Multiple Subpial Transections and Other Interventions
Charles E. Polkey
Michael C. Smith
Introduction
Disconnection and ablation are long-standing techniques in the surgical treatment of epilepsy. They are based on the rationale that epilepsy afflicts the brain by setting up and using abnormal pathways within the brain, and that therefore, if such pathways are interrupted or destroyed, then the abnormal pathways and activity will cease to influence the brain and thereby reduce or remove the clinical manifestations of epilepsy. These ideas, however, were not based on pure speculation but initially on observations from electroencephalographic (EEG) recordings in experimental models. The development of epilepsy surgery both in North America and in Europe was much accelerated after the Second World War, and in particular the growth of stereotactic techniques applied to the clinical problems resulted in the use of stereotactic lesions in various cerebral structures to try to control epilepsy. A more detailed account follows later, but there were two main groups of targets. One group was the thalamic nuclei because of the perceived relationship between the thalamus and cortex; the other group was the temporal lobe structures, especially the hippocampus and amygdala, when it was recognized that they were part of the limbic circuit and concerned with temporal lobe epilepsy. There had been a search by the early workers for models in EEG work in animals, which would suggest that interventions that changed the EEG features would control clinical seizures. The work of Erickson and others demonstrating that the corpus callosum played a major part in the generalization of seizures from an epileptogenic focus was part of the rationale for the next major procedure, callosotomy.7 The present status of callosotomy is dealt with elsewhere in this volume. There were other operations of historical interest involving disconnection, as, for example, the temporal lobotomy described by Turner.49
By and large the field remained with callosal section and its variations until the description of multiple subpial transection by Morrell and Hanbery in 1969.23 This was based on extensive experimental work over many years on secondary epileptogenesis. A detail of this work was the demonstration that epileptic discharges travel tangentially in the cortex, and that a minimal population of neurones was necessary to maintain the momentum of an epileptic discharge. As a result, Morrell and Hanbery hypothesized that if the connections within and from epileptic cortex were divided horizontally, then the propagation of the epileptic discharge would be prevented and the clinical manifestations of seizures abolished. As a result, and based on the animal work, a surgical intervention was devised using special instruments to divide the short tangential fibers within the cortex.
The final stage in the development of disconnection and lesioning takes a physiologic twist: The discovery by Benabid et al. that high-frequency stimulation is effectively lesioning the adjacent tissue, with the advantage that it can be reversed.1
Multiple Subpial Transection
Rationale
Morrell himself pointed out that multiple subpial transection (MST) was a technique that attacked the epileptogenic lesion at its source by compromising the capacity of the cortex to develop the synchrony necessary for epileptogenesis. He advanced three types of experimental evidence to support this idea. The first was the idea that a minimal volume of transversely arranged contiguous tissue was necessary for the initiation and propagation of an epileptic discharge. The second was the observation from many different sources that the vertically orientated column is the key to cortical organization and that a consequence of this is that normal cortical function is based on these radially orientated columns and their afferent and efferent connections, largely in the same plane. This was broadly confirmed by some work carried out in Sperry’s laboratory in the 1950s, which showed that if the cortex was interrupted vertically by section and the insertion of mica plates, the normal function of that cortex was retained. Although not neglecting the subtle contributions of these tangential connections to normal cortical function, Morrell realized that the majority of cortical function would be available if the tangential fibers were interrupted. It was clear that the vertical cut had to involve the whole depth of the cortical ribbon, and from laboratory experiments he concluded that the distance between the cuts should be a maximum of 5 mm. The area of cortex to be transected (i.e., both the location and the actual area) was determined by defining the epileptogenic zone. This was performed mainly as a neurophysiologic exercise. As a check on the efficacy of the procedure, elimination of the abnormal discharges, which was not always possible, was taken as the end-point of the procedure.
Indications for Multiple Subpial Transection
Although MST can be used alone or in combination with cortical resection, the selection criteria remain the same. The selection criteria, based on the rationale for MST, are an identified area of epileptogenic cortex whose resection is precluded by potential loss of function. This translates into a number of clinical scenarios, some of which are listed in Table 1.
Table 1 Indications for Multiple Subpial Transaction | ||||||
---|---|---|---|---|---|---|
|
Technique
A clear strategy for the use of MST is needed prior to surgery. In addition to the usual clinical details and investigations involved in a presurgical assessment program, it is necessary to identify the epileptogenic zone. This will frequently depend on the use of intracranial electrodes, often grid electrodes, since the accurate identification of epileptogenic cortex will be required to determine the extent and location of the craniotomy. In operations for Landau-Kleffner syndrome, it is almost always necessary to open the sylvian fissure. Although the record of MST suggests that functional complications such as hemiparesis and dysphasia are low, where circumstances are appropriate it may be prudent to perform the procedure under local anaesthesia. It is clearly necessary to use electrocorticography in these cases in order to determine the end-point of the procedure.
Technical aspects of the procedure are fairly straightforward. A set of specially designed instruments is used (Fig. 1). Each consists of a heavy steel wire flattened at one end to a thickness of 0.3 mm and turned up at an angle of 105 degrees with respect to the rest of the wire. The upturned portion is exactly 4 mm long (the thickness of most areas of cortical gray matter in humans). The other end of the wire is fused to a handle that is flattened at the sides so as to be exactly aligned with the flattened surface of the upturned end of the wire. The latter feature is important because the instrument must be maintained in a strictly vertical orientation with respect to the gyral surface (even when the tip is not visible to the surgeon) to avoid damage to the vertically arranged neural elements. The “springiness” of the wire is also critical, as the tip must be drawn along the undersurface of the pia, slightly elevating it but not producing a pial tear.
The gyri containing epileptogenic activity are delineated by the presence of epileptiform potentials on intraoperative electrocorticography (ECoG). A small hole is made in the pia, using the tip of a Beevor knife, or with a No. 20 needle, at a site situated as close to the lateral margin of the sulcus as it is possible to reach, access often being limited by the presence of large blood vessels. The instrument is introduced through this pial opening and then swept forward, dipping in an arc-like fashion underneath the gyrus (Fig. 2). The thin but blunt blade is maintained in a strictly vertical orientation to avoid undercutting the cortex. The tip is maneuvered so that it impinges, as low as possible, on the subpial surface of the vertical portion of the gyrus opposite the point of insertion. It is then drawn up this surface until it reaches the visible part of the gyrus opposite the point of insertion. From here, the tip is visible beneath the pia, which it just elevates but does not penetrate. The blade is then gently drawn straight back across the gyrus in the same plane as its forward movement, with the tip always kept visible through the pia until the gyrus is transected. Care is taken not to snag with the blade of the hook any cortical vessels, especially from the opposite sulcus, and this is largely a matter of feeling the movement of the tip of the blade. Finally, the hook is inserted vertically downward at the point of insertion to approximately 4 mm, with the tip against the vertical wall of the sulcus and withdrawn to the surface. The next transection is made parallel to the first and 5 mm away (Fig. 2). A small piece of card cut to an appropriate size and held between the jaws of a hemostat can be used to judge the position of the next transection. Transections are repeated as often as necessary to include the entire area of electrical abnormality, and they may encompass several gyri. The capillary bleeding associated with each traverse of the instrument results in a fine red line. The bleeding does not seem to cause damage, and the line is a useful landmark against which to gauge the location of the next transection. This is continued over the selected area until the abnormal discharges have been minimized on ECoG; it is not always possible to abolish them completely, although this has been achieved in the case illustrated in FIGURE 3. The operative appearance of the transected cortex is shown in FIGURE 4, and in FIGURE 5 there is a photomicrograph of transection from a patient who subsequently underwent hemispherectomy.
There are technical limitations to the use of MST. Although the handles of the complete set of six instruments are designed to get into awkward areas, a minimal space is required in which to record and work. Furthermore, retraction to gain access may itself risk complications, and finally the distribution of venous drainage, especially in the posterior part of the hemisphere, may limit retraction. The buried part of the insular cortex can be exposed with careful microsurgical technique, and this is mandatory when using the technique for the treatment of Landau-Kleffner syndrome. Access to the orbital part of the frontal cortex is likely to be difficult, as is the primary visual area on the medial surface of the occipital lobe. However, in many of these areas access depends on the individual arrangements of vessels in any particular patient. As may be surmised, although the trauma is minimal, the technique is traumatic and therefore, after transecting large areas, some swelling might be expected. In the absence of clinically significant problems, the use of postoperative steroids is not mandatory.
![]() FIGURE 4. Appearance of the cortex in a patient with Rasmussen’s disease who has undergone a frontal resection and multiple subpial transection of the adjacent cortex. |
Outcome from Multiple Subpial Transection
A number of considerations apply to the assessment of the outcome from MST. These include whether the technique is used alone or in combination with a resection, the effect on seizure control using accepted outcome scales, and the frequency of complications, especially in relation to the normal function of the treated cortex. With the exception of some reports from China and the meta-analysis of Spencer et al.,47 the number of patients described in series in the literature is <100. The methodology of this meta-analysis varies in two other respects from the other papers. The patients are grouped into those
with >95% improvement in seizure control, so that it is not possible to discover how many patients were seizure free. It also deals with the response of individual seizure types to the intervention. In this respect the authors make two important observations. The first is that the procedure is more effective in partial seizures than generalized seizures and that this is the expected result from the physiologic basis of MST. They also noted that in their material the superiority of resection combined with MST over MST alone applied only to generalized seizures. Finally, they also reported that partial seizures sometimes increased in frequency after MST. The literature includes reports of patients varying in numbers from 10 to 200, and is invariably divided into those patients in whom the technique has been combined with resection and those in whom it was used alone. In general, and possibly at variance with the meta-analysis, complete seizure freedom is only seen where MST is combined with resection. In relevant series, seizure-free rates between 31% and 56% are reported.2,5,13 Mulligan et al. reported that none of their 12 patients became seizure free, and although it was not statistically significant, it appeared that resection with MST was more effective.25
with >95% improvement in seizure control, so that it is not possible to discover how many patients were seizure free. It also deals with the response of individual seizure types to the intervention. In this respect the authors make two important observations. The first is that the procedure is more effective in partial seizures than generalized seizures and that this is the expected result from the physiologic basis of MST. They also noted that in their material the superiority of resection combined with MST over MST alone applied only to generalized seizures. Finally, they also reported that partial seizures sometimes increased in frequency after MST. The literature includes reports of patients varying in numbers from 10 to 200, and is invariably divided into those patients in whom the technique has been combined with resection and those in whom it was used alone. In general, and possibly at variance with the meta-analysis, complete seizure freedom is only seen where MST is combined with resection. In relevant series, seizure-free rates between 31% and 56% are reported.2,5,13 Mulligan et al. reported that none of their 12 patients became seizure free, and although it was not statistically significant, it appeared that resection with MST was more effective.25
Similar outcomes are described in children. In a report of 158 pediatric cases, Shimuzu and Maehara used MST in 25 (15.8%) and reported an Engel class I or II outcome in ten of these cases.45 Hader et al., in a series of children with focal cortical dysplasia (FCD), noted that when combined with incomplete resection MST did not improve the control of seizures, whereas complete resection of the lesion outside of an eloquent area gave the best results, with 87% of patients in Engel class I or II compared with 50% of patients who were treated with incomplete resection and MST.11 Otsubo et al. reported the use of MST in seven children with malignant rolandic-sylvian epilepsy. In six children interictal scalp EEG spikes were seen over the frontocentrotemporal regions bilaterally and unilaterally in one. Magnetoencephalography (MEG) showed bilateral spike sources in the perisylvian region in two patients and in the perirolandic fissure in five, two of which were bilateral. Three patients required bilateral subdural strips to lateralize seizures before electrocorticography. ECoG showed an ictal onset zone around the rolandic region in four cases and the rolandic-sylvian regions in three cases. After cortical excision and multiple subpial transection, three were seizure free and four had seizures rarely with a mean follow-up of 30 months. Histology showed definite evidence of neuronal migration disorders in three cases and gliosis in two.29
There are a number of reports of MST being used alone. The largest series of 20 patients was described by Schramm et al. Although a good outcome, judged either by Engel’s scale or by an alternative outcome scale, was seen in 45% and 50% of patients, respectively, only two patients (10%) were seizure free. A better outcome was seen when there was no magnetic resonance imaging (MRI) lesion and a worse one when the area of MST was greater.42 Wyler’s group described six patients in whom sensory motor cortex was transected. In four of these patients the MRI was normal. Where samples were taken the cortex showed gliosis. One patient was rendered seizure free and two of the remaining patients had a >90% decrease in their seizures.50 Rougier et al. also reported seven patients with pure MST, and three patients had a >75% decrease in their seizure frequency with follow-up periods between 1 and 4 years.41 Shimizu et al. described the use of MST in the exposed hippocampus, using abolition of seizure discharges as the end-point. They did not report the result with reference to seizure control but noted that postoperatively, verbal memory was preserved. In the absence of other details it is difficult to evaluate this report.44 The two reports from Chinese groups do not distinguish between various combinations of treatment and therefore their seizure-free rates of 64% and 47.5% are difficult to interpret.18,19
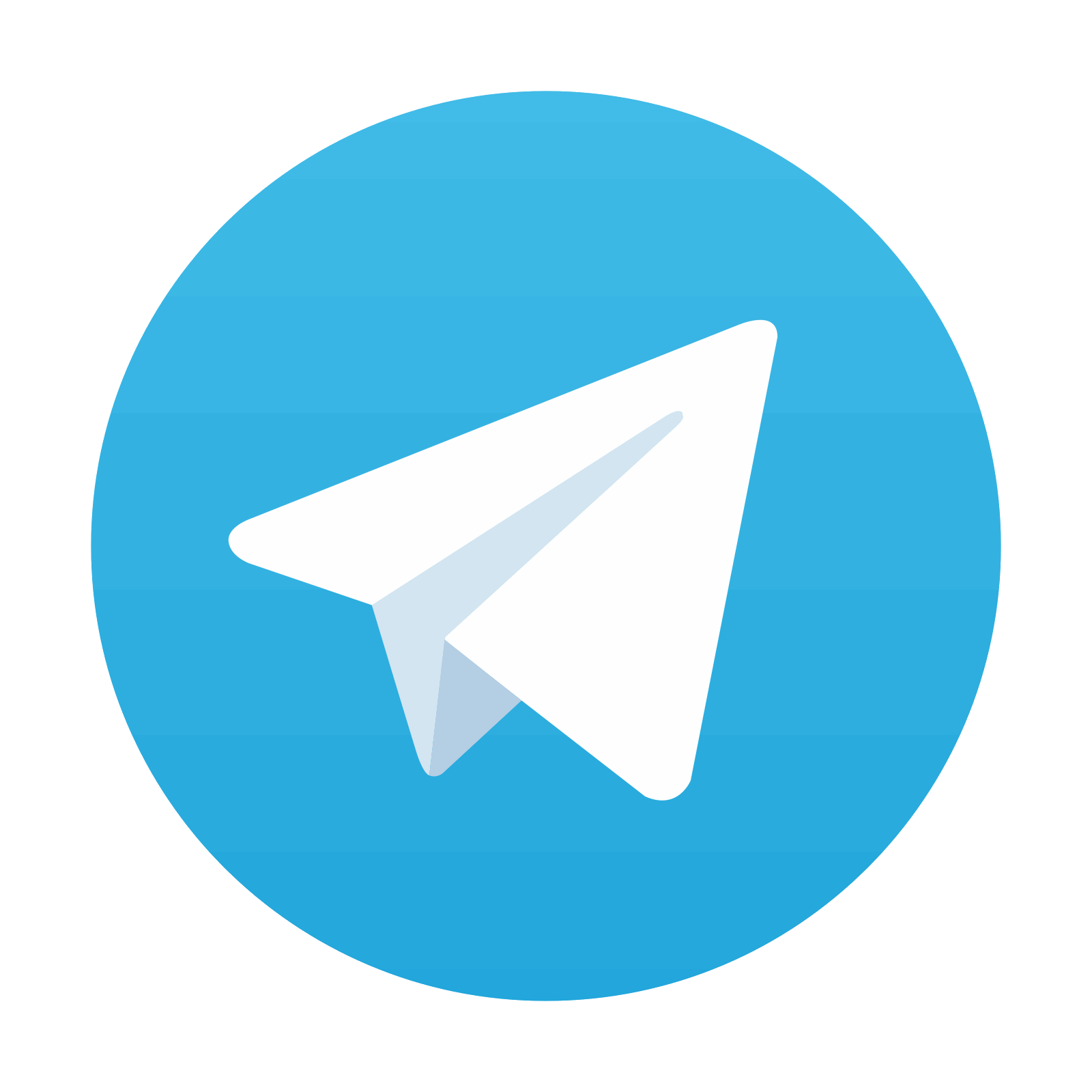
Stay updated, free articles. Join our Telegram channel

Full access? Get Clinical Tree
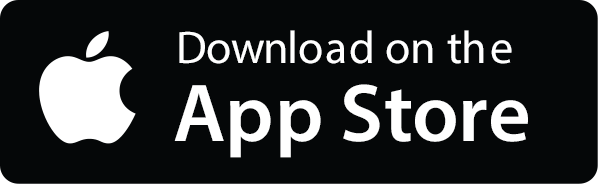
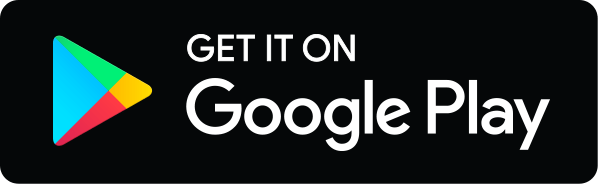