans’ islets, pNETs, and pancreatic acinar carcinomas [62]. The overexpression of miR-103 and miR-107, associated with lack of expression of miR-155, significantly discriminated tumor from normal samples. Moreover, a set of 10 miRNAs (miR-125a, miR-99a, miR-99b, miR-125b-1, miR-342, miR-130a, miR-132, miR-129-2, miR-125b-2) allowed the discrimination of PNETs from acinar tumors. This specific miRNA signature is possibly associated with either normal endocrine differentiation or endocrine tumorigenesis.
Among pNETs, miR-204 was primarily expressed in insulinomas (well-differentiated) and correlated with immunohistochemical expression of insulin. On the other hand, miR-21 overexpression was associated with higher Ki67 proliferation index and the presence of liver metastases [62]. The fact that PTEN is one of miR-21 targets further supports the increasing evidence of mTOR pathway involvement in NET pathogenesis.
Further confirming miRNAs’ stability in body fluids, a nine-miRNA expression pattern (miR-24, miR-30a-3p, miR-18a, miR-92a, miR-342-3p, miR-99b, miR-106b, miR-142-3p, and miR-532-3p) derived from the analysis of selected miRNAs in cyst fluid samples, successfully discriminated cystic forms of pNETs from other – more common – pancreatic cystic lesions [67].
Another group from the Johns Hopkins identified the downregulation of miR-1290 that had the best diagnostic performance in discriminating pNETs from adenocarcinomas [68].
Two relatively recent miRNA expression profile studies find evidence of miRNA deregulation in ileal NET progression [63, 64]. Rubel and colleagues from Mayo Clinic associated miRNA-133a downregulation to progression from primary to metastatic carcinoid tumor, suggesting that it may have an important role in ileal NET development and progression with use for diagnosis and/or prognosis [63]. The same group in collaboration with the Uppsala University investigated miRNA expression in well-differentiated small intestinal NETs. Nine miRNAs were significantly deregulated: five (miR-96, miR-182, miR-183, miR-196, and miR-200) were upregulated during tumor progression, whereas four (miR-31, miR-129-5p, miR-133a, and miR-215) were significantly downregulated in ileal NETs [64].
4.6 From Single Gene Alteration to Signaling Pathways Perturbations
In recent years, much attention has focused on identifying key cellular signal transduction pathways that are abnormally activated or deactivated in cancer cells [71]. Such pathways regulate cancer-relevant cellular processes, such as cell growth, cell division, and cell survival. Typically, these pathways involve cascades of cytoplasmic kinases that ultimately impinge on gene transcription. Therapeutically, kinases are attractive drug targets; thus, there is keen interest in elucidating the specific pathways altered in a given tumor in order to identify relevant targets and help predict treatment responses.
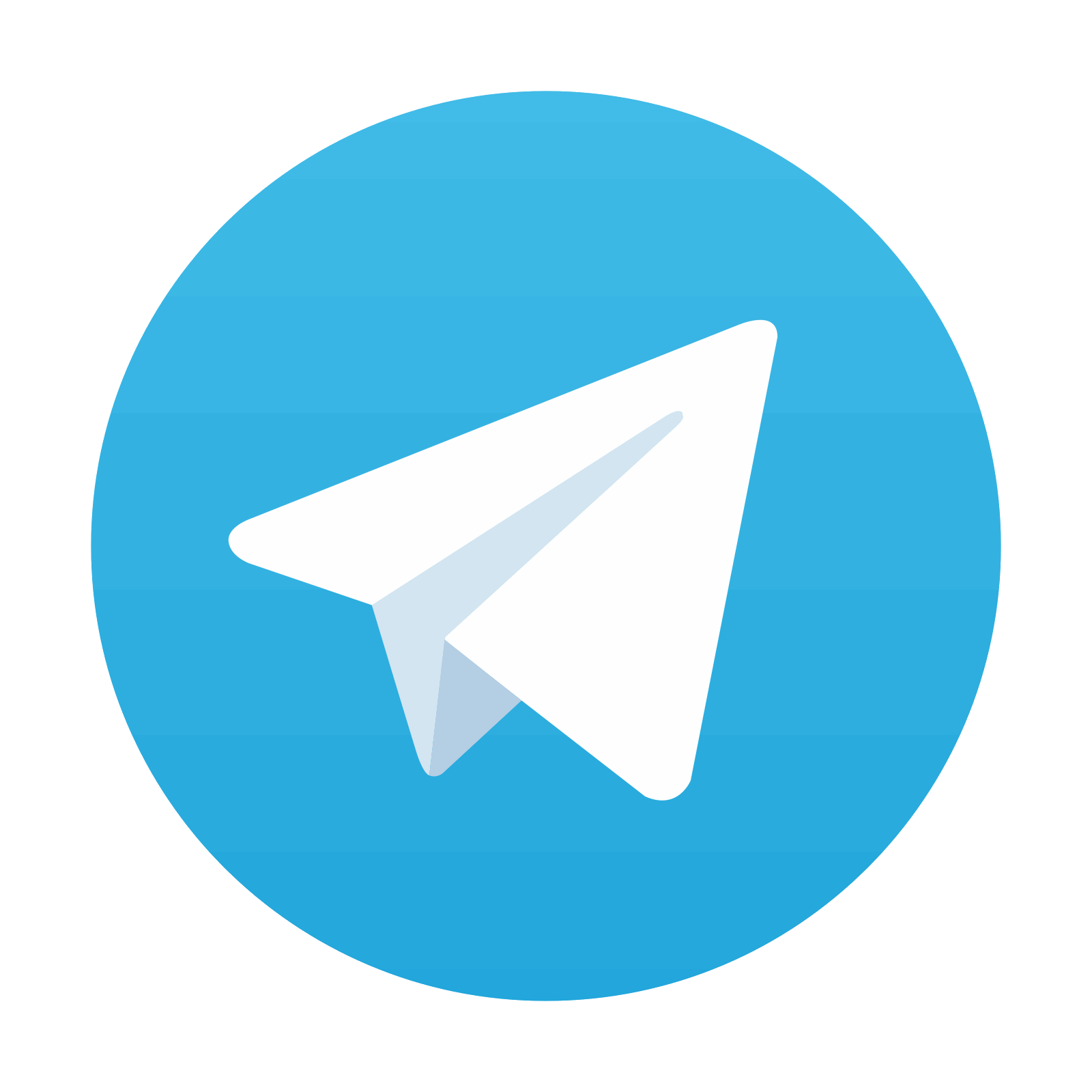
Stay updated, free articles. Join our Telegram channel

Full access? Get Clinical Tree
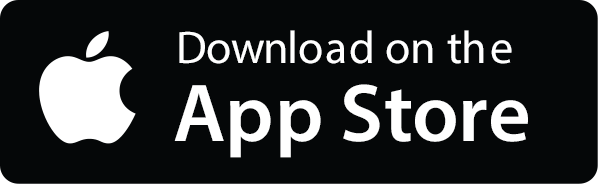
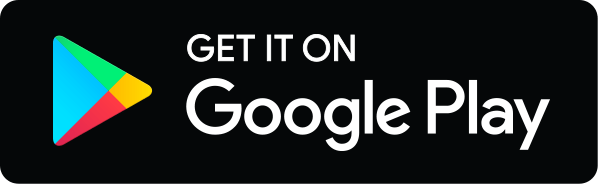