Fig. 2.1
The panels display the new ‘layered’ approach to the diagnosis of malignant gliomas as suggested by the ‘ISN (International Society of Neuropathology) Haarlem consensus guidelines’ and revised World Health Organization (WHO) classification. The light microscopic evaluation of gliomas begins the process of glioma classification and grading according to 2016 WHO standards (Preliminary Typing and Grading). Included are lineage-specific immunohistochemical stains such as GFAP. A second step involves molecular testing/biomarker detection for further subclassification and stratification. The results of both, morphology and biomarker analysis, are combined into a ‘final integrated diagnosis’. Certain mutational profiles appear to be mutually exclusive and define tumor lineages: The combination of IDH1/1p19q/TERT defines oligodendrogliomas, whereas the combination of IDH1/p53/ATRX is typical for astrocytic tumors. Additional molecular tests are included to add further prognostic and predictive value (e.g., MGMT)
Diffuse Astrocytic and Oligodendroglial Gliomas
The inclusion of molecular markers led to significant changes in the 2016 classification system of gliomas. In prior editions, astrocytic gliomas, oligodendrogliomas, and mixed oligoastrocytic gliomas each formed a separate entity within the larger category of neuroepithelial neoplasms [1]. The 2016 WHO classification (see Table 2.1) merges these gliomas into a single group as ‘Diffuse astrocytic and oligodendroglial tumors’ [3]. Aside from their infiltrative growth pattern, diffuse gliomas share frequent isocitrate dehydrogenase (IDH) mutations, a hallmark genetic alteration, which plays a significant role for the stratification of gliomas (please see below). Seminal studies could demonstrate that IDH-mutant gliomas are biologically and clinically distinct from IDH-wild-type gliomas [12, 13].
Table 2.1
2016 WHO Classification of Gliomas
Tumor entity/variant | WHO grade |
---|---|
Diffuse astrocytic and oligodendroglial tumors | |
Diffuse astrocytoma, IDH-mutant | II |
Gemistocytic astrocytoma, IDH-mutant | II |
Diffuse astrocytoma, IDH-wild type | II |
Diffuse astrocytoma, NOS | II |
Anaplastic astrocytoma, IDH-mutant | III |
Anaplastic astrocytoma, IDH-wild type | III |
Anaplastic astrocytoma, NOS | III |
Glioblastoma, IDH-wild type | IV |
Giant cell glioblastoma | IV |
Gliosarcoma | IV |
Epithelioid glioblastoma | IV |
Glioblastoma, IDH-mutant | IV |
Glioblastoma, NOS | IV |
Diffuse midline glioma, H3K27M-mutant | IV |
Oligodendroglioma, IDH-mutant and 1p/19q-co-deleted | II |
Oligodendroglioma, NOS | II |
Anaplastic oligodendroglioma, IDH-mutant and 1p/19q-co-deleted | III |
Anaplastic oligodendroglioma, NOS | III |
Oligoastrocytoma, NOS | II |
Anaplastic oligoastrocytoma, NOS | III |
Other astrocytic tumors | |
Pilocytic astrocytoma | I |
Pilomyxoid astrocytoma | II |
Subependymal giant cell astrocytoma | I |
Pleomorphic xanthoastrocytoma | II |
Anaplastic pleomorphic xanthoastrocytoma | III |
Ependymal tumors | |
Subependymoma | I |
Myxopapillary ependymoma | I |
Ependymoma | II |
Papillary ependymoma | II |
Clear cell ependymoma | II |
Tanycytic ependymoma | II |
Ependymoma, RELA fusion-positive | II or III |
Anaplastic ependymoma | III |
Other gliomas | |
Chordoid glioma of the third ventricle | II |
Angiocentric glioma | I |
Astroblastoma |
Diffuse gliomas form the vast majority of glial neoplasms and are primarily classified according to their histopathological appearance as astrocytic, oligodendroglial, or mixed oligoastrocytic tumors. Although the cellular origin is still under investigation, the histological classification (cell lineage) relies on morphological similarities of tumor cells with their presumed non-neoplastic counterpart. Diffuse gliomas are graded in a tiered system as WHO grade II (low-grade), WHO grade III (anaplastic), or WHO grade IV (glioblastoma and variants). The grade is based on histological criteria such as cell density, nuclear atypia, cellular pleomorphism, mitotic activity, vascular proliferation, and necrosis. It can be viewed as ‘malignancy scale’ that is used to predict the biological behavior of neoplasms [1, 3].
As the name implies, diffuse gliomas display a diffusely infiltrative growth pattern with tumor cells invading brain parenchyma as single cells or small groups of cells. The ability to diffusely disseminate in a single-cell fashion throughout the brain is a rather unique feature among tumor cells and typical of glioma cells. They have the remarkable ability to migrate over long distances along myelinated fiber tracts and not infrequently cross the corpus callosum to infiltrate the contralateral hemisphere (‘butterfly glioma’) or follow descending fiber tracts. The accumulation of glioma cells around neurons (‘perineuronal satellitosis’), around blood vessels and under the pial membrane (‘secondary structures of Scherer’) are additional classic features [14].
Histological Profiles of Diffuse Astrocytic and Oligodendroglial Tumors
Diffuse Astrocytic Tumors
The incidence of diffuse astrocytomas differs somewhat regionally, but recent estimates suggest an incidence rate of 0.4 per 100,000 people for WHO grade II astrocytomas and an incidence rate of 3.2 per 100,000 people for glioblastomas. The histological grade shows a direct correlation with the age at presentation, as WHO grade II tumors tend to present in younger adults in their 4th or 5th decades, while glioblastomas (WHO grade IV) peak in the elderly (mean age at diagnosis 61 years). Males appear to be more affected than females with a male:female ratio of 1.5:1.0 for all astrocytic tumors [15].
WHO grade II diffuse astrocytomas are morphologically heterogeneous and characterized by a higher degree of cellular differentiation, relatively slow growth, low mitotic activity, diffuse infiltration, and spread into adjacent brain structures (Fig. 2.2a). Tumor cells express GFAP (glial fibrillary acidic protein), a protein typically found in astrocytomas. WHO grade II diffuse astrocytomas can be found at any site within the CNS, but preferentially within the cerebral hemispheres, particularly within the subcortical and deep white matter of frontotemporal lobes. Although these lesions are rare in children, the main site in pediatric patients is the brain stem (so-called brain stem glioma). The 2016 WHO classification removed two variants, fibrillary and protoplasmic astrocytoma, due to lack of reproducible definition. The gemistocytic variant, which shows a very distinct appearance with eccentrically placed nuclei and dense cytoplasm, remains. Further, gliomatosis cerebri, previously defined by the diffuse involvement of several cerebral lobes, was also removed as separate entity, and it is simply viewed as an extreme example of widespread dissemination of tumor cells [3, 16].


Fig. 2.2
Morphologic appearance of gliomas (hematoxylin- and eosin-stained sections). a Diffuse astrocytoma, WHO grade II, characterized by low cellularity and mild nuclear pleomorphism; b Anaplastic astrocytoma, WHO grade III with increased cellularity and anaplastic nuclei; c Glioblastoma, WHO grade IV with pleomorphic tumor cells, mitoses, and pseudo-palisading necrosis; d Diffuse midline glioma with a high degree of pleomorphism; e Oligodendroglioma, WHO grade II, with relatively round to oval cell nuclei and typical cytoplasmic clearing (‘fried-egg’ appearance); f Ependymoma, WHO grade II, relative monomorphous appearance of small tumor cells which form perivascular pseudorosettes
Anaplastic astrocytomas (WHO grade III) are defined as diffuse astrocytomas with focal or dispersed anaplasia. These tumors are grossly more discernible since they are more cellular and form a more readily identifiable tumor mass. The infiltrative nature tends to create an overall increase in tissue volume without inducing a destructive effect. They are seen to arise from low-grade astrocytomas, but are also frequently diagnosed at first biopsy, without indication of a less malignant precursor lesion. In comparison with low-grade tumors, these neoplasms are microscopically remarkable for increased cellularity and enlarged, irregular hyperchromatic nuclei (Fig. 2.2b). Capillaries are lined by a single layer of endothelium, and frank vascular proliferation and necrosis are not present. Immunoreactivity for GFAP is less consistent than that for grade II lesions. In contrast to low-grade astrocytomas, these lesions display increased mitotic activity with a proliferative index (Ki-67/MIB-1 labeling) of 5–10% [3].
Glioblastomas (WHO grade IV) are the most malignant tumors within the spectrum of diffuse astrocytomas and account for up to 60% of all astrocytic tumors [15]. They affect mainly adults with a peak incidence between 40 and 70 years. Less than 10% of glioblastomas arise from a lesion of lower malignancy grade (secondary glioblastoma) and manifest in younger patients (mean age of 45 years). Most are found de novo (primary glioblastoma) after a short clinical history and are seen in older individuals (mean age 62 years). The majority of tumors are located within the cerebral hemispheres and show a tendency to infiltrate deep nuclei and spread along white matter tracts to the contralateral hemisphere. Brain stem involvement is rare and mainly present in children. Sites such as spinal cord or cerebellum are infrequently involved. Microscopically, glioblastomas are extremely heterogeneous and show a higher degree of cellularity, nuclear atypia, cellular pleomorphism, and mitotic activity, in addition to microvascular proliferation and (palisading) necrosis (Fig. 2.2c). The latter two features are the cardinal diagnostic features of glioblastomas and help distinguish them from grade III astrocytomas. Three distinct glioblastoma variants are part of the 2016 classification, which are giant cell glioblastoma, gliosarcoma, and the recently added variant of epithelioid glioblastoma. [3].
Giant cell glioblastoma is a variant remarkable for the presence and predominance of many markedly large and bizarre appearing, multinucleated giant cells, within an abundant stromal reticulin network. In spite of their unusual appearance, the consistent expression of GFAP in conjunction with data from genetic profiling confirmed their astrocytic nature.
Gliosarcomas are defined as high-grade astrocytomas with an intermixed sarcomatous component. Gliosarcomas are relatively rare and represent about 2% of all glioblastomas. The clinical features are similar to those of classic glioblastomas. Critical diagnostic parameters are a biphasic growth pattern with areas of glial and mesenchymal differentiation. Molecular changes are variable, but similar to those occurring in glioblastoma; however, tumor histogenesis is still controversial.
Diffuse Oligodendroglial Tumors
Oligodendrogliomas form a group of diffusely infiltrative glial tumors with features reminiscent of oligodendrocytes. Oligodendrogliomas account for approximately 5–6% of all glial neoplasms, and overall for 2–3% of all primary brain tumors. The annual estimated incidence rate lies within a range of 0.27–0.35 per 100,000 individuals. Although oligodendrogliomas can develop at any age, the majority of tumors arise within the 4th–5th decade, and less than 2% of oligodendrogliomas are found in children younger than 14 years. Males are more affected than females [15]. Oligodendrogliomas can arise anywhere within the central nervous system, but the majority of tumors are found within the frontal and temporal lobes of the cerebral hemispheres. Other cortical regions are less involved and oligodendrogliomas are rare within deep nuclei or spinal cord. Microscopically, these tumors are composed of a relatively monomorphous population of cells with round-to-oval nuclei with delicate chromatin pattern, and surrounded by perinuclear ‘halos’ (cytoplasmic clearing), an artifact seen in formalin-fixed and paraffin-embedded sections. The vasculature is typically thin-walled and described by some authors as ‘chicken-wire’ vasculature (Fig. 2.2e).
The WHO classification assigns two grades to oligodendrogliomas: well-differentiated relatively slow-growing tumors correspond to WHO grade II, whereas oligodendrogliomas with anaplastic features are assigned WHO grade III. Anaplastic oligodendrogliomas are characterized by an increase in cellularity and nuclear atypia, increased cellular pleomorphism, in addition to increased mitotic activity, endothelial proliferation, and necrosis. In contrast to other gliomas, such as astrocytomas and ependymomas, oligodendrogliomas show a more slowly progressive clinical course [3].
Diffuse Oligoastrocytomas
Oligoastrocytomas are defined as diffusely infiltrative glial neoplasms consisting of a mixture of two distinct cell types, which morphologically resemble the tumor cells of diffuse astrocytomas as well as oligodendrogliomas. These two components coexist either side by side or in a diffusely intermingled fashion. Definitive criteria for identification and classification of these lesions, however, remain somewhat controversial. Oligoastrocytomas are graded as WHO grade II lesions, and the acquisition of anaplastic features will increase the grade to WHO grade III.
Although oligoastrocytomas appear to have a mixed phenotypic appearance, they seem to demonstrate either an astrocytic or oligodendroglial genotype. This indicates that these tumors do not form a separate entity. The new WHO classification recommends molecular testing to assign these tumors a definitive lineage. Therefore, the new WHO classification discourages the diagnoses of oligoastrocytoma and anaplastic oligoastrocytoma. The term ‘oligoastrocytoma, NOS’ and ‘anaplastic oligoastrocytoma, NOS’ should be used in cases when gliomas are morphologically mixed or ambiguous and cannot be resolved using molecular testing [2, 18].
Molecular Profiles of Diffuse Astrocytic and Oligodendroglial Tumors
Isocitrate Dehydrogenase (IDH) Mutations
The hallmark genetic alterations in diffuse gliomas are somatic mutations in the gene encoding human cytosolic NADPH-dependent isocitrate dehydrogenase 1 (IDH1), a citric acid cycle component. Less frequently involved are mutations of IDH2. The IDH1 enzyme normally catalyzes the oxidative carboxylation of isocitrate to alpha-ketoglutarate (α-KG), resulting in the reduction of nicotinamide adenine dinucleotide phosphate (NADP) to NADPH. Numerous studies uncovered several mechanisms to explain the tumorigenic potential of IDH proteins. There is convincing evidence that mutant IDH, such as IDH1 (R132H), acquires neomorphic activity that converts alpha-ketoglutarate (α-KG) to 2-hydroxygluturate (2-HG) [19]. 2-HG in turn inhibits α-KG-dependent dioxygenases, including the members of the TET family of 5-methylcytosine hydroxylases and Jumonji-C domain-containing histone lysine demethylases. It has been shown that inhibition of these enzymes increases DNA and histone methylation, which eventually triggers the aberrant methylation of multiple cytosine–phosphate–guanine (CpG) dinucleotide-rich islands across the genome [20]. This ‘glioma CpG-island methylator phenotype (G-CIMP)’ is a characteristic profile seen in diffuse gliomas [21, 22] and likely contributes to the neoplastic transformation of neural stem or progenitor cells. Additional studies have shown that the production of 2-HG stimulates the activity of prolyl-hydroxylase domain isoform 3 (PHD3/EGLN) and prolyl 4-hydroxylases, which leads to reduced levels of hypoxia-inducible factor (HIF) and consequently to the enhanced proliferation of human astrocytes [23]. Increased oxidative stress due to decreased intracellular NADPH levels as a result of IDH mutations additionally promotes tumorigenesis [24].
It has been postulated that IDH mutations likely represent an initiating event, but that they are probably not sufficient to induce tumor growth on their own, instead they have to be accompanied by additional genetic mutations. Mutations involving tumor protein 53 (TP53) and ATRX genes play a role in diffuse and anaplastic astrocytomas. Co-deletions of 1p/19q and mutations involving the promoter region of telomerase reverse transcriptase (TERT) have been described for oligodendrogliomas [25].
These mutational profiles appear to be mutually exclusive and define tumor lineages: The combination of IDH1/p53/ATRX and IDH1/1p19q/TERT are mutational signatures for astrocytic tumors and oligodendrogliomas, respectively [26] (see Fig. 2.1). The new WHO classification requires the demonstration of both IDH1 mutation and 1p19q co-deletion for the diagnosis of oligodendroglioma and anaplastic oligodendroglioma. Similarly, the diagnosis of diffuse or anaplastic astrocytoma requires molecular testing for IDH mutations, with additional demonstration of ATRX mutation or loss of nuclear ATRX expression confirming an astrocytic lineage [27, 28].
Numerous studies established that IDH1 mutations are present at high frequency in secondary glioblastomas that originate from prior low-grade gliomas (~85%), which is contrasted by the fact that these mutations rarely occur in primary or de novo glioblastomas (<1%), which are found in the absence of low-grade precursor lesion. IDH1 mutations are further identified in the vast majority of diffuse low-grade (WHO grade II) and anaplastic (WHO grade III) astrocytomas (~70–80%), oligodendrogliomas (80%), anaplastic oligodendrogliomas (85%), and mixed oligoastrocytomas (100%). The IDH1 mutation frequency appears to be similar for WHO grade II and WHO grade III tumors [29]. Interestingly, the mutation rate in pilocytic astrocytomas (WHO grade I), ependymal tumors, or other less common glial tumors is extremely low or absent [13]. It was further demonstrated that IDH1 mutations do not exist in reactive conditions related to cerebral ischemia or infarctions, viral infections, or radiation change [30]. These findings are of particular diagnostic value as they enable the distinction of reactive gliosis from low-grade diffuse astrocytoma, a diagnostically challenging task, especially in the context of small biopsy samples.
It is of clinical importance that IDH 1 and IDH 2 mutations are found to be associated with a favorable prognosis and overall prolonged survival time independent of treatment. The survival of patients with the mutant form of IDH1 in astrocytomas or oligodendrogliomas (WHO grade II-III) and glioblastoma is longer than that of their IDH1 wild-type counterparts. Interestingly, patients with IDH1-mutated glioblastomas (WHO grade IV) show better survival than patients with wild-type anaplastic astrocytomas (WHO grade III). The IDH status, however, does not predict treatment-specific responses of patients with glioma [31].
In 2010, an antibody (Fig. 2.3a) was developed which is able to specifically recognize the mutant IDH1-R132H protein, which represents the majority (90%) of glioma-associated hotspot mutations [32, 33]. In the case of IDH1-R132H-negative immunostaining, testing for other IDH1 or IDH2 mutations is required for WHO grade II and III gliomas as well as glioblastomas from young patients and secondary glioblastomas [34]. This is usually accomplished by direct DNA sequencing or pyrosequencing using DNA extracted either from frozen tissue or more commonly formalin-fixed tissue [35, 36].


Fig. 2.3
Molecular biomarkers detected in FFPE (formalin-fixed paraffin-embedded) tissue a Immunohistochemistry for IDH1 shows mainly cytoplasmic, to a lesser extent nuclear, staining. This mutation-specific antibody against the most common IDH1 mutation, R132H, allows the identification of more than 90% of all IDH-mutant diffuse gliomas. b FISH (fluorescent in situ hybridization) for EGFR amplification is recognized by innumerable interphase FISH signals in red (probe set for gene region 7p11). The green signal is a SE7 gene region probe to facilitate chromosome identification. c FISH (fluorescent in situ hybridization) to demonstrate loss of the short arm of chromosome 1 (1p loss) as indicated by the presence of only one red signal (probe binds to gene region on 1p). The green signal serves as control and is a centromeric enumeration probe for chromosome 1 (CEP1). The presence of 2 green signals indicates both chromosomes (paternal and maternal) are present
Co-deletion of 1p/19q
The combined deletion of the short arm of chromosome 1 (1p) and the long arm of chromosome 19 (19q) together with IDH1 mutations defines oligodendrogliomas and anaplastic oligodendrogliomas [37]. Mechanistically, this co-deletion results from of an unbalanced centromeric translocation and leads to loss of entire chromosomal arms t(1;19) (q10;p10). The frequency of 1p/19q co-deletions has been estimated to be 80–90% in WHO grade II oligodendrogliomas and 50–70% in WHO grade III oligodendrogliomas. In spite of a strong association between 1p/19q loss and classic oligodendroglioma morphology, morphology alone cannot predict the 1p/19q status. Interestingly, the chromosomal regions of 1p and 19q have been mapped in great detail; however, no definitive candidate genes have been identified which could explain the tumorigenic effect. Although the genes on 1p/19q remain enigmatic, numerous correlations have been established demonstrating that many tumors with 1p/19q co-deletions also show IDH1/IDH2 mutations; however, 1p/19q loss appears to be absent in cases with tumor protein p53 (TP53) mutations or EGFR amplifications. Notably, the combined loss of 1p/19q is also found in mixed glial tumors (oligoastrocytomas), but extremely rare in non-glial malignancies.
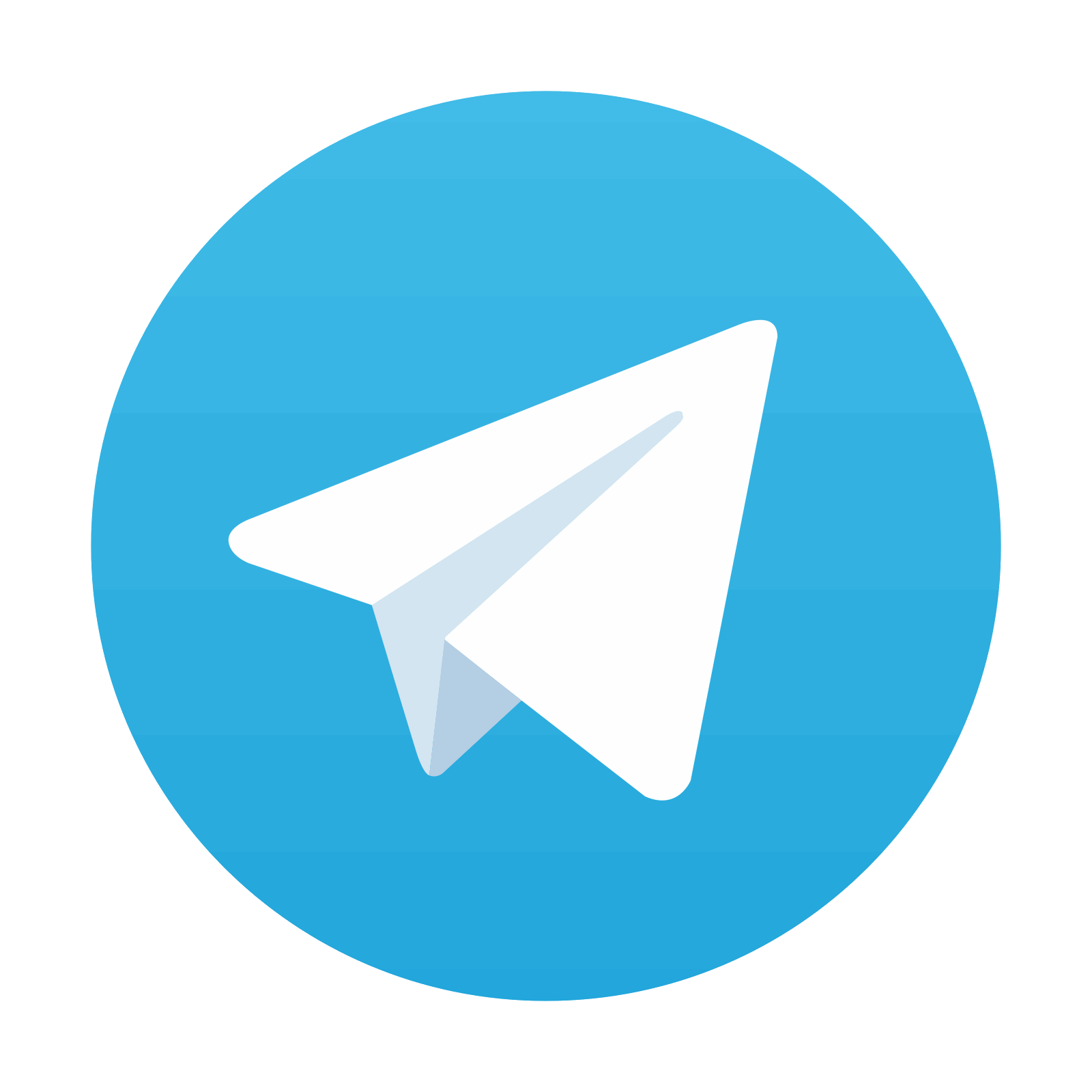
Stay updated, free articles. Join our Telegram channel

Full access? Get Clinical Tree
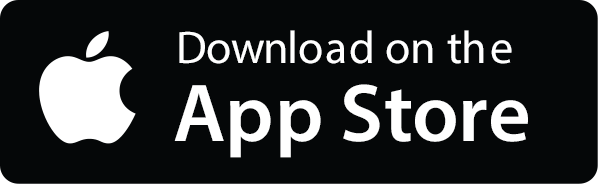
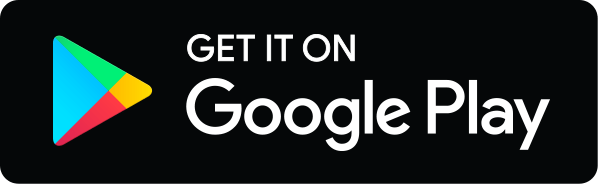